5 The Mechanisms of Pain from Intervertebral Discs Todd F. Alamin and Vijay Agarwal Any therapeutic endeavor relies on an understanding of the mechanism by which the pain is produced. Is the pain originating in the intervertebral disc (IVD) an inflammatory process, or a purely mechanical one? From which part of the disc does the pain originate? The answers are critical to the patient for whom treatment is contemplated: the information put forth in this chapter will hopefully aid in this analysis. Pain that originates at the level of the disc can be divided into two categories: radicular pain (typically manifested as leg pain caused by a process originating at the level of the root) and discogenic pain (typically manifested as axial back pain caused by mechanical loading of a pathologically degenerated disc). These broad categories of pain may have features in common, but their distinct clinical behavior warrants categorization when considering their pathophysiology. The mechanism of pain production can be thought of first as the stimulation and firing of a nociceptive afferent, and second, as the conveyance of this signal through the signal processing pathway to the level of consciousness. This chapter begins with a description of the signal processing pathway, and then moves to the research area of focus: characteristics of relevant nociceptive afferents and the factors that lead to their firing. Radicular pain and discogenic pain will be addressed sequentially—deeper knowledge of one will facilitate a better understanding of the other. A comprehensive understanding of lumbar spine innervation from an anatomical point of view is critical when analyzing potential mechanisms of pain caused by the IVD. There are two pathways by which afferent information can be transmitted from the disc to the dorsal root ganglion: segmentally, via the sinuvertebral nerve,1–3 and nonsegmentally, through the paravertebral sympathetic trunk.2,3 The spinal cord sends off a ventral and a dorsal root, which combine to form the spinal nerve. The ventral root is the efferent motor root; all motor fibers, both somatic and autonomic, leave the spinal cord via the ventral roots. The dorsal root is the afferent sensory root; at its distal end, before the formation of the spinal nerve, is the dorsal root ganglion (DRG), containing the soma of the afferent nerve fibers. DRG neurons are of the pseudo-unipolar type, conveying sensory information from the receptor to the soma, and from the soma to the junction in the dorsal horn of the spinal cord, via two independent axons. The dorsal and ventral roots combine to form the spinal nerve, which divides into the dorsal and ventral primary rami, each containing both motor and sensory fibers. The dorsal primary ramus then splits into the medial and lateral branches for the supply of the muscles and skin of the posterior trunk; the larger ventral primary ramus supplies the anterolateral trunk and limbs. Ventral primary rami from different levels combine in the cervical, lumbar, and sacral regions to form peripheral plexuses. The sympathetic trunk is a group of nerve fibers that originates as the internal carotid nerve and runs from the carotid canal in the skull to the coccyx, anterolateral to the vertebral column, receiving contributions along the way from nerve roots exiting the spine through the thoracic and upper two lumbar segments. Cell bodies of preganglionic neurons in the sympathetic system reside in the intermediolateral cell column in the lateral horn of the spinal cord, from the T1-L2 levels. Preganglionic fibers travel via the ventral root at each spinal level, through the T1-L2 spinal nerves and ventral rami to join the sympathetic trunk via the communicating ramus (described below). Paravertebral ganglia that exist as fusiform expansions at the junction of the communicating ramus (and the sympathetic trunk along its course) are locations of interconnection among these autonomic fibers and contain the cell bodies of the postganglionic nerves. The sympathetic trunk travels superiorly with the internal carotid artery through the carotid canal into the skull. Inferiorly, the bilateral chains converge in front of the coccyx to form the ganglion impar. The two branches of the sympathetic trunk contributing to the spinal nerve are the gray ramus communicans (containing unmyelinated postganglionic sympathetic fibers) and white ramus communicans from the thoracic, first, and second lumbar