1 The Human Spinal Disc: Relevant Anatomy and Physiology
Julien Tremblay Gravel, Fahad H. Abduljabbar, Jean Quellet, and Lisbet Haglund
Abstract
The intervertebral disc (IVD) is a well-engineered avascular fibrocartilaginous organ designed to unite two adjacent vertebral bodies. Its anatomical and physiological properties provide constrained motion and force dissipation while maintaining the mechanical stability of the spine. The IVD is divided into two main sections: the annulus fibrosus (AF) and the nucleus pulposus (NP). The NP’s structure is of gelatinous consistency and has a high concentration of aggrecan and water that enable it to resist compression. As the spine is axially loaded, the forces are dissipated via the NP and the lamina of the AF.
Keywords: anatomy, biomechanics, intervertebral disc, spine
1.1 The Vertebral Column
The vertebral column is part of the axial skeleton; it is composed of 33 vertebral bodies that connect the skull base to the pelvis. It is divided into five regions: cervical (7 vertebrae), thoracic (12 vertebrae), lumbar (5 vertebrae), sacral (5 vertebrae), and coccygeal (4 vertebrae). The vertebral bodies are named in the cervical region C1–C7, in the thoracic region T1–T12, and in the lumbar region L1–L5. In the coronal plane the spine is straight, yet in the sagittal plane the spine has primary and secondary curves (▶ Fig. 1.1). The primary curvatures consist of the thoracic and sacral kyphosis. As we learn to sit and stand, the secondary curves take shape, giving rise to the cervical and lumbar lordosis. These curves are important for absorbing forces, maintaining balance, and allowing a range of motion throughout the vertebral column. Motion within the vertebral column varies between each spinal segment. The greatest freedom of motion is found in the cervical and lumbar segments and the most restrained motion is found in the thoracic and sacral segments as they are constrained by the ribs and pelvis. Each vertebra is composed of the vertebral body anteriorly and vertebral arch posteriorly. Each vertebral segment has a spinal canal and two intervertebral foramina, formed by the bony structures of the posterior arch. These structures provide a protected passage for the spinal cord and nerve roots, respectively. The vertebrae also serve as anchor points for the rib cage posteriorly, which helps protect the thoracic cavity organs. The vertebral arches have, posteriorly, two articulating synovial diarthrodial joints called facet joints. The facet joints’ surfaces are covered with articular cartilage and are enclosed by a synovial capsule. The facets prevent two adjacent vertebrae from translating during spinal motion, avoiding damage to the nerve roots and spinal cord. IVDs separate the upper 24 vertebral bodies, whereas the lower 9 are fused in adults. The IVDs and the facet joints provide the capacity for flexion and extension and to a lesser extent, rotation and lateral bending. Moreover, these facets share the load transmitted through the spine with the IVDs. The IVD is a fibrocartilaginous organ uniting two adjacent vertebral bodies, contributing to the spine’s height and function (▶ Fig. 1.2). The discs are named according to the upper and lower vertebrae they join. For example, the disc situated between the thoracic vertebra T12 and the lumbar vertebra L1 is called T12–L1. The discs, in aggregate, make up approximately one fourth of the height of the spinal column excluding the sacrum and coccyx.1 The human spinal disc is essential for maintaining posture and for protecting delicate neural tissue and rigid structures of the vertebrae and skull during locomotion.
Fig. 1.1 Schematic representation and anatomical regions of the human spine.
1.2 Development of the Intervertebral Disc
The internal structure of the disc has distinct anatomical regions of different developmental origin. These structures are traditionally separated into the central, gelatinous NP, the outer, fibrous AF, and the cartilaginous end plates.
