Depression is a highly prevalent and disabling mental illness characterized primarily by abnormalities of mood, cognition and behavior. Individuals with major depression frequently experience anhedonia, depressed mood, negative self-referential thinking, impaired concentration, suicidal ideation, abnormal psychomotor activity, and variable changes in appetite and sleep. Major depression is among the most studied idiopathic psychiatric conditions, with an increasingly well-understood neurobiology. These insights are a pre-requisite to the development of more targeted therapeutic strategies, and can be helpful in understanding this disorder in the context of medical illness.
In this chapter, an overview of the neurocircuits frequently implicated in mood disorders is first detailed to allow for a systems-level conceptualization of brain-behavior relationships in depression.1 This is followed by a discussion of in vivo human structural, neurochemical and functional neuroimaging findings and postmortem brain abnormalities in depressed subjects. Neuroendocrine, inflammatory, neurotransmitter, cellular–molecular and genetic–epigenetic disturbances associated with depression are also summarized to provide a comprehensive multi-level understanding of this illness. The frequently encountered clinical and neurobiological intersection of depression and anxiety is discussed in Box 2-1. It should also be noted that the neural circuits discussed in this chapter are the same distributed brain regions frequently implicated in mood related symptoms as a consequence of medical and neurological conditions discussed elsewhere in this book. Throughout the chapter, the term “depression” will be used synonymously with major depressive disorder, unless otherwise specified, though it is relevant to highlight that a dimensional, symptom-oriented approach is increasingly being taken across traditional diagnostic boundaries.
BOX 2-1 THE INTERSECTION OF DEPRESSION AND ANXIETY
Depression is frequently comorbid with anxiety which includes generalized anxiety disorder, social phobia, posttraumatic stress disorder, and panic disorder among other disorders.185,186 From a dimensional perspective, this overlap may be referred to as an anxious depression. High trait anxiety and neuroticism have been shown to be risk factors for the development of depression,187 and depressed individuals show enhanced fear learning.188 From a systems-level perspective, neuroimaging research studies have started investigating brain–behavior relationships in individuals with anxious depression compared to nonanxious depressed individuals. For example, during an emotional conflict identification task that involved categorizing emotionally valenced faces while ignoring overlaid emotional words, depressed patients with and without comorbid generalized anxiety disorder similarly demonstrated impaired ventral anterior cingulate and amygdala activations and connectivity on functional magnetic resonance imaging (fMRI); depressed only individuals, however, showed additional recruitment of lateral prefrontal regions that positively associated with task performance.189 Resting-state functional connectivity analyses in a cohort of individuals with late-life anxious depression compared to nonanxious depressed subjects showed increased coupling of posterior Default Mode Network (DMN) regions and decreased coupling of anterior DMN in anxious depressed subjects.190 Structural analyses in a large group of patients with nonanxious depression, mixed anxiety disorders, and anxious depression demonstrated similar rostral anterior cingulate cortex gray matter volume reductions across all patient groups compared to healthy subjects.191 Electrophysiology studies including electroencephalography (EEG) have also been used to study brain differences in depressed individuals with and without anxiety192; for example, a quantitative EEG study showed right-lateralized hemispheric activation differences in patients with anxious depression compared to depressed subjects.193 From a neuroendocrine perspective, subjects with anxious depression exhibited attenuated adrenocorticotropic hormone (ACTH) and cortisol secretion following CRH challenge compared to mixed depressed-bipolar disorder and healthy subject cohorts.194 Anxious depressed individuals have also demonstrated impaired cortisol suppression following dexamethasone challenge.195 Early-stage genetic investigations implicate some convergent and interrelated neurochemical and cellular–molecular pathways in the neurobiology of depression and anxious depression. For example, less robust antidepressant treatment response has been associated with anxious depressed individuals carrying the short allele of the serotonin-transporter–linked promotor region (5-HTTLPR)196 Genes that regulate the CRH system and BDNF have also been linked to antidepressant treatment response in anxious depressed individuals.197,198 In summary, depression and anxiety symptoms are frequently clinically interrelated and early neurobiological investigations suggest neurocircuit, cellular–molecular and genetic level relationships that warrant further research inquiry.