nerves (containing myelinated preganglionic sympathetic fibers). Each sinuvertebral nerve is formed by the union of a branch of the ventral ramus of the spinal nerve at that level and a branch of the sympathetic trunk via the segmental gray ramus communicans. The posterior and posterolateral anulus and posterior longitudinal ligament are innervated by the sinuvertebral nerve, direct sensory branches from the ventral primary ramus, and, to a lesser degree, rami communicantes from the sympathetic trunk.4,5 The lateral and anterior aspects of the anulus, as well as the anterior longitudinal ligament, are innervated via rami communicantes from the sympathetic trunk, along with direct branches from the sympathetic trunk.3,4,6,7 The innervation of the endplate is 2-fold: intraosseous nerves that are direct branches of the primary ventral rami follow vasculature channels into the endplate,8 and the basivertebral nerve—a branch of the sinuvertebral nerve—enters the endplate centrally and posteriorly9 via the posterior neurovascular foramen. In a nondegenerated sheep disc, Fagan et al10 found a limited nerve supply confined to the outermost structures; there was no significant difference in overall density of endplate and anulus innervation, with the richest area of innervations in the superficial anulus. No innervation was found in the inner anulus or nucleus. In human patients with degenerative disc disease, Brown et al11 presented similar findings, with no innervation detected in disc inner anulus or nucleus pulposus (NP). Animal models have been the basis for extensive characterization of lumbar disc innervation.12–17 The fluorogold injection technique, in which tracer is taken up at the level of the nerve ending and then transported up the afferent axon, has been used to establish the transmission pathway from the level of the disc.18 Investigators have found that particles injected into the disc can then be detected in primary sensory neurons in the dorsal root ganglia and primary sensory fibers in the posterior horn of the spinal cord not only at the injected level but at multiple levels—clearly documenting the polysegmental nature of the afferent nerve supply of the disc. This polysegmental origin of innervation is not only the case for the somatic but also for the autonomic innervation of the disc via the sympathetic trunk. Vertebral endplate innervation, predominantly somatic via the sinuvertebral nerve and branches of the primary ventral ramus, is also polysegmental in origin. In both the somatic and autonomic pathways, stimulation of afferents results in contralateral dorsal root ganglia involvement. The first comprehensive description of the source and pattern of lumbar IVD innervation in humans is based on the meticulous dissection of four adult cadaveric spines.4 The sinuvertebral nerve was found to arise from both the ventral primary ramus (somatic origin) and a ramus communicans (sympathetic origin). The nerve was found to enter the vertebral canal through the intervertebral foramen by crossing the vertebral body just caudal to the pedicle. Lesser branches of the nerve passed medially and caudally from the intervertebral foramen and branched over the IVD at the level of the origin of the primary (or parent) nerve. The sinuvertebral nerve gives rise to both ascending and descending branches, but Bogduk and others found that the ascending branch was consistently much larger.4 This major branch of the sinuvertebral nerve courses parallel to the posterior longitudinal ligament, giving off transverse branches that pass deep to the ligament to supply the anulus (Fig. 5.1). Fig. 5.1 The sinuvertebral nerves. The arrow (C) indicates the cranial end of the specimen. (A) Lateral view of a right L1 intervertebral foramen, showing the origin of the sinuvertebral nerve. (B) Medial view of the same foramen as in A, showing the recurrent course of the nerve through the foramen. The nerve has been lifted by a 0- to 75-mm probe. The sinuvertebral nerves. The arrow (C) indicates the cranial end of the specimen. (C) The course of a left L2 sinuvertebral nerve within the vertebral canal. (D) A close-up view of the lesser descending branch of the nerve in C. P, pedicle; LF, ligamentum flavum; PLL, posterior longitudinal ligament; IVD, intervertebral disc; drg, dorsal root ganglion; dr, dorsal ramus; vr, ventral ramus; rc: ramus communicans; svn, sinuvertebral nerve; ar, autonomic root of svn; sr, somatic root of svn; m, major