Structures of the spinal column originate from the notochord and from the sclerotome of the mesodermal somites. There are four developmental stages to the formation of the vertebrae and discs. First, the notochord is formed from the mesoderm during gastrulation. It is positioned in the dorsal region of the embryo, along the anteroposterior axis. The notochord consists of a flexible core of glycoprotein with high osmotic potential surrounded by a sheath of fibrous connective tissue.2 During the second stage, at the 4th week of development, cells of the sclerotome migrate around the notochord forming the vertebrae, cartilaginous end plate, AF, and ribs. In the ventromedial region, the notochord is segmented. Some sections are remodeled to make way for the forming vertebral bodies, whereas others expand to form the NP, the gelatinous portion of the IVD.3 The annulus is formed by a condensation of sclerotomal cells surrounding the remaining sections of notochord. The condensation of these cells makes way for the expansion of the notochordal tissue, forming the NP.4 During the third stage, at 6 weeks, the vertebral parts of the spine undergo chondrogenesis and become cartilaginous (▶ Fig. 1.3). The fourth and final stage is ossification, which begins during the 8th week of the embryonic period and is completed around 25 years of age.
During fetal/early natal life, blood vessels penetrate the disc to the inner annulus. By the juvenile stage, the blood vessels resorb to the outer annulus and the cartilaginous end plates.5 In the nondegenerate adult disc, blood vessels are only seen in the connective tissue surrounding the AF and budding in the cartilaginous end plates. This lack of vascularity limits the flow of nutrients reaching the central region of the disc. Nerves follow a similar pattern, penetrating only the outer AF in nondegenerate adult discs (▶ Fig. 1.4, ▶ Fig. 1.5).
1.3 Cells in the intervertebral Disc
The overall cell density of the disc is fairly high in the fetal stage but decreases significantly with age, especially in the regions furthest from the periphery.
Cells of the NP are notochordal in origin.6,7 Notochordal cells are large (> 15 μm) and contain large vacuoles,8 whereas mature NP cells are relatively small (10 um diameter) and display a rounded chondrocyte-like morphology. Multiple studies have demonstrated that cells of the mature human NP express distinct notochordal markers, such as brachyury, further establishing the notochord as the developmental origin of NP cells.8,9 The vacuoles of notochord cells carry a multitude of anabolic factors suggested to induce matrix synthesis in neighboring cells. Notochordal cells disappear with age and are no longer visible by the age of 4 in humans.2 Loss or terminal differentiation of these cells may therefore contribute to age-related tissue deterioration. NP cells are situated in lacunae and do not contain vacuoles.3 They are sparsely and randomly distributed within the tissue, with a cell density of about 4,000 cells per mm3 in the adult.10
Annulus cells originate from the sclerotome, and are elongated and spindle shaped. They are arranged following the lamellae’s orientation. Their diameter varies between 15 and 30 um.11 The AF is more densely cellularized, with about 9,000 cells per mm3 in the adult human.10
The cells in the end plate cartilage are chondrocytes of mesenchymal origin. Like in the deep layers of articular cartilage, the chondrocytes are situated in lacunae arranged in a columnar fashion. The end plate has the highest cell density of any disc tissue, with approximately 15,000 cells/mm3.10 Their average diameter is 20 μm12 (▶ Fig. 1.6).
NP, AF, and cartilage end plate cells are, in addition to matrix synthesis, responsible for maintenance and turnover of the extracellular matrix (ECM), a process that is in balance in the young and nondegenerate disc.
1.4 Intervertebral Disc Organization and Composition
The disc’s distinct anatomical regions have different mechanical and biological properties. The AF and NP regions are clearly distinguishable in fetal and juvenile discs but the clear demarcations diminish in the adult. The NP region expands in the adult disc into a transition region called the inner AF, and it can be difficult to establish where one region begins and the other ends (▶ Fig. 1.2). The tissue regions contain similar matrix elements, albeit in widely differing concentrations.13 The disc’s ECM is rich in collagen and proteoglycan. Collagen is a ubiquitous protein in mammalian connective tissue. Different types of collagen are present in varying amounts within the disc, but the most prominent by far are types I and II. Other collagens present within the mature, nondegenerate disc are types III, V, VI, IX, XI, XII, and XIV.14 Collagen provides attachment to disc cells and a three-dimensional (3D) mesh confining other matrix elements, such as proteoglycans. Proteoglycans exist in two forms within the disc, either bound to hyaluronic acid or unbound. The most abundant proteoglycan within the disc is aggrecan. In early development stages, most aggrecan within the disc is bound to hyaluronan, with a shift toward unbound forms in later stages of development. Other proteoglycans found in the disc’s ECM are versican and members of the small leucine rich protein (SLRP) family: chondroadherin, decorin, fibromodulin, and lumican.15 The SLRPs have many defined functions in the tissue and most of them carry glycosaminoglycan (GAG) chains. Chondroadherin is one of the few without GAG chains; it anchors the cells to the ECM via integrin and syndecan receptors.16,17,18 Decorin, fibromodulin, and lumican carry GAG chains. Decorin cross-links collagen fibers, whereas fibromodulin and lumican have highly negative sulfated tyrosine domains that bind cytokines and matrix metalloproteinases.18,19,20 Proteoglycans are made up of a core protein to which one or more GAG chains of highly sulphated repeating disaccharide units are covalently attached. Most proteoglycans have 1 or 2 GAG chains, whereas aggrecan has up to 150.
