© Springer Science+Business Media Dordrecht 2015
Manuel F. Casanova and Ioan Opris (eds.)Recent Advances on the Modular Organization of the Cortex10.1007/978-94-017-9900-3_1313. The Minicolumnopathy of Autism
(1)
Department of Psychiatry, University of Louisville, Louisville, KY, USA
Abstract
The organization of the cerebral cortex is centered around a modular construct. The smallest module capable of information processing is the minicolumn. Recent studies on minicolumnar morphometry using either pyramidal cell arrays or the gray level index (GLI) suggest distinct abnormalities of this structure in several psychiatric conditions including schizophrenia, dyslexia, and autism. More specifically, minicolumns in autism as compared to controls seem thinner and more numerous. An increased number of minicolumns indicates the supernumerary division of periventricular germinal cells. A reduction in size of pyramidal cell somas within affected minicolumns suggests a bias towards shorter corticocortical connectivity. Compartmentalization of the minicolumn indicates that the majority of the deficit is found within the peripheral neuropil space. This compartment includes, among other things, many of the inhibitory elements of the cerebral cortex and provides the so-called “shower curtain of inhibition” to the minicolumn. Laminae studies indicate that in autism the peripheral neuropil defect extends the width of the cerebral cortex. A possible explanation to the above described minicolumnar abnormalities is the heterochronic division of germinal cells. Neuroblasts generated from heterochronic divisions of germinal cells can give rise to heterotopias and dysplastic cortical lesions. Once the radially migrating neuroblasts (future pyramidal cells) reach the cortex they develop asynchronously from tangentially dividing neuronal elements (interneurons). The resultant excitatory/inhibitory imbalance may provide for seizures and sensorimotor abnormalities
Keywords
MinicolumnsCerebral cortexAutism spectrum disordersInterneuronsPyramidal cellsThe known neuropathology of the different entities comprising syndromic autism strongly suggest an onset during brain development. The percentage of individuals showing an autism phenotype in many of these conditions is exceedingly high. Different series suggest that 25–50 % of all patients with tuberous sclerosis meet criteria for an autism spectrum disorder (ASD). According to some series the frequency is even larger for the Lujan Fryns syndrome (mental retardation, X-linked, Marfanoid body habitus) and the Smith-Lemli-Opitz syndrome (mental retardation and an inborn error of cholesterol synthesis). The commonality among many syndromic cases appears to be a migratory abnormality where neuroblasts fated to form part of either cortex or brainstem either fail to reach their normal target areas or reach the same and are desynchronized in their development from other cellular elements already in place. Syndromic cases with migratory abnormalities exhibit subependymal nodular heterotopias, cluster of neuronal cells within the white matter, and a variety of cortical malformations. Although the above mentioned cases are the result of genetic conditions (including de novo mutations), exogenous factors such as viral infections (e.g., cytomegalovirus) and even cocaine during gestation may alter cellular divisions of the germinal matrix thus providing for similarities in both neuropathology and clinical presentations.
Abnormalities of neuronal migration are intimately related to malformations of the cerebral cortex. Malformations during brain development, also called dysplastic changes, are usually manifested as cortical thickening or thinning, blurring of the gray-whiter matter junction and a variety of histological findings, e.g. effacement of laminar patters, minicolumnar morphometric abnormalities, malpositioned neurons (supernumerary neurons in the molecular layer and white matter). In this regard the pathology observed in the cerebral cortex is secondary to processes impinging on its formation, e.g. germinal cell divisions, migration of neuroblasts to the cortical plate. All of the previously observed dysplastic changes have been reported in both syndromic and idiopathic autism (Casanova et al. 2013). The significance of the findings lays in their capacity to explain some of the phenomenology in autism spectrum disorders, e.g., medically refractory seizures and sensory abnormalities.
