Fig. 3.1
Acute pain does not influence risk taking behavior. Here depicted: mean values +/− standard error of the mean for N = 24 healthy participants (third experiment of a series of three similar experiments) for two conditions (painful versus control) and three consecutive sessions of approximately 7 min each. The y-axis shows the average “number of pumps” (a measure of risk taking with higher number representing higher risk taking) for each session and condition. The graph is representative for all three experiments we conducted: acute pain has no effect on risk taking in an experimental setting
Long-Term Pain
In contrast to short-term stress, long-term exposure, which encompasses persistent pain, is associated with stress-induced hyperalgesia rather than stress-induced analgesia [94]. Dopamine release in the nucleus accumbens has been shown to be reduced in chronically stressed rats [95, 96]. Similarly, dopamine release in response to experimental pain has been found to be reduced in patients with chronic widespread pain [81]. As discussed above, the role of dopamine for pain processing is currently not clear, but if dopamine had indeed pain-reducing properties as suggested by animal studies, stress-induced attenuation of phasic dopamine release might lead to hyperalgesia. Since tonic and phasic dopamine activity are inversely related [56, 97, 98] decreased phasic dopamine release in chronic pain/stress might be related to increased tonic dopamine levels [74]. To date, however, no direct evidence of increased tonic dopamine levels induced by long-term stress or pain exists to our knowledge. In fact, human dopamine receptor positron emission tomography (PET) studies show increased striatal binding potential in patients at rest compared to healthy controls [99, 100], suggesting either lower endogenous dopamine levels or increased receptor density/affinity [101]. Consequently, it is currently only safe to conclude that dopaminergic neurotransmission is altered in chronic pain. Considering the prominent role dopamine plays in motivation, altered dopaminergic neurotransmission in pain patients may change their motivational drive to obtain reward. Some preliminary evidence exists for alterations of motivational drive in chronic pain patients [102, 103]; however, we think that the direction of change can not necessarily be inferred from these studies that found reduced motivation, primarily because they did not control for depression.
In addition to its impact on the dopaminergic system, repeated exposure to stress has been shown to change the opioidergic system. In rats exposed to long-term stress (daily 1 h movement restraint on 5 days per week for 40 days in total), analgesic effects of morphine were significantly decreased compared to a nonstressed control group [94]. Results from animal stress studies are presumably important for clinical long-term pain as chronic pain can be understood as an unavoidable long-term stressor. In fact, human patient studies using opioid receptor PET have revealed decreased resting binding potentials in chronic pain patients suffering from neuropathic pain [104] and fibromyalgia [105]. Reduced resting binding potentials can result from increased endogenous opioid levels as well as decreased receptor density/affinity, as discussed above. Theoretically, both of these possible mechanisms might contribute to the reduced binding potential in pain patients: opioid systems might be chronically activated in patients in an attempt to reduce pain. To attenuate cellular responses to increased levels of agonists receptor internalization can occur, decreasing the density of available receptors in order to protect the cell [101]. If internalization of opioid receptors occurred also in neuronal assemblies concerned with reward processing, opioid release in response to rewarding stimuli might consequently be altered in chronic pain patients. In support of this hypotheses, anhedonia [106] has been observed in chronic pain patients.
There are further studies indicating that reward processing is impaired in chronic pain albeit they do not always allow pinpointing the specific aspect of reward that is altered. For instance, chronic pain patients suffering from fibromyalgia or complex regional pain syndrome have been shown to be impaired in improving their performance on reward-dependent operant learning tasks [107, 108]. These studies suggest that pain patients are malfunctioning in their reward processing on a cognitive [109] as well as on a perceptual level [107], which is possibly related to reduced hedonic responses, reduced motivation, and/or altered reward sensitivity.
In many instances, the existing literature on altered reward processing in pain patients does not allow excluding potential influences of medication or pain at the time of testing. Nevertheless, support for the influence of long-term pain on reward processing is provided by animal data. It has been shown that rats with neuropathic pain symptoms require higher doses of rewarding morphine than sham operated animals to learn a place-preference [110]. Similar to the human studies, this could be caused by decreased “liking” of the reward, decreased motivational drive or decreased reward sensitivity. These results are complemented by a study showing that rats with persistent inflammatory pain developed a preference for a higher risk option over a lower risk option, indicating potentially higher tolerance of not receiving reward, in the presence of a potentially decreased motivation to work for reward [111]. These results are not fully in accordance with the few human studies indicating reduced motivation in chronic pain patients. However, as pointed out above, it would seem important to control for depression and other comorbidities in chronic pain patients that might lead to reduced motivation. Animal models might in many instances not sufficiently model comorbidities that often accompany chronic pain in humans.
