CLINICAL CASE | Anterior Temporal Lobe Degeneration
A 67-year-old woman was dining with her family when she was not able to recognize a food she commonly ate. She had been an empathetic person but recently has begun to be self-centered and unconcerned about others’ feelings, including those of her daughter, with whom she was close. She had been socially dominant and extraverted, but recently lost that dominance, and has become neurotic and introverted. She had been a successful travel agent and had visited many countries worldwide, but she was now unable to recall the names of many of the places she had visited multiple times.
Over the next 2 years, her condition progressed, so that she was unable to recognize familiar people, words, and objects. Despite having normal calculation abilities, she stopped controlling her own finances. Around this time, her eating behavior changed. She also expressed socially inappropriate behaviors. For example, she developed a preference for sweets and condiments, and sometimes she ate condiments as food. She also tried to eat nonfood items. Her sensory and motor functions were unaffected, as were her visuospatial functions and episodic memory. Her speech and language were grammatically correct and fluent.
Figure 16–1A is from the patient, showing clear and marked degeneration of the right anterior temporal lobe; Figure 16–1B is an MRI from a healthy person at a similar placement within the anterior temporal lobe. Degeneration is manifested both as a reduction in the gray and white matter of the anterior temporal lobe and insular region, as well as a corresponding expansion of the lateral sulcus and other temporal lobe sulci (eg, rostral superior temporal sulcus). Notice that other brain regions (eg, head of caudate nucleus) appear normal.
Answer the following question based on your reading of this chapter.
1. Degeneration in which part of the temporal lobe accounts for the patient’s impairments?
Key neurological signs and corresponding damaged brain structures Frontotemporal dementiaThe patient is suffering from frontotemporal dementia, a progressive degenerative disease characterized by loss of parts of the frontal and/or anterior temporal lobe; it can be lateralized. In this patient, it is primarily right-sided.
Brodmann’s area 38, amygdala, and corticocortical connectionsBrodmann’s area 38, the cortex of the temporal pole (see Figure 2–19), has corticocortical interconnections with other limbic cortical areas, including orbitofrontal cortex; it is interconnected with the amygdala, as well. These areas form a network, so that the personality changes, oral tendencies, and semantic impairments are difficult to attribute to a single structure.
ReferencesGainotti G, Barbier A, Marra C. Slowly progressive defect in recognition of familiar people in a patient with right anterior temporal atrophy. Brain. 2003;126:792-803.
Gorno-Tempini ML, Rankin KP, Woolley JD, Rosen HJ, Phengrasamy L, Miller BL. Cognitive and behavioral profile in a case of right anterior temporal lobe neurodegeneration. Cortex. 2004;40(4-5):631-644.
Mummery CJ, Patterson K, Price CJ, Ashburner J, Frackowiak RSJ, Hodges JR. A voxel-based morphometry study of semantic dementia: relationship between temporal lobe atrophy and semantic memory. Ann Neurol. 2000;47:36-45.
Olson IR, Plotzker A, Ezzyat Y. The enigmatic temporal pole: a review of findings on social and emotional processing. Brain. 2007;130(Pt 7):1718-1731.
FIGURE 16–1
Frontotemporal dementia. A. Coronal MRIs from a patient with frontotemporal dementia B. Coronal MRI from a healthy person. (A, Reproduced with permission from Gainotti G, Barbier A, Marra C. Slowly progressive defect in recognition of familiar people in a patient with right anterior temporal atrophy. Brain. 2003;126:792-803. B, Courtesy of Dr. Joy Hirsch, Columbia University.)

The limbic system is a diverse collection of cortical and subcortical regions that are crucial for normal human behavior. Who you are—your memories, your unique personality, your thoughts, your emotions—in large measure is determined by the functions of the diverse brain regions that comprise the limbic system. Virtually all psychiatric diseases involve dysfunction of these structures.