This chapter will focus on certain aspects of cortical dysplasia in ASD as revealed by minicolumnar morphometry. Vertical aggregates of cells forming closed circuits were first described anatomically by Lorente de Nó (1938): “All the elements of the cortex are represented in it, and therefore it may be called an elementary unit, in which, theoretically, the whole process of transmission of impulses from the afferent fiber to the efferent axon may be accomplished”. According to Mountcastle, the minicolumn is the smallest element of information processing within the cerebral cortex (Mountcastle 1998). Recent electrophysiological studies reveal that sensory processing at the level of the minicolumn modulates the expression of higher cognitive functions (Opris et al. 2013). Differences in minicolumnar morphometry between hemispheres have been used to explain cerebral dominance for the language regions of the brain (Buxhoeveden et al. 2001). Since this difference appears to be specific to our species (not present in non-human primates), it may be a putative speciation event relating language to cytoarchitectonic features of the brain (Buxhoeveden et al. 2001). Defects in minicolumnar morphometry may therefore be present in psychiatric conditions such as ASD which are defined by language abnormalities.
13.1 Minicolumnar Morphometry in Autism Spectrum Disorders
The first study on minicolumnar morphometry in autism spectrum disorders sampled nine cases and an equal number of age-matched controls (Casanova et al. 2002a, b, c). All of the specimens were collected and processed by Drs. Thomas Kemper and Margaret Bauman. There were no abnormalities for any of the cases at gross examination. Four of the nine autistic cases were macrocephalic with brain weights more than two standard deviations above the means for their corresponding ages. The medical records detailed little information as to whether the abnormal brain weights were due to postmortem swelling, an artifact, or a real phenomenon. These cases had been cut midsagittally, freezing one hemisphere and leaving the other for microscopic examination. In order to avoid shrinkage artifacts, brains were embedded in celloidin. Sections were cut at 35 μm and Nissl stained. Every 20th section was collected and for each 100th section and adjacent slide was stained for myelin using the Loyez method. Previous reports on the pathology of these cases used a Zeiss comparison microscope and offered subjective assessments of findings when viewing simultaneously side-by-side anatomically matched slides. In this patient population, Bauman and Kemper indicated the presence of reduced neuronal size and increased cell density in the amygdala, hippocampal complex, subiculum, entorhinal cortex, medial septal nuclei, and mammillary bodies (Bauman and Kemper 2005).
Bauman and Kemper reported few cortical findings in this series. Most prominent among these were an indistinct pattern of lamination in the anterior cingulate gyrus and a minor malformation in one case of the orbitofrontal cortex (Bauman and Kemper 1987; Kemper and Bauman 1998). Subjective appraisal of the slides (whole hemisphere coronal sections) made it difficult to draw firm conclusions about the cerebral cortex. It is in this regard that we attempted relative quantitation by using computerized image analysis and implementing an algorithm based on the Euclidian minimum spanning tree. Images from lamina III in three cortical regions (Brodmann areas 9, 21, and 22) were digitized and cells reduced to points using lines such that the total length of all lines was minimized. Minicolumns were identified as vertical clusters of large neurons delimited on either side by cell-sparse areas. Imaginary lines through the sparse areas partitioned the field into polygonal regions thus defining minicolumnar segments. Descriptive statistic of minicolumnar morphometry included columnar width (CW), peripheral neuropil space (NS), interneuronal distance (MCS), and compactness (RDR). Results from this study revealed that minicolumns in ASD were narrower with a mean CW of 46.8 vs. 52.8 μm in the control brain. Both the peripheral neuropil space and compactness were reduced in autism.
Results from this first study revealed that minicolumns in ASD are of reduced width with a significant portion of the abnormality accounted by a diminution of the peripheral neuropil space. The lateral compartment of the minicolumns, the peripheral neuropil space, is the conduit, among other things, for inhibitory circuit projections. Previous researchers have called this compartment of the minicolumns: “a shower curtain of inhibition” or a “strong vertically directed stream of inhibition” (Szentágothai 1978; Mountcastle 1997). This inhibitory compartment sharpens the functional borders of the minicolumn and increases their definition or discreteness (DeFelipe 1999; Favorov and Kelly 1994a, b; Szentágothai 1978). The primary source of this inhibitory effect stems from the action of double-bouquet and basket cells. Double-bouquet cell axons arrange themselves in repeating vertical patterns between 15 and 30 μm apart depending on cortical area examined (DeFelipe 1999). It is thought that the vertical descent of double bouquet cells across laminae acts as a buffer by inhibiting dendritic terminals belonging to excitatory (pyramidal) cells of neighboring minicolumns. A defect in these inhibitory elements could cause the signals being processed in the core of the minicolumn to suffuse into adjacent minicolumns. The resultant avalanche of activity could help explain the significant prevalence of seizures in ASD.