The human prefrontal cortex, anterior to the primary motor cortex, plays critical roles in many of the complex affective, cognitive, and behavioral functions (and dysfunctions) important in the neurobiology of mood disorders. From a structural perspective, the prefrontal cortex is organized into five distinct prefrontal–subcortical circuits. Cortically, these five distinct circuits include: (1) primary motor/premotor/supplementary motor; (2) oculomotor; (3) dorsolateral prefrontal cortex (dlPFC); (4) anterior cingulate cortex (ACC); (5) orbitofrontal cortex (OFC).2 Each of these regions has distinct, parallel connections to the caudate-putamen (striatum), which in-turn projects to the globus pallidus and specific thalamic nuclei. Afferent connections from discrete thalamic nuclei project back to the same prefrontal cortical region. While these cortical–subcortical–cortical circuits have distinct motor–cognitive–affective–behavioral functions, the subcortical components of each of these circuits are implicated in the automaticity of these distinct functions.
Of particular importance for psychiatry are the three nonmotor prefrontal–subcortical circuits originating from the ACC, OFC, and dlPFC (Fig. 2-1).3 The ACC, a paralimbic cortical structure, and its subcortical connections are implicated in motivated behavior, conflict monitoring, cognitive control, and affective regulation. Regions of the ACC located subgenually and rostral to the genu of the corpus callosum have been implicated in emotion regulation, while more dorsal regions are involved in cognitive functions and behavioral expression of emotional states.4,5 An important cognitive function of the dorsal ACC is the ability to engage in aspects of cognitive control—the ability to pursue and regulate goal-oriented behavior. ACC–subcortical connections include the nucleus accumbens/ventromedial caudate, ventral globus pallidus, and ventral aspects of the magnocellular mediodorsal and ventral anterior thalamic nuclei. Deficit syndromes linked to the ACC–subcortical circuits include the spectrum of amotivational syndromes (apathy, abulia, akinetic mutism), and cognitive impairments, including poor response inhibition, error detection and goal-directed behavior.
Figure 2-1
Graphic depiction of anterior cingulate, orbitofrontal and dorsolateral prefrontal–subcortical circuits. ACC, anterior cingulate cortex; OFC, orbitofrontal cortex; dlPFC, dorsolateral prefrontal cortex; VM, ventromedial; vDM, ventral portion of dorsomedial thalamic nucleus; vVA, ventral portion of ventral anterior thalamic nucleus; DM, dorsomedial; imDM, inferomedial portion of mediodorsal thalamic nucleus; mVA, medial portion of ventral anterior thalamic nucleus; DL, dorsolateral; LDM, lateral dorsomedial; MD, mediodorsal; VA, ventral anterior. (Adapted with permission from Perez DL, Catenaccio E, Epstein J: Confusion, hyperactive delirium, and secondary mania in right hemispheric strokes: A focused review of neuroanatomical correlates, J Neurol Neurophysiol 2011; Special Issue 1.)

The OFC–subcortical circuit is implicated in socially appropriate and empathic behavior, reward-based decision making, mental flexibility, response inhibition, and emotion regulation. Similar to the regional functional specificity described for the ACC, the OFC has functional specificity along its anterior–posterior and medial–lateral axes. The medial OFC has been primarily linked to behavioral responses in the context of viscerosomatic processing, while more lateral regions mediate more external, sensory evaluations.6 The posterior OFC is particularly important for emotion regulation. The OFC–subcortical connections include the ventromedial caudate, mediodorsal aspects of the globus pallidus interna, and the medial ventral anterior and inferomedial aspects of the magnocellular mediodorsal thalamus. OFC dysfunction, depicted in the classic personality change experienced by Phineas Gage following injury of his left medial prefrontal cortex by a metal rod in a construction accident,7 is associated with impulsivity, disinhibition, socially inappropriate behavior, and mental inflexibility.
The dlPFC–subcortical circuit is principally involved in attentional and higher-order cognitive executive functions. Executive functions include the ability to shift sets, organization, planning, cognitive control, and working memory. Shifting sets is related to mental flexibility and consists of the ability to move between different concepts or motor plans, or the ability to shift between different aspects of the same or related concept. Working memory is the on-line maintenance and manipulation of information. The dlPFC–subcortical circuit consists of the dorsolateral head of the caudate, lateral mediodorsal globus pallidus interna, and the parvocellular aspects of the mediodorsal and ventral anterior thalamic nuclei. Dysfunction in this circuit has been linked to environmental dependence syndromes (including utilization and imitation behavior), poor organization and planning, mental inflexibility, and working memory deficits.