Nineteenth century neurologists and anatomists recognized that damage to particular parts of the human brain was associated with disorders of emotion and memory. These lesions, unlike those of the cerebellum, occipital lobe, or cortical regions around the central sulcus, for example, spared perception and movement. This research led to the understanding that the neural systems for reward, emotions, and memory are distinct from the sensory and motor systems and, hence, are grouped into a single system called the limbic system. The term limbic derives from the Latin word limbus for “border,” because many of the structures engaged in these functions encircle the diencephalon on the medial brain surface and are at the border between subcortical nuclei and the cerebral cortex.
However, the more that is understood about the myriad functions of limbic system structures, the less helpful it is to adhere to the notion of a single system. It becomes more meaningful to consider the component functional systems. As a consequence, the term limbic system is gradually being abandoned in favor of a more functionally descriptive terminology. Nevertheless, the notion of the limbic system has some utility. Brain structures that comprise the limbic system have been conserved throughout much of vertebrate evolution, reflecting the common and important need for the functions they serve.
The basic organizational plan of the circuits for reward, emotions, and memory appears to be different from the sensory and motor systems. The different sensory and motor systems consist of structurally and functionally independent regions that are interconnected only at the highest levels of processing. This functional independence makes sense. For example, although perceptions are enriched when information from different modalities is combined, you can nevertheless identify an apple by touch alone or a dog by the sound of a bark. In contrast, circuits for reward, emotions, and memory are highly integrated from the start. This no doubt reflects the fact that reward and emotion depend on the concurrent analysis of diverse sensory information and actions, and therefore are highly integrated behaviors. So, too, are memories. The sight of an old house and children playing in the yard can evoke vivid recollection of times spent during childhood.
This chapter first considers the components of the limbic system in relation to their generalized roles in reward, emotions, and memory. Then the chapter reexamines the same structures from the perspective of their spatial interrelations, their tracts, and their connections.
The circuits for reward, emotions, and memory have tremendous anatomical and functional diversity, involving an interplay between cortical and subcortical structures (Table 16–1). Components of the limbic system are highly interconnected, just as their functions are interdependent. And not surprisingly, it is difficult to categorically assign one or another function to each component of the limbic system. Even so, major functional distinctions emerge after disturbance to one or another structure, such as after removal for intractable epilepsy or after stroke.
Major brain division | Structure | Component part |
---|---|---|
Cerebral hemisphere (telencephalon) | Limbic association cortex | Orbitofrontal |
Cingulate | ||
Entorhinal | ||
Temporal pole | ||
Perirhinal | ||
Parahippocampal | ||
Hippocampal formation | Hippocampus (Ammon’s horn) | |
Subiculum | ||
Dentate gyrus | ||
Amygdala | Corticomedial | |
Basolateral | ||
Central nucleus1 | ||
Ventral striatum | Nucleus accumbens | |
Olfactory tubercle | ||
Ventromedial caudate and putamen | ||
Diencephalon | Thalamus | Anterior nucleus |
Medial dorsal nucleus | ||
Midline nuclei | ||
Hypothalamus | Mammillary nuclei | |
Ventromedial nucleus | ||
Lateral hypothalamic area | ||
Epithalamus2 | Habenula | |
Midbrain | Portions of the periaqueductal gray matter and reticular formation |
The hippocampal formation is central to memory and the amygdala, to emotions (Figure 16–2A). In addition, the amygdala participates in the acquisition, consolidation, and recall of emotional memories. Two other subcortical structures— the ventral tegmental area and the ventral striatum and other components of the emotional loop of the basal ganglia (see Figure 14–2)—are key to reward and other reward-related behaviors, punishment, and aspects of decision. Recall that the ventral striatum comprises the nucleus accumbens and adjoining parts of the ventral caudate nucleus and putamen. And all of these structures are interconnected with the limbic association cortex (Figures 16–3 and 16–4). These cortical areas receive information from integrative thalamic nuclei, higher-order sensory areas; and from the other cortical association areas. In turn, they project to the subcortical limbic system structures.