What Is the Significance of Pain-Induced Alterations in Reward-Processing Systems for Addiction?
Exogenously administered opioids are the most powerful analgesics available. Opioids are effective with short-term use [112–116] as well as with long-term use over months [117]. Nevertheless, opioid treatment has been discussed critically with respect to its potential to inflict tolerance, dependence, and/or addiction [118]. In the following, we speculate how pain-induced changes in reward processing as well as in dopaminergic and opioidergic neurotransmission might contribute to addictive behaviors in susceptible individuals.
As outlined above, chronic pain constitutes a constant stressor. Enhanced stress levels are well-known risk factors for addicted behavior and relapse (for review, e.g., [119]) suggesting that pain patients might be vulnerable to become addicted. Some studies suggest that pain increases motivational drive while the pleasure associated with reward is not changed or even decreased, although it is currently not known whether the effects of pain extend beyond the effects of stress. The constellation of increased motivation without an increase in pleasure may lead to a vicious circle of increased desire for and seeking of reward. In the context of opioid therapy for long-term pain, decreased pleasure associated with reward translates into less pain relief, so that gradually increasing drug doses are required. Moreover, prolonged use of opioidergic drugs can lead to negative emotional states, caused by withdrawal symptoms or by a lack of expected drug effect due to tolerance. Increased negative emotions, decreased positive emotions and the constant exposure to stress lead to “hedonic worsening” over time, which can potentially exceed the capacity of the reward system to reestablish homeostasis leading to long-term changes in the reward system by establishing a new homeostatic set-point (“allostasis” [120]). Allostasis is often accompanied by physiological changes (i.e., “allostatic load”), which have been described in chronic pain patients as a result of increased stress exposure [121].
To make matters worse, opioid-induced hyperalgesia is observed with administration of opioids, both clinically as well as in healthy volunteers [122–125]. As a consequence of hyperalgesia following opioid administration, increased pain sensitivity might become the new homeostatic set-point in some patients. The physiological changes associated with the new set-point have been discussed with respect to exposing these patients to higher risks of problematic opioid use [118].
Thus, increased motivational drive for reward without simultaneously increased liking of reward, combined with a drug-induced negative emotional state and hyperalgesia, may have the potential to make some chronic pain patients vulnerable to developing comorbid addictive behaviors. Drug-induced negative emotional states might contribute to diminished self-control and compulsive behaviors, whereas increased motivational drive is likely to contribute to appetitive urge and craving. Further, a formerly neutral stimulus such as a tablet or a capsule might become associated with rewarding properties by classical conditioning [126]. Now, the cue itself can trigger increased motivation and craving, possibly resulting in inappropriate drug intake, even in the absence of excessive pain, i.e., the unconditioned cue. This process is well known from the addiction literature in that drug conditioned, formerly neutral, cues cause craving and compulsive behavior [127, 128].
Not surprisingly, brain systems that appear to be central in the interaction of pain and reward also play important roles in addiction (see [129] for review). For example, drug-induced dopamine release is blunted but dopamine release in response to drug-conditioned cues is increased [127, 128, 130]. Further, activity in the orbitofrontal cortex and ventral striatum has been associated with enhanced motivational drive in addiction (see [131] for review; [132–134]). As discussed for pain, addiction-related alterations in brain systems seem to favor increased motivational drive and urge without providing the expected outcome, i.e., pleasure or relief. Such parallels between pain and addiction might mean that some chronic pain patients suffer an increased vulnerability for developing addictive behaviors.