ascending branch of svn; l, lesser descending branch of svn; b, branches to posterior longitudinal ligament. (From Bogduk N, Tynan W, Wilson AS. The nerve supply to the human lumbar intervertebral discs. J Anat 1981;132[Pt 1]: 39–56. Reprinted with permission.) Each ventral primary ramus receives at least one rami communicantes, but more often two or more rami extend from the sympathetic trunk around the vertebral body and through the psoas muscle. Bogduk described “paradiscal” rami as fibers that cross the IVD on its surface, instead of passing through the muscle belly of the psoas adjacent to the disc. These paradiscal rami are likely the predominant source of autonomic input to the ventral primary ramus. The autonomic component of disc innervation of lower lumbar levels is not affected by root blocks performed below the L2 level; these sympathetic fibers predominantly enter the spinal canal as part of the L2 root. Clinical reports of significant relief of low back pain via L2 selective nerve root blocks1,14,19–25 suggest that these autonomic fibers may play a role in some patients with low back pain, but this role has not been clearly established. It seems likely that this autonomic innervation is important in patients with neuropathic pain states, and less so in patients with low back pain that is more clearly mechanical in nature. The sources of somatic afferent information from the disc are sinuvertebral nerve, basivertebral nerve, and direct branches of the primary ventral rami, which either supply the posterior anulus or course with the intraosseous vessels to the endplate. This information is collected polysegmentally via ipsilateral and contralateral DRGs and is then transmitted to the dorsal horn of the spinal cord. In the spinal cord, pain signals synapse on the superficial layer of the dorsal horn and travel via the spinothalamic tract to the thalamus and somatosensory and pain centers in the cortex. Information from the autonomic afferent innervation of the lower lumbar discs is predominantly collected at the level of the L2 dorsal root ganglia is transmitted to the dorsal horn, and subsequently courses proximally into the central nervous system (Fig. 5.2). In 1934, Mixter and Barr’s description of sciatica caused by disc herniation hypothesized that this complaint was caused solely by the mechanical deformation of the root affected by the disc herniation.26 Imaging studies and the finding of relief of sciatica with fragment removal have rendered the mechanical deformation of the root an obvious point of focus, but animal studies and clinical investigations have painted a more complex picture of the clinical syndrome. Several animal models of sciatica have been developed that involve the simple application of NP material to the nerve root without mechanical deformation of the root; results show that this is sufficient to elicit pathophysiologic events which are likely closely related to the clinical complaint of sciatica. These findings have led investigators to attempt to determine the chemical basis of this pathologic response; a better understanding of this signaling may boost the effectiveness of targeted pharmaceutical therapy. Another line of empirical evidence also leads to the conclusion that mechanical deformation of the root is not alone in its role in sciatica: purely physical deformation of nerves elsewhere in the body caused by spinal cord tumors, nerve root sheath tumors, and compressive neuropathies typically present predominantly with numbness and weakness without significant pain.27 Why, then, would a herniated nucleus pulposus (HNP), if predominantly a lesion inducing mechanical deformation of the nerve root, almost always present first with pain as the chief complaint? Fig. 5.2 Sources of somatic afferent innervation of the vertebral disc and the subsequent pain signaling pathway. Different levels of information are available to investigators interested in the pathophysiology of sciatica. Animal studies, in which an experimental lesion is induced and followed by histologic assessments of proximal afferent structures, provide some information about structural changes that could be representative of inflammation or injury. Neurophysiologic studies of lesioned animals demonstrate, with more physiologic emphasis than anatomic studies, the effect of the lesion on structures in the signaling pathway. Animal behavioral studies are perhaps more compelling as these sorts of studies