FIGURE 16–3
Midsagittal view of the right cerebral hemisphere, with the brain stem removed. The limbic association areas are indicated by the different shaded regions. The lines drawn on the cingulate cortex roughly divide it into anterior, middle, and posterior regions, as described in the text. The inset shows the prefrontal association cortex and the parietal-temporal-occipital association cortex.

The Limbic Association Cortex Is Located on the Medial Surface of the Frontal, Parietal, and Temporal Lobes
There are three major cortical association areas: (1) the parietal-temporal-occipital area, (2) the dorsolateral prefrontal association cortex (Figure 16–3, inset), and (3) the limbic association cortex. The limbic association cortex consists of morphologically and functionally diverse regions on four sets of gyri primarily on the medial and orbital surfaces of the cerebral hemisphere (Figures 16–3 and 16–4): the cingulate gyrus, the parahippocampal gyrus, the orbitofrontal and medial frontal gyri, and the gyri of the temporal pole. On the ventral brain surface (Figure 16–4), the lateral boundary of the limbic association cortex corresponds approximately to the collateral sulcus.
The cingulate gyrus, receiving its major thalamic input from the anterior nucleus, comprises three functional regions: rostral, middle, and posterior (Figure 16–3). The rostral portion of the cingulate gyrus is important in emotions, with connections with the amygdala, orbitofrontal, and insular cortex. We learned that a portion of this cortex receives information about physically painful stimuli. This portion is also involved in “emotional pain” of certain social situations (Figure 2–7). We also learned in Chapter 5 that the anterolateral system projects to the medial dorsal nucleus of the thalamus to convey physical pain information to the anterior cingulate gyrus. The portion under the genu of the corpus callosum, sometimes termed the subgenual region of the cingulate gyrus, is associated with the mood disorder, depression. This region is the target of therapeutic brain stimulation to ameliorate depression in patients who are refractory to pharmacological antidepressant therapy. The middle portion corresponds to the cingulate motor areas (see Chapter 10; Figure 10–7B). This portion may be involved in aspects of movement control driven by emotions and reward. The posterior cingulate appears to be more related to higher-order sensory functions and memory. The parahippocampal gyrus contains several subdivisions that provide information to the hippocampal formation (Figure 16–4). These areas are discussed below. Together, the cingulate and parahippocampal gyri form a C-shaped ring of cortex that partially encircles the corpus callosum, diencephalon, and midbrain (Figure 16–2). The cingulum (or cingulum bundle) is a collection of axons that courses in the white matter deep within the cingulate and parahippocampal gyri. Cortical association fibers course in the cingulum and terminate in the parahippocampal gyrus.
Rostral to the cortical ring are the medial frontal and orbitofrontal gyri. These areas are central to reward and decision making. A famous case study called attention to the orbitofrontal cortex in emotions. Phineas Gage was a railway foreman who was seriously injured in an accident in which an explosion drove a metal rod through his skull, largely ablating the orbitofrontal cortex and adjoining prefrontal cortex on one side of the brain. He survived but was a changed man. He was no longer a responsible worker, he became “short-tempered, capricious, and profane;” he was “no longer Gage.” These changes occurred without major defects in intellect. Frontal lobe research led to development of the prefrontal lobotomy—whereby physical removal of orbitofrontal cortex and adjacent areas or section of its connections—to quell the disruptive behaviors of psychiatric disease. The orbitofrontal cortex receives information from all sensory modalities, typically via higher-order sensory cortical regions, together with inputs from subcortical reward centers (see below). It is thought to integrate this information for decision making and to evaluate the hedonic value of stimulation.
The cortex of the temporal pole, corresponding to Brodmann’s area 38 (Figures 16–3 and 16–4; see Figure 2–19) is interconnected with the orbitofrontal cortex and subcortically with the amygdala and hypothalamus. Lesion of this part of the temporal lobe can produce personality changes, such as social withdrawal. In this chapter’s case study, the person with degeneration of the temporal pole changed from being highly extroverted and empathetic to introverted and cold. Indiscriminate eating habits also are reported.