From a general perspective, these affective, cognitive and behavioral prefrontal–subcortical connections are closed circuits; the subcortical components are thought to be nonoverlapping across the distinct circuits in the healthy brain. Interactions across the circuits are critical for integrated brain function and occur at the corticocortical level (Fig. 2-2).8 Reciprocal connections exist between the dlPFC–ACC and between the ACC–OFC. Given these connectivity patterns, the anterior aspects of the dorsal ACC are theorized as an integrative zone for cognitive-emotional processing.
Figure 2-2
Graphic depiction of frontolimbic neurocircuitry. Reciprocal connections are outlined among the perigenual anterior cingulate cortex (pACC), subgenual ACC (sgACC), orbitofrontal cortex (OFC), dorsolateral prefrontal cortex (dlPFC), insula, amygdala (A), and hypothalamus (H). Adapted with permission from Perez DL, Barsky AJ, Daffner K, et al. Motor and somatosensory conversion disorder: a functional unawareness syndrome? J Neuropsychiatry Clin Neurosci. 2012 Spring;24(2):141–151.

In addition to cortical–basal ganglia–thalamic circuits, fronto-amygdalar connections are important in the neurobiology of mood. The amygdala, an almond-shaped structure in the medial aspect of the temporal lobe anterior to the hippocampus, is a critical brain region for emotional processing and affectively driven memories, fear-based behaviors, arousal, and salience determinations.9 Bilateral amygdala lesions, as seen in the Kluver Bucy syndrome, result in loss of appropriate behavioral responses to potential threats and indiscriminate consummatory behaviors. The amygdala is composed of discrete nuclei (Fig. 2-3). The lateral and basal nuclei are the main sites receiving afferents from sensory cortices, heteromodal association cortices, the hippocampal–entorhinal cortex, and regulatory prefrontal regions. The central nucleus is the principal output nucleus and has efferent connections with the hypothalamus, periaqueductal gray and brainstem monoamine neurotransmitter systems among other regions. A major amygdala outflow tract is the bed nucleus of the stria terminalis. The efferent connectivity pattern of the amygdala enables activation of the fight-or-flight response in response to motivationally salient stimuli. Amygdala outflow is regulated, in part, by the prefrontal cortex. Medial prefrontal cortices, including the ACC and OFC, have reciprocal connections with the amygdala and are important in the inhibitory regulation of amygdalar function. Disruption of prefrontal efferents projecting to the amygdala result in unregulated amygdalar activity (impaired top-down inhibition) and subsequent heightened affective symptoms and emotional responses. The amygdala also interacts with the prefrontal–subcortical circuits through its connections with the mediodorsal nucleus of the thalamus.
Figure 2-3
A and B: Panel A: inputs into specific amygdala nuclei. Panel B: outputs from the amygdala. B, basal nucleus; Ce, central nucleus; ict, intercalated cells; La, lateral nucleus; M, medial nucleus; aud, auditory; vis, visual; somato, somatosensory; gust, gustatory; olf, olfactory; assoc, association; NE, norepinephrine, DA, dopamine, Ach, acetylcholine; 5-HT, serotonin; parasym ns, parasympathetic nervous system; symp ns, sympathetic nervous system; inst, instinctual. (Reproduced with permission from LeDoux J. The amygdala. Curr Biol. 2007;17(20):R868–R874.)