An interesting possibility worth considering is what would happen in ASD if thalamic fields retain the same area dimension while minicolumns are smaller? The result for ASD would be an increased number of minicolumns innervated per thalamic afferent than in the normal brain. Alternatively, the failure to assimilate additional minicolumns into a thalamic field would impel these processing units to establish connections with functionally dissimilar sets of thalamic neurons (Favorov and Kelly 1994a, 1994b). In the later instance, the failure of the system to assimilate the additional minicolumns would result in cortical noise; that is, supernumerary units of activity that overtax the system (Casanova et al. 2002a, b, c).
Individual cells when depolarized cannot produce gamma activity. This fast oscillation, in the gamma range, is responsible for creating the unity of conscious perception. Only thalamic modulation of neuronal networks can provide for intracortical gamma activity (Sukov and Barth 2001; Macdonald et al. 1998). Because interneurons can help synchronize neuronal discharges and some exhibit fast spiking activity, they are important mediators of gamma activity. Lack of integration among separate specialized local networks (a binding failure) may account for a weak central coherence in autism spectrum disorders. Several laboratories have recently reported a disorder of binding related gamma EEG oscillatory activity in autism spectrum disorders (Grice et al. 2001; Sokhadze et al. 2009; Baruth et al. 2010).
The same patients and regions of interests reported by Casanova et al. (2002a) were examined for descriptive parameters of the Gray Level Index (Casanova et al. 2002b; Schlaug et al. 1995). According to this method a fractional area of Nissl-stained objects was computed (profiles of 11 pixels 110 um wide). Images were smoothed with the resultant profiles leading to the analysis of its peaks and troughs. The method produced measurements of peak width distance and a verticality index. The verticality index quantified the degree of columnar organization relative to the mean of the entire sample. The overall statistical test revealed significant differences between autistic patients and controls and between hemispheres. There was no significant age dependency between factors. Follow-up univariate tests showed significant diagnosis-dependent effects in feature distance. No significant differences were evident in overall verticality.
Results from the latter two studies using different algorithms indicate the presence of vertical cellular structures in the cerebral cortex of individuals with autism spectrum disorders that are packed more closely together and more regularly spaced than in controls (Casanova et al. 2002a, b). The results equally applied to all areas examined (Brodmann areas 9, 21, 22) in both hemispheres. The findings suggest a developmental defect of the nervous system.
A third study on minicolumnar morphometry in ASD was carried in an independent sample as an international effort sponsored by the Autism Tissue Program (Casanova et al. 2006a, b). Different laboratories were charged with collecting samples, constructing photomicrograph montages, and performing the computerized image analysis. The results were made available to all of the participating researchers before the codes were broken and the data analyzed statistically. The patient population consisted of six age-matched pairs of ASD subjects (DSM-IV-TR and ADI-R diagnosed). Tissue specimens consisted of full coronal sections embedded in celloidin and cut at 200 μm. Gallocyanin-stained sections were used to identify cortical areas M1, V1, frontal association cortex, and S1 (Brodmann areas 4, 17, 9, and 3b). Sections were delineated with a stereology workstation and photographed with a digital camera at 40X. The Virtual Slice module of the StereoInvestigator was used to assemble digitized photomicrographs into one mosaic. Computerized image analysis of minicolumnar morphometry in Laminae II through VI was performed with algorithms previously described in the literature (Casanova and Switala 2005). A threshold function eliminated the smaller cellular elements (interneurons) and allowed the researchers to concentrate on measurements provided by pyramidal cells. Minicolumnar width was reduced in autism spectrum disorder individuals as compared to controls. Mean neuron (soma) and nucleolar size was reduced in ASD, while neuron density (based on a point process model) in autism exceeded the control group by 23 %.