Important insights into the function of the hippocampal formation have been obtained by studying the behavior of patients whose medial temporal lobe either was damaged because of a stroke or was ablated to ameliorate the serious symptoms of temporal lobe epilepsy. In one of the most extensively examined cases, this region was removed bilaterally from a patient referred to as H.M. After surgery, H.M. lost the capacity for consolidating short-term memory into long-term memory, but he retained the memory of events that occurred before the lesion. This is termed anterograde amnesia. The impairment was selective for consolidating explicit memories (also termed declarative memories), such as the conscious recollection of facts. By contrast, H.M. and other patients with hippocampal (or medial temporal lobe) damage are capable of remembering procedures and actions (ie, implicit or nondeclarative memory), and they retain the capacity for a variety of simple forms of memory. More common than surgical ablation, sometimes after a severe heart attack, patients suffer bilateral damage to a key part of the hippocampal formation. During a heart attack, circulation of blood to the brain can become compromised because of insufficiency in the pumping action of the heart. This brain injury results because certain neurons in the hippocampal formation require consistently high circulating blood oxygen levels. What has emerged from this research is that the hippocampal formation is involved in the long-term consolidation of explicit memory. It is thought that the memories themselves reside in the higher-order association areas of the cerebral cortex.
Whereas the hippocampal formation is best known for its role in memory consolidation, it has also been implicated in the body’s response to stress and emotions. Interestingly, animal and human fMRI studies suggest that the posterior part of the hippocampal formation is more important for explicit memory, cognition, and spatial memory, while the anterior portion is more related to stress and emotions. Interestingly, London taxicab drivers, who must master the complex London street map, have a larger posterior hippocampal formation than control subjects. Located anteriorly, there is a division related to stress and emotions. Further, the size of the hippocampal formation is reduced in schizophrenia, linking it with human psychiatric disease.
The hippocampal formation comprises three anatomical components, each with distinctive morphologies and connections (Figure 16–5; Table 16–1; see Box 16–1): the dentate gyrus, the hippocampus proper, and the subiculum. (The nomenclature of the hippocampal formation is variable, and exactly which components are considered to be part of this structure may differ, depending on the source.) The three components are organized roughly as parallel strips running antero-posteriorly within the temporal lobe and together forming a cylinder (Figure 16–5). These strips are initially a flattened sheet located on the brain surface, but during prenatal development they become buried within the cortex (see Figure 16–16A). The flat sheet also folds in a complex manner to assume its mature configuration, which resembles a jelly-roll pastry. The dentate gyrus—together with the subventricular zone of the lateral ventricle, which was discussed in Chapter 9 (see Box 9–1)—are the two brain sites for neurogenesis in the mature brain.
FIGURE 16–5
The general spatial relations of components of the hippocampal formation, its efferent pathway (fornix), and the entorhinal cortex are shown. The middle portion of the hippocampal formation is distinguished in the schematic view. A coronal slice through the hippocampal formation (cut end, anteriorly) reveals a cylindrical shape and circular sequence of component structures (i.e., dentate gyrus, hippocampus, and subiculum). These components are present at all anterior-to-posterior levels; each forming a longitudinal strip along the antero-posterior axis of the cylinder.

Box 16–1 Circuits of the Hippocampal Formation and Entorhinal Cortex Are Important for Memory
Memory impairment after damage to the hippocampal formation and certain adjoining cortical structures is selective for explicit memories (also termed declarative memories). Consolidation of both forms of explicit memories is impaired: semantic memory, such as knowledge of facts, people, and objects, including new word meaning, and the episodic memory of events that have a specific spatial and temporal context, such as meeting a friend last week. Formation of spatial memories is also impaired, such as being able to navigate around a familiar city. By contrast, patients with hippocampal (or medial temporal lobe) damage are capable of remembering procedures and actions (ie, implicit or nondeclarative memory), and they retain the capacity for a variety of simple forms of learning and memory.