But of course, not all chronic pain patients pharmacologically treated develop addictive behaviors. In fact, although the available evidence is limited, it appears that only a small fraction of chronic pain patients using prescribed opioids become addicted [118, 135]. The results of a recent rodent study potentially help understanding the observed low risk of addiction in pain patients. In this study, pain relief was used as negative reinforcement (the termination of an aversive stimulus) in a conditioned place preference (CPP) paradigm in rats with postsurgical pain [136]. The group found that negative reinforcement, i.e., pain relief, shares characteristics of appetitive rewards, such as a similar behavioral outcome, i.e., learning the place preference (e.g., [137–139]), and the involvement of VTA dopamine release in reinforcement (reviewed in [140]). However, a potentially important difference was that endogenous opioids in the VTA were not required to learn CPP by pain relief; in contrast, using appetitive stimuli such as nonopioidergic drugs, endogenous opioids have been repeatedly shown to be involved in learning place preferences [139, 141–143]. This might mean that pain relief by itself does not lead to opioid release in the VTA and thus might lack a euphoric sensation, which is an important driver of addiction [15]. Further, it is conceivable that chronic pain patients have protective factors such as decreased novelty seeking together with increased harm avoidance; both personality traits have been described in chronic pain patients [144], and they are negatively correlated with risk taking [145] which might act as protective factor against the development of addiction. Clinically, opioid-induced hyperalgesia has been mainly described in patients with a history of drug addiction, high-dose opioid treatment [123, 146], and in patients who are undertreated [147]. Hence, careful screening of patients before prescription of potentially addictive therapeutic agents and close monitoring is recommended and likely to decrease problematic medication use in pain patients [118].
Summary and Outlook
Taken together, pain influences reward processing on several accounts. Albeit limited, the data for acute pain are relatively clear. With respect to long-term pain, the evidence is more conflicting, mainly regarding motivational drive and risk taking. Such inconsistencies might well reflect the complex picture of chronic pain with its many constituents, including comorbidities and medication use. As discussed, changes of opioidergic as well as dopaminergic systems underlying reward processing induced by repeated exposure to unavoidable stress (chronic pain) are likely to explain the alterations of reward processing and impairments of reward-dependent learning abilities in chronic pain patients. But in order to better understand the consequences of long-term pain, it will be important to unravel which of these changes are caused by long-term exposure to pain and which by comorbidities such as emotional disturbances and dyscognition. Also, we need more studies on the effects of acute pain on specific aspects of reward processing, including hedonic responses, motivational drive, and reward-responsiveness, because their results will help interpreting studies in patients who experience pain at the time of testing. Once the links between pain and reward are better understood, it will be interesting to investigate potential moderating factors such as anxiety and mood. Since anxiety and mood influence pain perception [51, 148, 149] as well as reward processing [90, 150], it is conceivable that they have a modulating influence on the interaction between pain and reward. Clinically, an improved understanding of the consequences of pain is likely to allow helping patients more effectively by addressing such sequelae specifically. Similarly, by comprehending pain-induced changes in reward systems better, we are likely to make progress regarding the issue of potential addictive behaviors in chronic pain patients. This seems particularly important considering the huge therapeutic benefits of pain medications, and in particular opioids, on one hand and the tremendous medical and political concerns associated with their use on the other hand.
References
1.
Leknes S, Tracey I. A common neurobiology for pain and pleasure. Nat Rev Neurosci. 2008;9:314–20.PubMed
2.
Fields HL. A motivation-decision model of pain: the role of opioids. In: Flor H, Kalso E, Dostrovsky JO, editors. Proceedings of the 11th world congress on pain. Seattle: IASP Press; 2006. p. 449–59.
3.
Pecina S, Berridge KC. Opioid site in nucleus accumbens shell mediates eating and hedonic ‘liking’ for food: map based on microinjection Fos plumes. Brain Res. 2000;863:71–86.PubMed
4.
Kelley AE, Bakshi VP, Haber SN, Steininger TL, Will MJ, Zhang M. Opioid modulation of taste hedonics within the ventral striatum. Physiol Behav. 2002;76:365–77.PubMed
5.
Pecina S, Berridge KC. Hedonic hot spot in nucleus accumbens shell: where do mu-opioids cause increased hedonic impact of sweetness? J Neurosci. 2005;25:11777–86.PubMed
6.
Pecina S, Smith KS, Berridge KC. Hedonic hot spots in the brain. Neuroscientist. 2006;12:500–11.PubMed
7.
Smith KS, Berridge KC. Opioid limbic circuit for reward: interaction between hedonic hotspots of nucleus accumbens and ventral pallidum. J Neurosci. 2007;27:1594–605.PubMed
8.
Mahler SV, Smith KS, Berridge KC. Endocannabinoid hedonic hotspot for sensory pleasure: anandamide in nucleus accumbens shell enhances ‘liking’ of a sweet reward. Neuropsychopharmacology. 2007;32:2267–78.PubMed
9.