assess the effect of the lesion on the animal’s overall behavior pattern and more closely model the clinical complaint of pain. Human experimental studies under local anesthesia can provide information regarding the pain sensitivity of particular anatomic structures, and histologic analysis of surgical specimens allows for an understanding of receptor presence and density in the tissue of origin. Finally, therapeutic trials allow for the assessment of the effect of a treatment strategy and represent the culmination of a long line of research into basic mechanisms. A series of articles by Olmarker et al28–30 examined the effect of NP applied directly onto porcine spinal nerves without mechanical compression. On assessment of nerve conduction velocities of the NP-treated roots compared with the control roots, the NP-treated roots were noted to exhibit a pronounced decrease in nerve conduction velocities at 1, 3, and 7 days after treatment. These roots were then examined via light microscopy, and morphologic changes consistent with nerve fiber injury were noted in some of the NP-treated roots. A majority of the nerve fibers, however, exhibited no structural changes at the light microscopic level; thus, the changes noted in some fibers could not completely explain the changes in function seen in all. Electron microscopy showed significant ultrastructural changes in the NP group, even in the myelinated fibers that did not show signs of axonal damage on light microscopy. Significant findings included an expanded Schwann cell cytoplasm and intracellular edema in the treated groups, which were thought to be related to the dramatic neurophysiologic changes in these groups. These findings were confirmed in a dog model by Kayama et al.31 At 7 days from exposure to NP, nerve conduction velocities were 13 ± 14 m/second in the experimental group, and 73 ± 5 m/second in the control group. Similar to findings in the Olmarker et al study,29 structural changes of the axons consistent with nerve fiber injury were noted, but the investigators felt that these were not dramatic enough to account for the observed neurophysiologic changes. Capillary stasis was also noted in the experimental group. NP has also been found to stimulate an autoimmune-like response: subcutaneous NP has been found in animal models to attract activated T and B cells.32 Olmarker et al27 reported a new method of studying pain in the rat that focused on changes in behavior rather than changes in neurophysiologic thresholds. In this study, 40 rats were divided into four groups: exposure of L4 DRG, incision of L4/5 disc (causing leakage of NP onto the root), exposure and displacement of L4 DRG with an implanted needle, and combination of incision and displacement. These animals were assessed at 1, 3, 7, 14, and 21 days after operation, and significant behavioral differences were only noted in the series with both incision and displacement. These rats demonstrated behavior consistent with increased focal pain (lifting of the hind paw on the operated side and increased rotation of the head toward the operated side). No detectable differences were noted at 14 days; however, by 21 days after surgery, increased immobility and decreased locomotion were noted in this group. The investigators were not certain if this represented an additive effect of the two factors or if both were necessary for the condition to develop. An assessment of walking ability in rats—subjected to either laminectomy or laminectomy and exposure to NP via incision of the lumbar disc—revealed decreased walking ability in the disc incision group.33 In a unique report, Kuslich et al34 described their findings in 193 consecutive patients treated for lumbar disc herniation with a microdiscectomy under local anesthetic. During the procedure, mechanical force was applied to various tissues in and around the exposed disc, and the patient’s response to stimulus was recorded. The noteworthy findings showed that, whereas retraction of the affected nerve root caused pain in 99% of patients, retraction of an uncompressed or normal nerve produced significant pain in only 9% of cases. In concordance with the findings in the Olmarker et al28 animal study, the Kuslich et al34 findings suggest that in the clinical situation, an inflamed or stimulated root is necessary for mechanical stress to cause significant pain. To determine the component of the NP responsible for the neurophysiologic changes noted in the