1.4.1 Nucleus Pulposus
The NP’s structure is gelatinous and has high aggrecan content. This molecule’s many GAG chains contribute to water retention and provide swelling pressure through their fixed negative charges. Aggrecan is the greatest contributor to NP function by enabling it to resist compression. Aggrecan concentration is highest in the central portion of the NP and declines throughout the AF. The other matrix molecule primarily responsible for the mechanical function of the NP is collagen type II. Collagen provides a scaffold entrapping aggrecan and other molecules and provides tensile properties to the tissue. Collagen and aggrecan make up 20% and 50% of the NP’s dry weight, respectively.21 The same molecules are responsible for the mechanical properties of cartilage. However, the ratio of aggrecan to collagen is 2:1 in cartilage, whereas it is 27:1 within the NP.22 Although aggrecan represents 50% of the dry weight, 70 to 90% of the NP’s wet volume is occupied by water bound to aggrecan.23 The high proteoglycan content with its negative charge is also thought to be a major factor preventing nerve ingrowth into the largely aneural and avascular mature, nondegenerate IVD.24,25 Peripherally, the NP is encircled by the AF.
1.4.2 Annulus Fibrosus
The function of the AF is to restrict lateral motion, as well as to prevent extrusion, of the nuclear material. To accomplish this, the collagen fibers of the mature annulus are arranged in up to 25 concentric lamellae wrapped around the NP. The lamellae are parallel to one another traversing between adjacent vertebrae at an angle of 60 degrees to the axis of the spine (▶ Fig. 1.2). The collagen fibers within a single annular lamella are organized in a parallel fashion, whereas the fibers in adjacent layers differ by 30 degrees.26 The concentration of collagen type I is highest in the annulus and decreases radially toward the NP. Collagen type II follows an inverse pattern with the highest concentration in the NP.21
Proteoglycans, such as aggrecan, are present throughout the AF but at a concentration much lower than in the NP. Collagen makes up 50 to 70% of annular tissue dry weight and proteoglycans only 10 to 20% of dry weight.27 Elastin fibers, arranged in a network between the lamellae, contribute to the structure and mechanical functions of the AF.28
1.4.3 Vertebral End Plate
The vertebral end plate has a dual function. It anchors the disc to the vertebrae and provides the main avenue for nutrient and waste exchange for the disc. Hence, it must be both resilient and porous. To achieve this, it is made up of two layers, the cartilage end plate consisting of hyaline cartilage and the bony end plate consisting of cortical bone. The main components of the cartilage end plate are water, type II collagen, and proteoglycans. Water makes up to 80% of tissue weight after birth, but lowers to below 70% after 15 years of age.21 The ratio between proteoglycans and collagens within the cartilaginous end plate is 2:1, which is similar to that of articular cartilage.22 The collagen fibers of the annulus’ lamellae clearly extend into the cartilage end plate, whereas integration is comparatively less organized in the NP region.29 Along the outermost region of the disc, the end plate is absent and the AF integrates directly with the vertebral bodies.30 The collagen fibers of the bony end plate are not connected to those of the cartilaginous layer.31
The thickness of the cartilage varies across the end plate and at various spinal levels, from 0.1 to 2.0 mm. The thickness of the cartilage end plate is higher in upper spinal levels than in lower spinal levels. Cartilage thickness is higher in the periphery of the disc and is thinner in the central region.32 The thickness of the bony layer, like that of the cartilaginous layer, varies between spinal levels and within each end plate. It is thicker at higher spinal levels and in the outer ring of each end plate.33 The bony end plate on the cranial surface of each spinal segment is also thicker and has higher bone mineral density overall than the one covering the caudal surface.34
With age, there is a decrease in aggrecan content and an increase in collagen and calcification of the cartilage end plate.35 This participates to the end plate’sgradualloss of permeability with age, reducing nutrient exchange.36 Microfractures within the end plate also become more common, leading to bulges of nuclear tissue into the end plate later in life. This decompresses the nuclear tissue, transferring the biomechanical load to the annulus.37 Nuclear extrusions into the end plate will progressively become calcified, forming Schmorl’s nodes.