The three divisions of the hippocampal formation—the dentate gyrus, hippocampus, and subiculum, and any component parts—each have a relatively simple circuit, compared with other cerebral cortical areas. Moreover, the basic circuit is the same from anterior in the temporal lobe, posteriorly. In this way, it is much like that of the cerebellum in which local circuits were the same for the different cerebellar cortical regions. In a slice through the hippocampal formation (Figure 16–17), we see that pyramidal cells of the entorhinal cortex send their axons to the dentate gyrus, roughly in the same coronal plane as the hippocampal formation, to synapse on granule cells. This is the perforant pathway. Granule cell axons, termed mossy fibers, synapse on pyramidal cells of one subregion of the hippocampus, termed the CA3 region, where neurons, in turn, send their axons (called the Schaefer collaterals) to neurons of the CA1 region. (These axon collaterals spare the CA2 region.) The subiculum receives the next projection in the sequence, from the CA1 region, and it projects back to the entorhinal cortex. Both CA1 and the subiculum also project axons into the fornix, primarily to the septal nuclei and mammillary bodies, respectively. Additional parallel projections from entorhinal cortex to the hippocampus and subiculum are also important. It is not yet known how the myriad connections of the entorhinal cortex and hippocampal formation are organized to play a pivotal role in memory consolidation, spatial memory and navagation, and other aspects of cognition. However, an important clue exists: The strength of many synapses in the hippocampal formation can be modified under various experimental conditions.
A model for the functional organization of the hippocampal formation is based on its anatomical circuitry. Information that is first processed in the higher-order association areas on the lateral surface of the cerebral hemisphere, such as the parietal-temporal-occipital association area, is next processed in the limbic association cortex on the medial temporal lobe. This processing takes place in three key areas: the perirhinal cortex, the parahippocampal cortex, and the entorhinal cortex (Figure 16–3). From here, information is transmitted to the hippocampal formation (Figure 16–6), where further processing results in changes in the amount or timing of activity of certain populations of neurons. The complex neural responses comprise a “representation” of the memory, which unfortunately is not well understood. Finally, via two sets of return projections to the cortex—back to entorhinal cortex directly and, via the fornix, to the mammillary bodies and anterior thalamus to the cingulate cortex—this hippocampal memory representation enables consolidation of explicit and spatial memories in the association areas.
FIGURE 16–6
Serial and parallel hippocampal circuits. A. Cortical inputs to the hippocampal formation. B. Subcortical outputs via the fornix. There are also cortical projections from the hippocampal formation back to the entorhinal cortex, which projects back to the cortical areas from which it received input.

The hippocampal formation receives complex sensory and cognitive information from a portion of the limbic association cortex termed the entorhinal cortex (Figures 16–3, 16–4, and 16–5). In fact, the hippocampal formation works so closely with the adjoining entorhinal cortex that the two are functionally inseparable. The entorhinal cortex, located on the parahippocampal gyrus adjacent to the hippocampal formation, collects information from other parts of the limbic association cortex (perirhinal and parahippocampal cortex) as well as from other association areas (Figure 16–6A). Extensive processing of information occurs within the hippocampal formation, within a prominent serial circuit, in which information is projected in sequential steps (see Box 16–1). There is also a parallel circuit, in which information from the entorhinal cortex projects directly to each hippocampal component. Combined serial and parallel processing within neural circuits is also a feature of sensory and motor pathways.