Posterior to the amygdala in the medial temporal lobe lies the hippocampus and related entorhinal cortex and parahippocampus. The hippocampus is a critical node in the cortical–hippocampal system for declarative forms of memory, including episodic (biographical) and semantic (factual) subtypes.10 Damage to this region, as seen in Alzheimer disease, results in deficits in the encoding of new information. The hippocampus, parahippocampus, and neocortical association areas comprise three critical components of this circuit. Cortical regions, including the ventromedial prefrontal cortex, anterior and posterior cingulate, dlPFC, posterior parietal and temporal cortices send afferent projections to the parahippocampus, providing cognitive, affective, motor and/or perceptual information. The parahippocampus in this capacity serves as a convergent site for cortical information, and subsequently projects to the hippocampus, where individual elements of memory are linked together across cognitive–affective–perceptual–behavioral domains. Reciprocal connections between the hippocampus—parahippocampus, and between the parahippocampus and neocortical regions allows for the cortical storage of this bound information. Additional regions in the declarative memory circuit include the fornix, mammillary bodies, and anterior nuclei of the thalamus (Fig. 2-4). As with amygdalar circuits, convergence of the prefrontal–subcortical circuits and the cortical–hippocampal system occurs at the level of the anterior thalamus. Of note, the precuneus has been implicated in retrieval of episodic memory,11 while the inferior lateral temporal lobes are more strongly associated with semantic memory. Implicit forms of memory, such as procedural memory, involve motor-related cortical–subcortical pathways and the cerebellum.
Figure 2-4
The limbic system and related deep-lying structures. A: Medial brain view showing prefrontal paralimbic cortices and medial temporal lobe structures, including the amygdala and the hippocampus. (Adapted with permission from Damasio AR, Van Hoesen GW. The limbic system and the localisation of herpes simplex encephalitis. J Neurol Neurosurg Psychiatry. 1985;48(4):297–301.) B: Interconnections of the deep-lying structures included in the limbic system. The arrows indicate the predominant direction of neural activity in each tract, although these tracts are typically bidirectional. (Adapted with permission from Nieuwenhuys R, Voogd J, van Huijzen C. The Human Central Nervous System: A Synopsis and Atlas. 4th ed. Berlin: Springer Verlag; 2008.)

In addition to classic frontolimbic regions, the insula has emerged as an important region for viscerosomatic and emotional processes.12,13 The insula sits on the inner banks of the lateral sulcus, and its posterior aspect receives viscerosomatic afferents from the thalamus. The posterior insula provides an interoceptive representation of the physiological condition of the body. The mid-insula is considered an integrative zone, in which emotional/motivational information from ACC, OFC, and amygdalar afferents influences sensory processing. The integration of visceral-somatic, affective, and motivational information converges onto the anterior insula (right > left) which, together with the ACC, has been implicated in emotional awareness.13 Interestingly, the insula and ACC share large spindle-shaped neurons, termed von Economo neurons, linked to social–emotional cognition.14
Disturbances in intra and inter network connectivity of the default mode network (DMN) have also been theorized to play a role in the pathobiology of mood disorders (Fig. 2-5).15 This widely distributed network comprises core regions that include ventral and dorsal medial prefrontal cortex, posterior cingulate cortex/precuneus, inferior parietal lobule, lateral temporal cortex, and the hippocampus. These regions co-activate when individuals are allowed to think in an unrestricted fashion, and co-deactivate when subjects engage in a broad array of cognitively demanding tasks. In addition, functional connectivity MRI analyses have revealed that low-frequency oscillations in the fMRI blood-oxygen-level-dependent (BOLD) signal are robustly correlated among these regions, further underscoring the notion that they form a coherent network (see below). DMN regions overlap with those postulated to serve a role in certain social and emotional functions in healthy individuals. For example, the dorsal medial prefrontal cortex appears to be important to self-referential processing and taking the mental perspective of others.
Figure 2-5
Default mode network (DMN). Performance of a wide variety of tasks leads to decreased activity in broadly distributed regions including dorsal medial prefrontal cortex and posterior cingulate cortex/precuneus referred to as the DMN (top row). This distributed network can be appreciated by capturing low frequency oscillations in the blood oxygen level dependent (BOLD) signal (middle row). The same regions that deactivate with task increase in activity when subjects allow their mind to freely wander and self-reflect (bottom row). (Reproduced with permission from Raichle ME. Two views of brain function. Trends Cogn Sci. 2010;14(4):180–190.)

Modern neuroimaging methodologies have allowed the in vivo examination of brain structure and function. Importantly, these techniques have shed light on important factors contributing to the range of brain disorders, including depression. Brain abnormalities in depressed patients delineated via structural and functional neuroimaging techniques are discussed below,16 preceded by brief descriptions of the relevant imaging technologies. Postmortem brain abnormalities are also reviewed to contextualize and validate the neuroimaging findings. These abnormalities are interpreted in the context of the neuroanatomy outlined above.