1.5 Vascularization and Innervation of the Intervertebral Disc
Vascularization of the end plate is supplied by the basivertebral vessel bundles, which enter the vertebral body through the posterior basivertebral foramen and capillary branches from the segmental artery arising from the anterior spinal artery.38 Early in postnatal life, the vascular density of the end plate decreases, reducing nutrient supply to the disc. The nondegenerate adult disc is largely avascular and the capillaries form an intricate “mesh” throughout the porous bony end plate and bud in the superficial cartilage layer, providing nutrient delivery and waste exchange with the disc tissue (▶ Fig. 1.4). The density of this network is greatest in the region adjacent to the NP.39
Following a similar pattern to vascularization, the nondegenerate adult human disc is largely aneural. The nerve fibers surrounding the discs are branches of the sinuvertebral nerve, or derived from the ventral rami or gray rami communicantes.38 A meningeal branch of the spinal nerve, known as the recurrent sinuvertebral nerve, nerve of Luschka, or ramus meningeus, originates near the disc space. This nerve exits from the dorsal root ganglion and enters the foramen, where it then divides into a superior and an inferior branch to supply two IVD spaces. The posterolateral aspects of the discs receive branches from adjacent ventral primary rami and from the gray rami communicantes near their junction with the ventral primary rami. The lateral aspects of the discs receive other branches from the rami communicantes.40 Nerve endings rarely penetrate beyond the outer layer of the AF in a nondegenerate adult disc (▶ Fig. 1.5). However, nerve fibers deep inside the disc have been demonstrated in painful degenerate samples, often accompanying blood vessels.13 Innervation is an important distinction between the diseased and aged disc. More information on the physiology of degenerative discs is available in Chapter 2.
1.6 Disc Mechanical Properties
The unique structure of the IVD facilitates the capacity to absorb shock and transmit high forces from different vectors around the spine. Yang and King reported that between 75 and 97% of the compressive load applied to the lumbar spine is carried by the IVDs.41 The tensile and elastic properties of different components of the disc allow a state of equilibrium between stability and flexibility. The fluid in the NP is distorted by compression forces from the vertebral bodies and redistributes them radially to the AF. In turn, the AF is distorted by the pressure imparted by the NP which allows the disc to be compressed, and the resilience of the AF allows it to recover its shape after the pressure has been removed (▶ Fig. 1.7).