The output neurons of the hippocampal formation are pyramidal neurons, similar to the neocortex covering most of the cerebral hemisphere, and they are located in the hippocampus and subiculum. The dentate gyrus contains neurons, termed granule cells, that make connections only within the hippocampal formation. Pyramidal neurons have axon branches that collect on the surface of the hippocampal formation. Eventually these axons form a compact fiber bundle, the fornix (Figures 16–2 and 16–5), which projects to other subcortical telencephalic and diencephalic structures. The hippocampal formation, together with the fornix, has a C-shape. Two output systems can be distinguished within the fornix, from the subiculum and the hippocampus (Figures 16–6B and 16–7B). Although these systems are involved in the cognitive aspects of memory, it is not yet understood how their functions differ.
From the subiculum, axons synapse mostly in the mammillary bodies of the hypothalamus (Figures 16–2 and 16–6B). This projection completes an anatomical loop: Via the mammillothalamic tract, the mammillary body projects to the anterior nuclei of the thalamus, which project to the cingulate gyrus (Figure 16–6B). The cingulate gyrus provides information to the entorhinal cortex, which projects to the hippocampal formation. In 1937, James Papez postulated that this pathway plays an important role in emotion. It is now known that the circuit named in his honor is part of a complex network of bidirectional connections and that many components of this network play an important role in memory. Some fornix fibers from the subiculum project to the amygdala. This may be part of the circuit for consolidating emotional memories.
From the hippocampus, most axons do not synapse in the mammillary bodies, but rather, in several other locations, including the septal nuclei, located more rostrally in the forebrain in close apposition to the septum pellicudum (Figure 16–6B). Little is known about the function of septal nuclei. In a fascinating series of experiments in the early 1950s, laboratory rats, when given the choice of receiving either electrical stimulation of the septal nuclei or food and water, preferred the electrical stimulation. Investigators reasoned that this region is a so-called pleasure center that likely plays an important role in regulating highly motivated behaviors, such as reproductive behaviors or feeding. The septal nuclei give rise to a cholinergic (see Figure 2–3A) and GABAergic projection, via the fornix, back to the hippocampal formation. This return septal projection is important in regulating hippocampal activity during certain active behavioral states.
The fornix is an extremely large tract, with over one million heavily myelinated axons on each side. This number is comparable to the number of myelinated axons in one medullary pyramid or an optic nerve. Despite its size, a major target of axons of the fornix is the ipsilateral mammillary body, whose output is also highly focused, on the anterior thalamic nuclei. How can the hippocampal formation, with such a focused subcortical projection, have a generalized role in memory? One answer is that the fornix is not the only major output of the hippocampal formation. The subiculum and hippocampus also project back to the entorhinal cortex (Figure 16–6C), which, in turn, has diverse efferent corticocortical connections to the prefrontal cortex, orbitofrontal cortex, parahippocampal gyrus, cingulate gyrus, and insular cortex (Figure 16–6B). And collectively these cortical areas also have widespread projections. Through the divergence of connections emerging from the entorhinal cortex to cortical association areas, the hippocampal formation can influence virtually all association areas of the temporal, parietal, and frontal lobes, as well as some higher-order sensory areas, after as few as three synapses. The divergence in the cortical output of the hippocampal formation parallels the widespread convergence of its inputs, also via the entorhinal cortex, from association areas.
The amygdala (sometimes termed the amygdaloid complex) is a collection of morphologically, histochemically, and functionally diverse nuclei. Located largely within the rostral temporal lobe (Figure 16–2), the main portion of the amygdala is almond-shaped (amygdala is Greek for “almond”). One of its output pathways, however, the stria terminalis, and one of its component nuclei, the bed nucleus of the stria terminalis, are C-shaped (Figure 16–7). Axons of the other output pathway of the amygdala, the ventral amygdalofugal pathway, take a somewhat more direct route to their targets.