root subsequent to its application, Olmarker et al29 subjected porcine cauda equina to either fresh NP, NP kept at 37 degrees for 24 hours, NP kept at —20°C for 24 hours, or NP digested by hyaluronidase for 24 hours. Only the group that was subjected to lysis of the NP cells by freezing of the NP showed no reduction in conduction velocity. The researchers interpreted this to mean that the effect induced by NP was related to cell population, not the matrix, which should have been digested in the hyaluronidase group. In a follow-up study, this group demonstrated that live and dead NP cells induced similar neurophysiologic changes; the authors concluded that the effects were related to membrane-bound substances or structures.35 In a dog model of NP-induced nerve root injury investigating a potential vascular correlate of nerve conduction velocity changes, Otani et al36 demonstrated a reduction in blood flow in the nerve root that began 1 day after disc incision and was maximal after 1 week, resolving by 1 month. Nerve conduction velocity was also reduced in the experimental group, but this did not begin until 3 days after incision and was not fully resolved until 2 months later. The authors hypothesized that the reduction in blood flow represents part of the mechanism of the NP-induced neurophysiologic changes. To further investigate the mechanism of action of the NP-induced neurophysiologic effect on the nerve root, Olmarker and Larsson used a monoclonal antibody to determine whether tumor necrosis factor-a (TNF-α), an inflammatory cytokine, was present in porcine NP cells.30 The authors found that TNF-α was present in the soma of NP cells. Application of NP to the root elicited the predicted decrease in nerve conduction velocity, but treatment with doxycycline (known to block TNF-α, as well as other cytokines such as interleukin-1 [IL-1], interferon-τ, and nitric oxide synthetase) completely blocked this effect, and local application of anti-TNF-α antibody induced a partial block of the effect. Direct evidence that TNF-α is a significant element in this pathway has been reported by multiple investigators.37–39 Direct application of TNF-α to rat nerve roots produces the characteristic neurophysiologic changes caused by NP application.38 The exposure of rat L5 nerve roots to TNF-α has also been reported to result in a significant increase in the frequency of spontaneous discharges and enhanced responses of wide dynamic range neurons to noxious stimulation, as well as inflammatory changes in the ganglion.39 NP application to the nerve root has also been associated with an increase in brain-derived nerve growth factor (BDGF), a neuromodulator of nociceptive information in the dorsal horn, which is thought to play an important role in inflammatory pain states through its effect on NMDA receptor activity. BDGF has been found to be upregulated in both the DRG and the spinal cord after application of NP to the root; a follow-up study demonstrated that this upregulation could be blocked by the administration of infliximab (a monoclonal antibody to TNF-α).40 Additional studies have demonstrated that the NP-induced effects may be blocked by methylprednisolone and cyclosporine A, and somewhat less efficiently by indomethacin and lidocaine.30 Murata and Olmarker et al41 investigated the effect of intraperitoneal injection of TNF-α inhibitor (infliximab) either 1 day before or 3 hours after an induced disc herniation on histologic features of the root in a rat model. No staining for TNF-α was noted in rat NP cells 1 to 3 days after the injection, and weak staining was noted 7 days after injection. Positive staining was noted in all rats that were not injected. A characteristic semilunar enlargement of the DRG (an “inflammatory crescent”) at the site of application of the NP was noted. This crescent was noted in all of the treatment groups and was particularly pronounced at 1 and 3 days after surgery. The groups treated with the inhibitor demonstrated a smaller number of deformed nuclei at the 1-, 3-, 7-, and 14-day time points; at 21 days after surgery, the crescent was thicker in the nontreatment group. There has also been interest in the potential therapeutic effect of serotonin (5-HT) receptor antagonists due to the discovery of 5-HT receptors in the dorsal root ganglia, and the role of 5-HT in the hyperalgesia of acute inflammation demonstrated in animal models.42,43 The effect of a 5-HT 2A receptor antagonist, sarpogrelate, was