The findings reported in the international study on minicolumnar morphometry in ASD reproduced previous findings (Casanova et al. 2006a). In this study the feature extraction properties of the program were corrected for minicolumnar fragments, curvature of the tissue section, and were adapted to 3D proportions (stereological modeling). The smaller minicolumns per given brain region translates into increased numbers when corrected for 3D quantitation. The smaller minicolumns and their overall increase in total numbers translated into an increased neuronal density. However, the basis for the increase cellular density remained conjectural, e.g., the presence of smaller supernumerary minicolumns, an increase in the total number of cells per minicolumn, or both. A subsequent analysis based on a Delaunay triangulation addressed the aforementioned concerns.
The Delaunay triangulation parcellates points by joining them with edges forming triangles. The edges satisfy the criteria of an “empty circle” property where for each edge we can find a circle containing the edges’ endpoints but no other points within the same. Owing to the clustering of cells within vertical arrangements, the Delaunay triangulation demonstrates a bimodal distribution of edges between cells in the same minicolumn (intracluster) and edges between cells in neighboring minicolumns (interclusters). The Delaunay triangulation indicated a significant reduction in the edges of intercluster distances but not within intracluster differences. Increased neuronal density in this series was due to the presence of supernumerary minicolumns, otherwise the total number of neurons (pyramidal cells) per minicolumn was normal (Casanova et al. 2006a).
Minicolumns in autism, although smaller in size, are increased in total numbers as compared to neurotypicals. Furthermore within each minicolumn a reduction of both neuronal soma and nucleolar size biases connectivity towards shorter connections. Casanova et al. (2006a) concluded that, “Just et al. (2004) have subsumed the evidence for a lower degree of information integration in autism under the rubric of the “underconnectivity theory”. However, the term may prove to be a misnomer when applied to shorter intra-areal connections (arcuate or u fibers). In autism, an increase in the total number of minicolumns requires a scale increase (roughly a 3/2 power law) in white matter to maintain modular interconnectivity (Hofman 1985). This additional white matter takes the form of short-range connections which makes up the bulk of intracortical connections.” Dysfunction of long projections translates into complex abnormalities within widely distribute networks. Some authors have suggested that a “dysexecutive syndrome” in autism could possibly result from the frontal lobe’s complex pattern of connectivity. The widely distributed network of connectivity of the frontal lobes accounts for the phenomenon of diaschisis where executive cognitive deficits may become apparent in lesions distant to the anterior cortical region (Mesulam 2002; Casanova et al. 2006b).
The international group that produced the Casanova et al. (2006a) study also expanded on previous findings by examining the topographical distribution of the autistic minicolumnopathy (Casanova et al. 2006b). Tissue embedding and processing was identical as before but the number of sampled regions of interest increased to include Brodmann areas: 10 (frontopolar), 11 (orbitofrontal), 9 (dorsolateral prefrontal), 4 (primary motor), 3b (primary sensory), 43 (frontoinsular), 44 (ventrolateral), 24 (anterior cingulate), and 17 (primary visual). The sampling included areas of the cortex representing paralimbic, heteromodal association, unimodal association, and primary areas. The study found an interaction of diagnosis and region for peripheral neuropil space. Post hoc analysis revealed significant differences for the frontopolar region (area 10) and the anterior cingulate gyrus (area 24). The role of the frontopolar cortex in executive functions and of the anterior cingulate gyrus in the analysis of socially salient information suggests that involvement of these areas may provide a correlate to some of the more salient clinical manifestations of autism.