FIGURE 16–7
Principal connections of the amygdala. The inset shows schematically the three divisions of the amygdala. A. The basolateral nuclei are reciprocally connected with the cortex of the temporal lobe, including higher-order sensory areas and association cortex. The basolateral amygdala also projects to the medial dorsal nucleus of the thalamus, the basal nucleus, and the ventral striatum. B. The central nuclei receive input from the brain stem, especially from visceral afferent relay nuclei (ie, solitary nucleus and parabrachial nucleus). The targets of its efferent projections include the hypothalamus and autonomic nuclei in the brain stem. C. The cortical nuclei have reciprocal connections with the olfactory bulb and efferent projections via the stria terminalis to the ventromedial nucleus of the hypothalamus.

Amygdala circuits are preferentially involved in emotions and their overt behavioral expressions. The functions of amygdala circuits are therefore similar to the functions originally proposed for the entire limbic system. What stimuli are responded to, how overt responses to these stimuli are organized, and the internal responses of the body’s organs are all dependent on this subcortical structure. Following damage to the amygdala, people lose the ability to recognize the affective meaning of facial expression, especially threatening faces. People also fail to recognize the emotional content of speech. Given the defects observed with its damage, it is not surprising that the amygdala is a central figure in emotion regulation, especially in relation to fear. For example, analysis of staring eyes, a vocalization, and body posture can lead to a set of potential emotional outcomes, such as fear or anxiety, and a set of possible actions, such as fleeing or attacking a potential foe. In animals, electrical stimulation of the amygdala, depending on the particular site, can evoke diverse defense reactions and visceral motor responses. The numerous nuclei of the amygdala can be divided into three principal nuclear groups (Figure 16–8): basolateral, central, and cortical. Each group has different connections and functions.
FIGURE 16–8
Brain regions important in addiction and reinforcement. A. The ventral tegmental area gives rise to a dopaminergic projection to the amygdala and ventral striatum that is important in various aspects of addiction. The ventral tegmental area also projects to the prefrontal cortex. B. Transverse section through the midbrain-diencephalon junction, showing the location of the ventral tegmental area and substantia nigra, both of which contain dopaminergic neurons. C. An expanded view of the region containing components of the limbic loop of the basal ganglia.

The basolateral nuclei (Figure 16–7A) comprise the largest division of the amygdala. These nuclei are thought to attach emotional significance to a stimulus. The basolateral nuclei receive information about the particular characteristics of a stimulus from higher-order sensory cortical areas in the temporal and insular cortical areas and from association cortex. Limbic association cortex conveys this information to the amygdala to link particular stimuli, such as seeing a particular object or hearing a certain sound, with particular emotions. The amygdala is an important target of the ventral stream for object recognition (see Figures 7–15 and 7–16). Importantly, the amygdala and hippocampal formation receive somewhat different kinds of sensory information. Whereas the amygdala receives highly processed sensory information, it retains its modality characteristics (eg, visual or auditory). On the other hand, the hippocampal formation receives more integrated sensory information that is thought to reflect complex features of the environment, such as spatial relationships and contexts. For example, when you see a snake, you may feel threatened and fearful. Visual pathways through the ventral portion of the temporal lobe convey information about the snake to the amygdala. The amygdala uses this information to organize your response, both the emotions you feel and your overt behavior to this potential danger. The hippocampal formation is thought to be important in learning the complex environmental setting, or context, in which the snake was seen.