examined in a rat NP root exposure model. When administered daily between days 7 and 14 after exposure, measured mechanical allodynia was significantly reduced on days 11 and 15. Two open-label studies assessing the results of treatment of patients with sciatica caused by lumbar disc herniation reported impressive results, with dramatic early relief of sciatica.44 In the first study, 10 patients with HNP underwent infusion of 3 mg/kg infliximab over 2 hours; leg pain was found to decrease by 50% at 1 hour, 60% of patients were pain-free at 2 weeks, and 90% were pain-free at 3 months.45 The control group was a historical group with sciatica caused by HNP that had been treated with periradicular injection of normal saline. A second study reported open-label use of three subcutaneous injections of a TNF-α inhibitor, etanercept (25 mg every 3 days), in 10 patients with sciatica caused by HNP.46 Again, a historical cohort was used as a control; this group had been treated with standard care and intravenous (IV) methylprednisolone. All 10 patients in the treatment group improved by day 10 as measured by the Visual Analogue Score for Leg Pain (VASL), the Oswestry Disability Index (ODI), and the Roland-Morris Disability Questionnaire (RMDQ); and 9 out of 10 continued to show improvement at 5 weeks. These improvements were significantly better than those observed in the methylprednisolone group. The findings in these open-label studies prompted a prospective, randomized clinical trial reported by Korhonen et al.47 In this study, 40 subjects with sciatica caused by HNP were randomized to treatment with either placebo or a single injection of infliximab (monoclonal antibody against TNF-α; 5 mg/kg). No difference in leg pain, back pain, health-related quality of life scales, or rate of surgery was noted in the two groups at either 3 months or 1 year after treatment. A subset of 21 of these 40 patients was analyzed by magnetic resonance imaging (MRI) both before and at 2, 4, 12, and 26 weeks after the administration of either infliximab or placebo. In both groups, HNP volume diminished at 6 months, but no difference in volume change was detected between the groups. The authors noted a slight decrease in the amount of disc herniation resorption at 2 weeks from the time of infusion in the infliximab group, but no difference was observed at the other time points; therefore, it was concluded that infliximab did not inhibit overall hernia resorption. Use of a different TNF-α antagonist, REN-1654, that belongs to a chemical class of compounds known as benzamide and has been shown to act as a functional TNF-α antagonist in vitro and in vivo, was reported by Carragee et al.48 Administration of this oral compound at 100 mg per day for 3 weeks in a placebo-controlled, prospective randomized study of 64 patients did not demonstrate a significant benefit in the primary endpoint of the study (decrease in daily leg pain at 3 weeks). However, a slightly greater reduction in maximal leg pain was observed in the treatment group in the first 2 weeks. The animal data suggesting a beneficial effect of a 5-HT2A receptor antagonist led to an NSAID-controlled, randomized trial in 40 patients with sciatica due to disc herniation.49 The authors of this study found that within the limits set by the power of their study, the efficacy of the 5HTA receptor antagonist was similar to that of NSAID therapy. Simple mechanical deformation of the nerve root is likely not sufficient to explain the clinical syndrome of sciatica related to disc herniation. Sensitization of the root, which may be related to exposure to inflammatory cytokines, seems to be necessary to induce in the root the property of signaling pain in response to the mechanical deformation caused by a disc herniation. It is likely that inflammatory cytokines such as TNF-α have a role in this process, as selective inhibitors can block the typical neurophysiologic effects elicited by NP in different animal models. It remains to be seen, however, whether this strategy of desensitization of the root via treatment with inflammatory inhibitors is useful in the treatment of radicular pain caused by disc herniation. Thus far, the few trials of this approach have not been successful in demonstrating a clinically significant effect. The mechanism of the second type of pain associated with the IVD, discogenic pain, is not well understood. The clinical and radiographic criteria by which the