Structural imaging techniques employed in studies of depression include computed tomography (CT), magnetic resonance imaging (MRI), and diffusion tensor imaging (DTI). Brain CT was first made readily available in the 1970s, and this technique uses ionizing radiation to distinguish bodily tissues based on density related differences in radiation attenuation. While particularly beneficial for acute hemorrhage detection, CT scans have limited spatial resolution, and limited ability to delineate subtle soft tissue differences. MRI scans have significant advantages over CT in spatial resolution, and rely on the physical properties of hydrogen protons in the context of statically and dynamically applied magnetic fields to visualize and differentiate brain tissues (Fig. 2-6). The appearance of the brain on MRI is dependent on acquisition parameters, such as echo time (TE) and the time repetition (TR). Commonly acquired MRI sequences include T1, T2 and Fluid Attenuated Inversion Recovery (FLAIR). On T1 sequences, the white matter appears white, and gray matter structures such as the cerebral cortex and brainstem nuclei appear gray. T2 sequences display an inverse pattern of gray-white differentiation. FLAIR-based sequences use an inversion recovery pulse sequence to block cerebrospinal fluid signal and aid detection of periventricular abnormalities. FLAIR/T2 sequences are predominantly used to detect parenchymal abnormalities, while the T1 sequence provides greater anatomic visualization (and regional atrophy characterization). Quantified structural neuroimaging research in psychiatry generally utilizes high-resolution MRI scans. Manual volumetry is a technique that combines manual tracing of regions of interest (ROIs) with calculations that estimate cross-sectional volumes. Although readily available, this technique is highly operator dependent and time consuming. Voxel-based morphometry (VBM) is an automated technique that deconstructs T1-weighted images into discrete voxels of either gray or white matter. Thereafter, voxel-wise statistical parametric mapping (SPM) techniques are applied across cohorts to detect group differences.17 Automated techniques have also been developed to specifically measure cortical gray matter thickness.18,19 In addition to comparative volumetric differences, variations in the gyral morphometry may also be studied using statistical techniques.
Structural studies in depression have principally identified frontolimbic and prefrontal–subcortical circuit abnormalities (Fig. 2-7). Early MRI studies in depression using manual tracing techniques identified decreased frontal lobe volumes and increased subcortical and periventricular white matter lesions.20,21 Lesion studies in patients with post-stroke depression (a secondary depression syndrome) suggested a particular association between depressed mood and left anterior frontal lesions.22
Figure 2-7
Graphic depiction of commonly detected volumetric neuroimaging abnormalities in depression. dlPFC, dorsolateral prefrontal cortex; Ins, insula; OFC, orbitofrontal cortex; ACC, anterior cingulate cortex, d, dorsal; r, rostral; sg, subgenual; THAL, thalamus; HF, hippocampal formation. (Used with permission from David Vago PhD.)

Hippocampal volume changes have been extensively studied in depression. Compared to healthy subjects, depressed patients exhibited hippocampal volume reductions,23 and these structural changes have also been described in individuals in the midst of their first depressive episode.24,25 While not all volumetric studies have cross-sectionally identified hippocampal atrophy in patients with depression, a strong association between total time depressed and hippocampal volume reductions has been reported26; a meta-analysis examining clinical predictors of hippocampal volume loss confirmed this finding.27 In a 3-year longitudinal VBM study, chronically depressed patients exhibited progressive decline in hippocampal volume, with less hippocampal atrophy in remitted patients.28 A volumetric tracing study of hippocampal differences in nondepressed individuals with a first-degree relative with major depressive disorder compared to healthy subjects without a family history of depression, identified smaller bilateral hippocampal volumes in patients with a family history of depression and previously reported early-life adversity (abuse).29 This suggested that hippocampal atrophy may represent a neural mechanism underlying vulnerability to the development of depression.