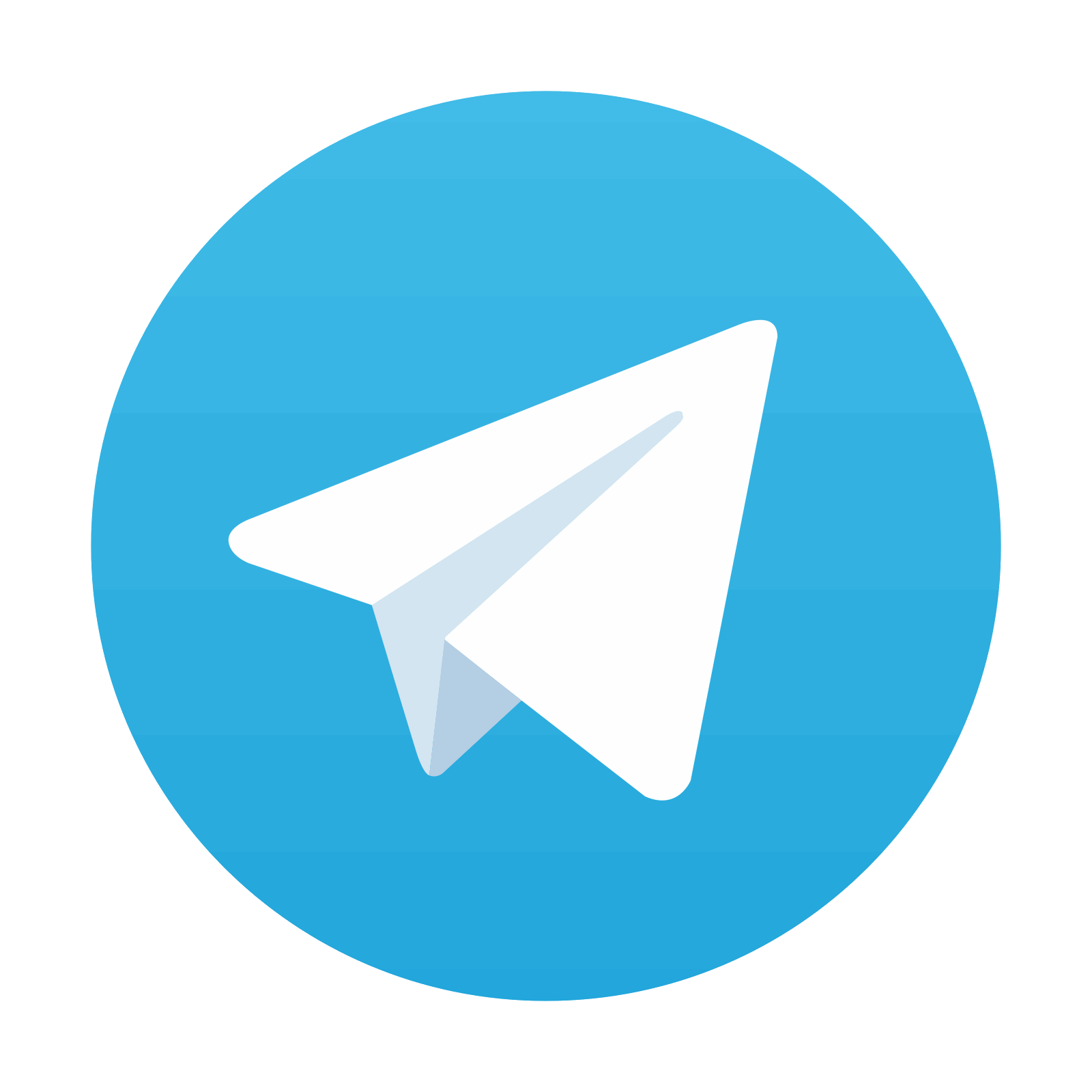
Stay updated, free articles. Join our Telegram channel

Full access? Get Clinical Tree
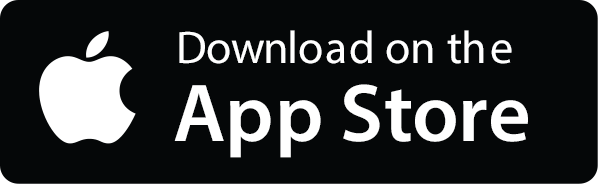
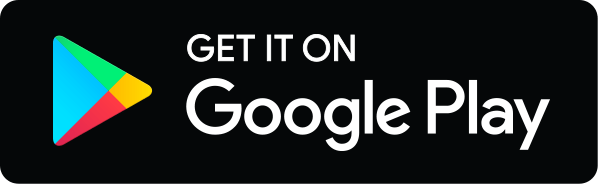