diagnosis of discogenic pain is established are disputed, and outcomes of operative treatment are not as successful as those for radicular pain. The uncertainty of diagnosis makes any investigation into the pathophysiologic mechanisms more challenging: without a firm understanding of what features are sufficient to cause the clinical problem, the appropriate pathophysiology to be investigated cannot be universally accepted. The goal here is to discuss mechanisms of pain generation at the level of the disc and not address other potential causes of low back pain; it will be assumed that axial back pain that arises from the disc is a significant clinical problem. Two processes may be critical to explaining discogenic pain: (1) sensitization of the nociceptive afferents, which renders them susceptible to firing in response to applied loads; and (2) degenerative changes of the disc subjected to these loading conditions. Sensitization of the nociceptive afferents, which innervate the posterior anulus and vertebral endplate, may occur in the same manner as the nerve root is sensitized by exposure to the proinflammatory substances found in the NP. The effect may be different, however, as these nociceptive afferents are clearly different structures than the nerve root or dorsal root ganglion that is stimulated and compressed by an HNP. Similar to the animal pain behavioral models of HNP that require an inflammatory stimulus and mechanical deformation of the root,50,51 axial pain arising from the disc may require both an inflammatory stimulus and mechanical loading of a degenerated disc. Degenerated discs on imaging studies in asymptomatic subjects become increasingly more common as the subject population ages; these findings of disc degeneration are not distinguishable from those found in subjects with significant pain.52 Common morphologic changes noted on imaging studies, then, are not able to differentiate painful from nonpainful disc degeneration. Many attempts have been made to identify a feature unique to painful degenerate discs compared with nonpainful degenerate discs, but there has been as yet no finding proven to have these characteristics. An understanding of the mechanical component of the syndrome of discogenic pain relies on an analysis of the biomechanics and anatomy of the degenerative disc. In an effort to describe the kinematic and mechanical correlates of disc degeneration, Kirkaldy-Willis described three biomechanical stages of disc degeneration: temporary dysfunction, unstable phase, and stabilization phase.53 The earlier phases of disc degeneration, in which the height of the disc is relatively preserved, but the nucleus has become dehydrated and the anulus has begun to degenerate, have been modeled experimentally. Significant findings of these cadaveric loading experiments are notable for increased segmental motion as a result of standard applied bending moments. In this early phase of disc instability, the flexion/extension resistance of the degenerate disc is reduced relative to its adjacent normal counterparts. Under such circumstances, an applied load and flexion moment to the spinal column (holding weight out in front of the body) will result in earlier and greater flexion at the degenerate disc relative to its counterparts with stiffer kinematics (Fig. 5.3). This situation is no longer the case in the stabilization phase, in which segmental stiffness is increased. Discogenic pain is a fundamentally painful process: for it to exist, there must be a mechanism of transmission of a nociceptive signal from the disc to proximal portions of the nervous system. In vivo evidence for significant innervation of both the posterior anulus and the vertebral endplate was reported by Kuslich et al.4 In this report, cited previously with regard to radicular pain, the anulus and vertebral endplate were stimulated during microdiscectomy under local anesthetic. It was found that stimulation of the anulus was noted to be painful in 73% of cases, and stimulation of the endplate was painful in 61% of cases. It has further been observed that the administration of small doses of short-acting local anesthetic, likely to stay confined to the intradiscal space, can quickly and dramatically improve pain thought to be discogenic in origin.54 This observation implies the presence of nociceptors accessible to the locally applied anesthetic, which are in the endplate and anulus and visualized with contrast on discography.