Jarrett MM, Scantlebury J, Parker LA. Effect of Delta(9)-tetrahydrocannabinol on quinine palatability and AM251 on sucrose and quinine palatability using the taste reactivity test. Physiol Behav. 2007;90:425–30.PubMed
10.
Smith KS, Tindell AJ, Aldridge JW, Berridge KC. Ventral pallidum roles in reward and motivation. Behav Brain Res. 2009;196:155–67.PubMedCentralPubMed
11.
Smith KS, Berridge KC. The ventral pallidum and hedonic reward: neurochemical maps of sucrose “liking” and food intake. J Neurosci. 2005;25:8637–49.PubMed
12.
Beaver JD, Lawrence AD, Van Ditzhuijzen J, Davis MH, Woods A, Calder AJ. Individual differences in reward drive predict neural responses to images of food. J Neurosci. 2006;26:5160–6.PubMed
13.
Pessiglione M, Schmidt L, Draganski B, Kalisch R, Lau H, Dolan RJ, Frith CD. How the brain translates money into force: a neuroimaging study of subliminal motivation. Science. 2007;316:904–6.PubMedCentralPubMed
14.
Childress AR, Ehrman RN, Wang Z, Li Y, Sciortino N, Hakun J, Jens W, Suh J, Listerud J, Marquez K, Franklin T, Langleben D, Detre J, O’Brien CP. Prelude to passion: limbic activation by “unseen” drug and sexual cues. PLoS One. 2008;3:e1506.PubMedCentralPubMed
15.
Bryant CD, Zaki PA, Carroll FI, Evans CJ. Opioids and addiction: emerging pharmaceutical strategies for reducing reward and opponent processes. Clin Neurosci Res. 2005;5:103–15.
16.
Zellner MR, Watt DF, Solms M, Panksepp J. Affective neuroscientific and neuropsychoanalytic approaches to two intractable psychiatric problems: why depression feels so bad and what addicts really want. Neurosci Biobehav Rev. 2011;35:2000–8.PubMed
17.
Venugopalan VV, Casey KF, O’Hara C, O’Loughlin J, Benkelfat C, Fellows LK, Leyton M. Acute phenylalanine/tyrosine depletion reduces motivation to smoke cigarettes across stages of addiction. Neuropsychopharmacology. 2011;36:2469–76.PubMedCentralPubMed
18.
Winkielman P, Berridge KC, Wilbarger JL. Unconscious affective reactions to masked happy versus angry faces influence consumption behavior and judgments of value. Pers Soc Psychol Bull. 2005;31:121–35.PubMed
21.
Berridge KC, Robinson TE. What is the role of dopamine in reward: hedonic impact, reward learning, or incentive salience? Brain Res Brain Res Rev. 1998;28:309–69.PubMed
23.
Wyvell CL, Berridge KC. Intra-accumbens amphetamine increases the conditioned incentive salience of sucrose reward: enhancement of reward “wanting” without enhanced “liking” or response reinforcement. J Neurosci. 2000;20:8122–30.PubMed
24.
Wyvell CL, Berridge KC. Incentive sensitization by previous amphetamine exposure: increased cue-triggered “wanting” for sucrose reward. J Neurosci. 2001;21:7831–40.PubMed
25.
Avila C, Parcet MA, Barros-Loscertales A. A cognitive neuroscience approach to individual differences in sensitivity to reward. Neurotox Res. 2008;14:191–203.PubMed
26.
Gray JA. The psychophysiological basis of introversion-extraversion. Behav Res Ther. 1970;8:249–66.PubMed
27.
Gray JA, McNaughton N. The neuropsychology of anxiety: an enquiry in to the functions of the septo-hippocampal system. Oxford: Oxford University; 2000.
28.
van der Linden D, Taris TW, Beckers DGJ, Kindt KB. Reinforcement sensitivity theory and occupational health: BAS and BIS on the job. Pers Individ Dif. 2007;42:1127–38.
29.