Amygdalar abnormalities in depression may display a dynamic pattern of volumetric change. Compared to both healthy subjects and patients with recurrent depression, patients in their first depressive episode exhibited enlarged amygdala volumes in an MRI study using volumetric tracing techniques.30 Parallel amygdala volume increases and hippocampal volume decreases were described in a cohort of women with recent-onset depression.31 With progressive illness duration, a longitudinal prospective study identified progressive left amygdala gray matter density reductions.28
In addition to medial temporal lobe abnormalities, prefrontal–subcortical structural changes have been extensively characterized in depression. Volumetric reductions in the left subgenual ACC were described in young and middle-aged women with depression compared to healthy women using manualized tracing methods,32 however, this finding has not consistently been replicated.33,34 Sex differences and illness duration may potentially account for some of this heterogeneity. Depressed men compared to depressed women demonstrated smaller left subgenual ACC volumes,35 and patients with three or more episodes of untreated depression were more likely to exhibit subgenual ACC volumetric reductions compared to individuals with shorter illness durations.36 Structural changes have also been reported in other regions of the ACC including gray matter volume reductions in the rostral ACC (using VBM), and an inverse correlation between rostral ACC volume and circulating salivary cortisol levels.37 The OFC, like the ACC, exhibits volumetric reductions38 and possible sex differences39 in depression. In a large cohort of 226 depressed and 144 nondepressed elderly individuals, depressed subjects exhibited smaller OFC volumes using semi-automated tracing methods,40 and OFC cortical thickness reductions have also been characterized.41 Of note, late-life (geriatric) depression may have distinct pathophysiology mechanisms compared to idiopathic depression which are discussed below.42 Caudate-putamen components of the prefrontal–cortical circuits display volumetric reductions in patients with depression.43,44 Meta-analyses confirmed extensive prefrontal, limbic, and subcortical volumetric reductions in the rostral/pregenual ACC, dlPFC, dorsomedial PFC, OFC, insula, striatum, globus pallidus, thalamus, and hippocampus.45–47 In addition to these regional abnormalities, enlarged pituitary and adrenal glands have been described in depression.48,49 Other regions implicated in cognitive-affective processing, such as the cerebellum, have been less well studied and require further investigation.50
DTI uses T2-weighted scans and relies on the detection and quantification of the random movement of water. DTI is based on the principal that diffusion within the brain is limited by physical barriers, and the direction-dependent displacement of water molecules (i.e., axonal) is called anisotropy.51 The anisotropic fraction quantifies white matter microstructural integrity, and decreased fractional anisotropy (FA) is associated with loss of tissue organization (including axonal degeneration, disruption and partial breakdown of cytoarchitecture, or demyelination).
While DTI imaging has been under-utilized in depression research to date, several studies have characterized prefrontal and striatal white matter abnormalities.52,53 In a meta-analysis of 231 patients with depression and 261 healthy subjects, decreased FA was observed in the bilateral frontal, right fusiform and right occipital white matter in depressed subjects. The fiber tracts implicated in these regions included interhemispheric fibers from the genu and body of the corpus callosum, right inferior longitudinal fasciculus, and right inferior fronto-occipital fasciculus. Disruption of dlPFC-related white matter tracts have also been reported,54 including decreased FA in mediodorsal thalamus to dlPFC pathways.55
Postmortem studies of depressed patients compared to matched controls revealed frontolimbic cellular abnormalities consistent with in vivo structural MRI findings. Patients with familial forms of depression exhibited reduced glial cell counts in the subgenual ACC.56 A similar glial cell density and neuronal size reduction in the dorsal (supracallosal) ACC was found in mixed familial and nonfamilial depressed patients compared to schizophrenia, bipolar disorder, and nonpsychiatric controls.57 In addition to glial abnormalities, layer VI pyramidal neurons in the ACC showed decreased dendritic arborization in depressed patients who died of suicide compared to controls.58 In a specific postmortem analysis of the left prefrontal cortex, depressed subjects compared to psychiatrically healthy subjects exhibited rostral OFC reductions in cortical thickness, neuronal size, and neuronal and glial densities.59 In addition, glial density was reduced in the caudal OFC and neuronal size and glial cell counts were reduced in the dlPFC. These glial and neuronal dlPFC abnormalities have also been replicated.60 Left hemisphere lateralized total glial and oligodendrocyte density reductions in the amygdala have also been characterized,61,62 as have hippocampal neuronal cell count abnormalities, particularly in the dentate gyrus.63
Magnetic resonance spectroscopy (MRS) is an MR technique that measures the average amount of distinct metabolites in a given region of brain tissue. MRS detects and quantifies molecular signals based on their magnetic resonance properties. This technique takes into account the effect of the local chemical environment on particular nuclei. Commonly detected metabolites include: N-acetyl aspartate (NAA)—a marker of neuronal integrity; choline (Cho)—an indicator of cell membrane synthesis; creatine (Cr)—a marker of cellular energy metabolism. In addition, glutamate-related metabolites (including glutamate and glutamine) can be quantified separately or as a composite (termed Glx). Gamma-amino butyric acid (GABA).64 concentrations can also be measured.