Innervation
Proximal Signaling Pathway
Radicular Pain
Effect of Nucleus Pulposus
Effect of Inflammatory Cytokines
Clinical Studies of Sciatica and Selective Antiinflammatories
Discogenic Pain
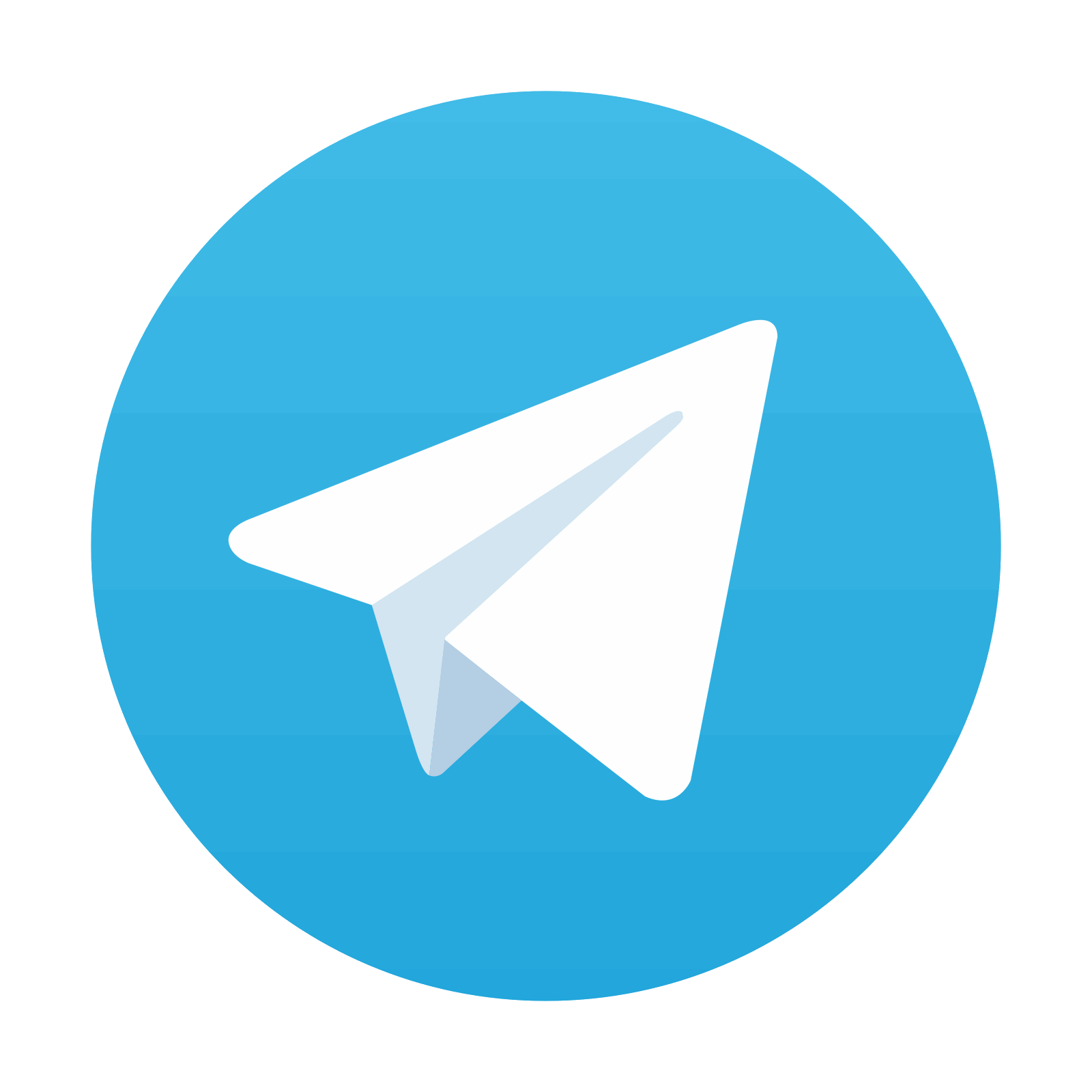
Stay updated, free articles. Join our Telegram channel

Full access? Get Clinical Tree
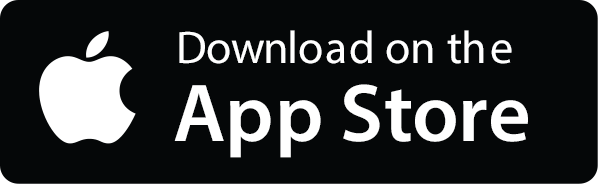
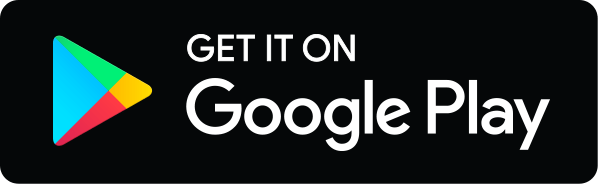