Smillie LD, Pickering AD, Jackson CJ. The new reinforcement sensitivity theory: implications for personality measurement. Pers Soc Psychol Rev. 2006;10:320–35.PubMed
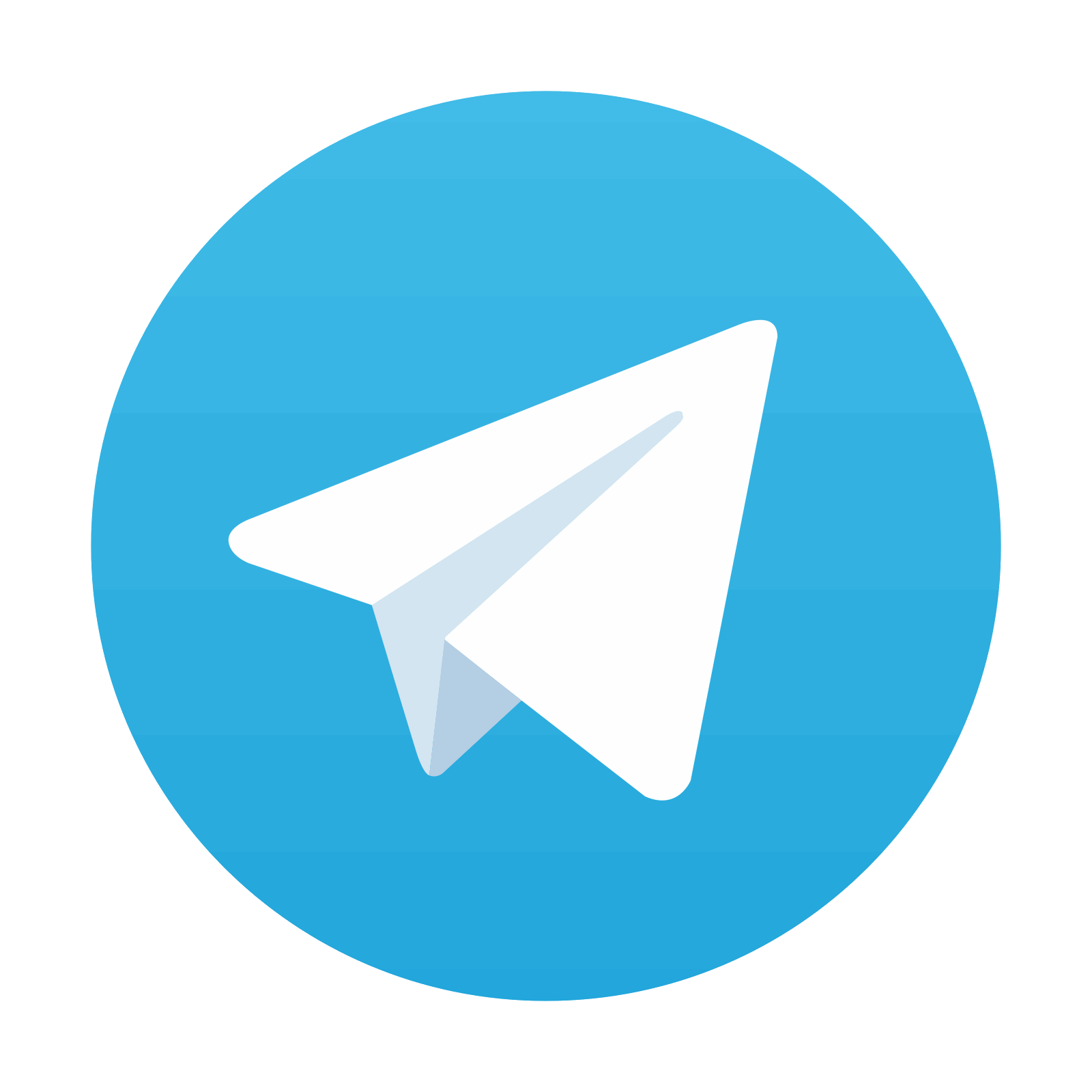
Stay updated, free articles. Join our Telegram channel

Full access? Get Clinical Tree
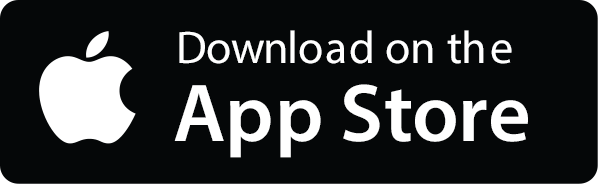
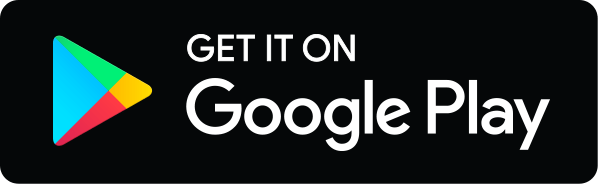