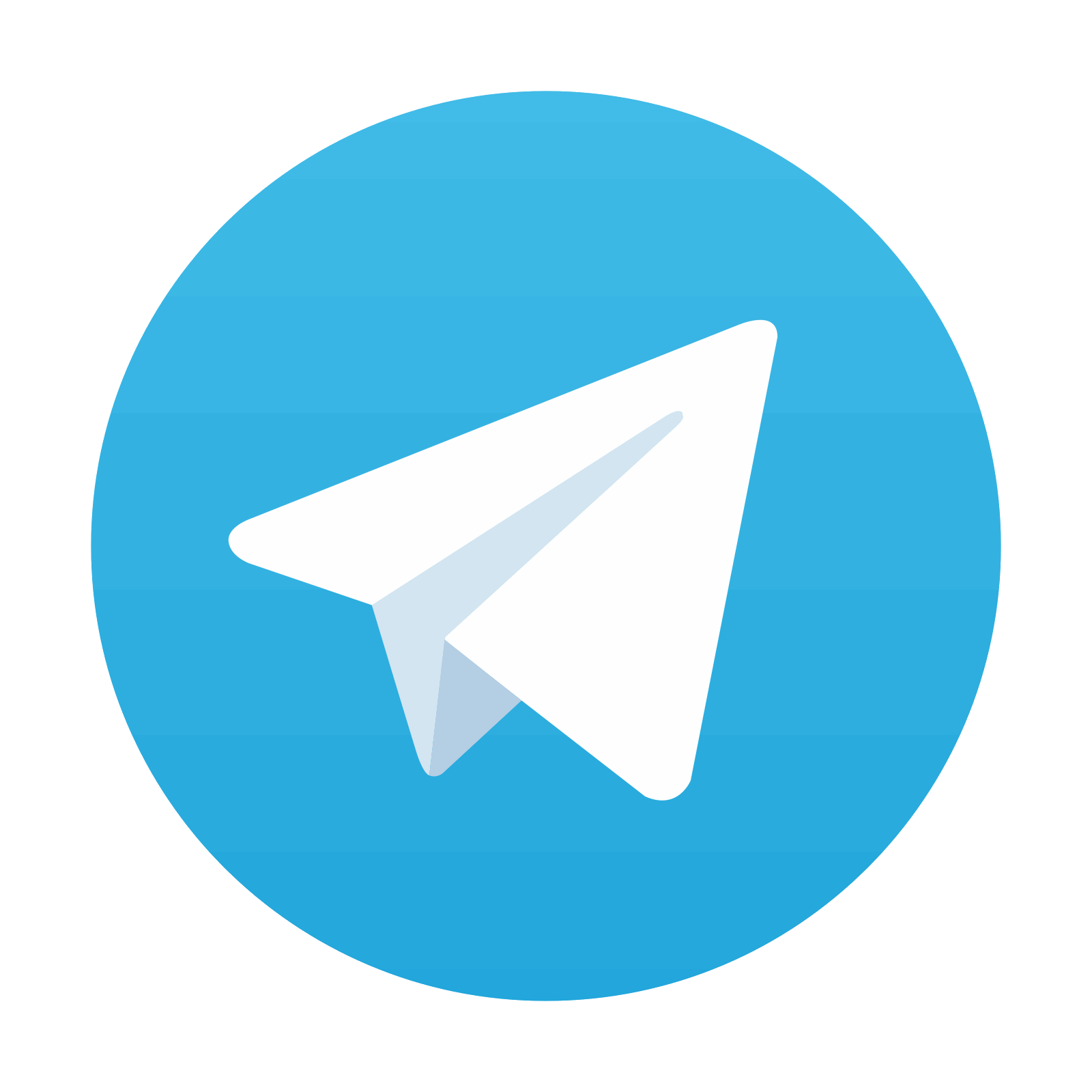
Stay updated, free articles. Join our Telegram channel

Full access? Get Clinical Tree
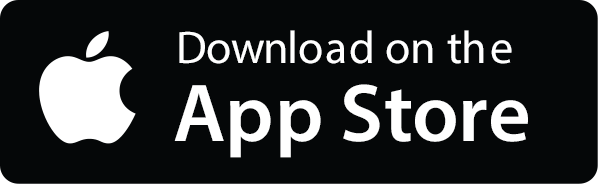
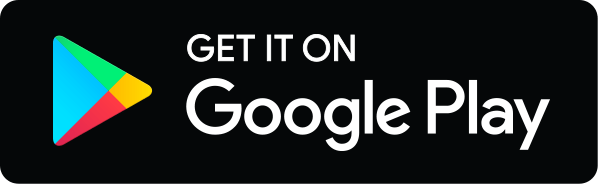