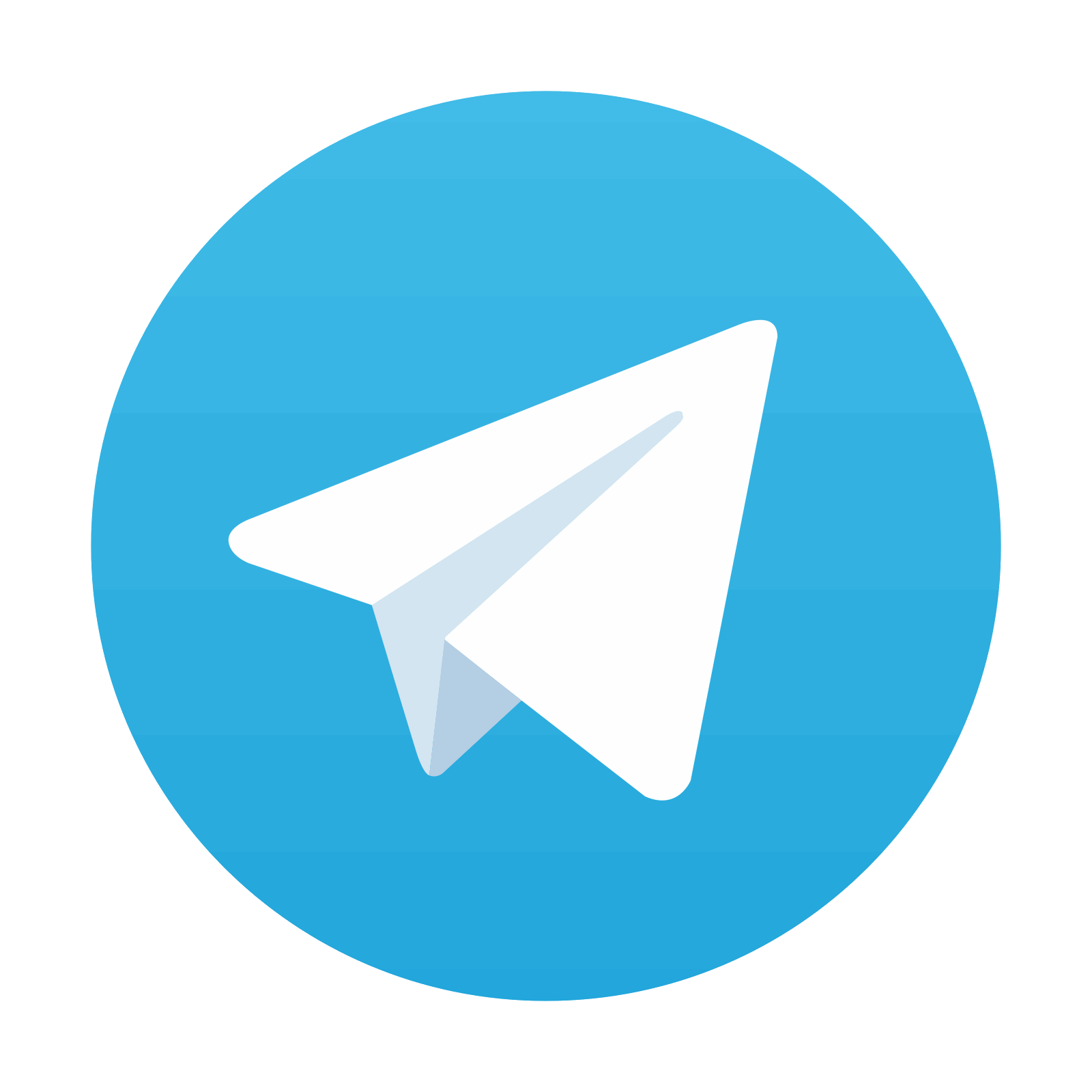
Stay updated, free articles. Join our Telegram channel

Full access? Get Clinical Tree
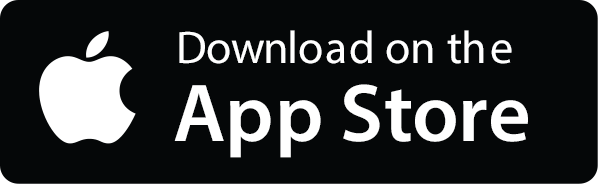
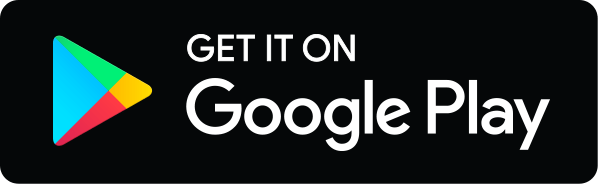