MRS has detected abnormal glutamate, GABA and choline concentrations in depression. Decreased Glx (glutamate/glutamine) concentrations were noted in the dorsomedial/dorsal anterolateral and ventromedial prefrontal cortices in patients with depression compared to healthy controls.65 GABA levels were also reduced in the dorsomedial/dorsal anterolateral prefrontal cortex. Studies have similarly characterized decreased ACC, amygdala, and hippocampal Glx in depression.64 A meta-analysis of glutamate MRS abnormalities in depression found a consistent decrease in Glx and glutamate concentrations in the ACC.66 Apart from excitatory and inhibitory neurotransmitter abnormalities, depression has been associated with basal ganglia Cho/Cr increases.67 This finding suggests possible glial dysfunction or increased membrane turnover in depression.
Single proton emission computed tomography (SPECT), positron emission tomography (PET), and functional MRI (fMRI) have been used to study primary and secondary depressive syndromes. SPECT detects gamma rays and measures regional cerebral blood flow (rCBF), an indirect measure of neuronal activity. Commonly injected tracers include 99mTc-hexamethyl-propylene-amine-oxime (99mTc-HMPAO) and 99mTc-ethyl cysteinate dimer (99mTc-ECD). These lipophilic tracers cross the cell membrane and remain intracellular following conversion to hydrophilic compounds. Their incorporation is proportional to rCBF in the first few minutes after injection, and subsequent blood flow modifications do not alter initial tracer distribution.68 SPECT tracers have longer half-lives and are less expensive to manufacture than those used in PET. The limitations of SPECT include exposure to ionizing radiation and relatively limited spatial resolution, although technical advancements, including coregistration of rCBF images onto MRI scans rather than CT images have improved spatial resolution.
Brain PET imaging detects paired gamma rays emitted at 180 degrees from a given brain region and has improved spatial resolution compared to SPECT. There are several radionuclides commonly used including: carbon-11, nitrogen-13, oxygen-15, and fluorine-18. Fluorine-18 has the longest half-life and fluorodeoxyglucose (18F-FDG), an analog of glucose labeled with fluorine-18, is commonly used as a marker of regional brain metabolic activity. PET can also utilize antibody-radionuclide compounds to determine the distribution of particular brain molecules, and ligand-radionuclide compounds can measure the distribution of neurotransmitter receptors. These techniques have aided researchers in elucidating the in vivo neurochemistry of depression.
BOLD fMRI is a noninvasive, nonradiation-based neuroimaging technique that relies on the inherent magnetic properties of hemoglobin to provide a proxy for neuronal activity. Oxy- (diamagnetic) and de-oxy (paramagnetic) hemoglobin have distinct magnetic properties. Regional brain activity is associated with local vasodilation and increases in oxygenated venous blood concentration. Increases in oxy-hemoglobin due to elevated neural demands for oxygen and parallel compensatory increases in blood flow that exceed local metabolic requirements lead to elevated BOLD signal.69 While BOLD fMRI relies on venous blood flow, other methods such as arterial spin labeling (ASL) provide an arterial phase measure of cerebral blood flow with the potential for improved spatial and temporal resolution (currently ASL has diminished signal-to-noise compared to BOLD signal). fMRI provides high spatial resolution brain images, but has relatively poor temporal resolution given its reliance on the hemodynamic response (peaks at 5–6 seconds). fMRI is also highly sensitive to head movements and cardiorespiratory artifacts, and individuals with certain metallic implants are unable to participate in MRI-based imaging. The MRI environment is also loud and confining, and individuals with claustrophobia are typically not studied.
fMRI uses a broad array of paradigm and nonparadigm experimental designs and analysis techniques to probe structure–function relationships in depression. The two most widely used paradigm types include task-based and resting-state neuroimaging studies. Task-based studies serve as a “brain-stress test” to probe the functional integrity of a given circuit or set of circuits through task performances. Examples of this include working memory tasks to probe dlPFC function and the display of affectively valenced words or faces to characterize frontolimbic emotional processing. These functional neuroimaging methods compare task-of-interest activation patterns in patient populations of interest (i.e. depression) compared to those in healthy subjects (or other relevant control cohorts such as nondepressed patients exposed to early life trauma if the depressed patient group also experienced early life trauma) to identify regional differential task-related brain activations. In resting-state neuroimaging studies, no task or induction is employed. Rather, subjects are instructed to allow their mind to freely wander while low-frequency oscillations in BOLD signal are measured.70 This approach allows for functional connectivity analyses that probe coherent activation patterns within and across neural networks. While such analyses can also be performed with task-based imaging with great utility, their use in the resting state allows for particular investigation of the DMN, a collection of functionally coupled regions that activate during naturalistic “mind wandering” (and self-referential processing) and deactivate during many cognitive and affective tasks.
Functional neuroimaging studies in depression using SPECT, PET and fMRI demonstrate abnormal prefrontal cortex, basal ganglia, and medial temporal lobe activations (Fig. 2-8). One of the earliest functional brain imaging studies in depression was performed in 1985 using 18F-FDG PET and identified lower glucose metabolic rates in the left frontal cortex and bilateral caudate regions.71 This left frontal hypoactivation was replicated and refined to the left anterior-medial prefrontal cortex by other early investigations in depression using PET and SPECT.72,73 From a dimensional symptom perspective, early studies also linked decreased left dlPFC and striatum activations to psychomotor slowing in depression.74,75
Figure 2-8
Graphic depiction of commonly detected functional neuroimaging abnormalities in depression. dlPFC, dorsolateral prefrontal cortex; Ins, insula; OFC, orbitofrontal cortex; ACC, anterior cingulate cortex, d, dorsal; r, rostral; sg, subgenual; THAL, thalamus; HF, hippocampal formation. (Used with permission from David Vago PhD.)

Increased basal and stimuli-driven amygdala activity has been extensively characterized in depression.76 Depressed patients with a family history of depression demonstrated increased left amygdala activation in an early PET imaging study, and this pattern of amygdalar hyperactivation was also observed in remitted subjects with a family history of depression.77 This suggested that enhanced amygdalar activity potentially represented a trait neural biomarker for depressive illness. Subsequent fMRI studies using negative valenced emotional faces and linguistic stimuli delineated both initial elevated amygdala activity and prolonged activation (failed habituation) in patients with depression.78,79 A number of studies have specifically linked enhanced amygdala activity to the negative attentional bias of information processing in depression. Selective increased amygdala activity to sad facial expressions (compared to happy) was demonstrated in both acutely depressed and remitted patients.80 Importantly, enhanced amygdala activity to negatively valenced stimuli normalized following selective serotonin reuptake inhibitor (SSRI) treatment.80,81 In addition to an association with the negative attentional bias of depression, increased amygdalar metabolic activity positively correlated with plasma cortisol levels.82 This suggested a possible link between elevated amygdalar activity and increased hypothalamic–pituitary–adrenal (HPA) axis function.
Prefrontal cortex dysfunction also plays important roles in depression neurobiology. The subgenual ACC has been implicated in the modulation of negative mood states.83 Several neuroimaging studies characterized elevated baseline subgenual activation in depression,84–87 while other investigations have described reduced subgenual activations.88 The induction of a sad mood state in healthy subjects increased BOLD activity in the subgenual cingulate on fMRI.89,90 Treatment response to SSRIs, subgenual ACC deep brain stimulation (DBS) and anterior cingulotomy has been linked to pretreatment elevated subgenual ACC activity.84,86,91 Mayberg et al. have suggested that depression can be potentially defined phenomenologically as “the tendency to enter into, and inability to disengage from, a negative mood state.”92 A key disturbance in depression may not be the experience of a dysphoric mood state itself, but rather the inability to shift out of a negative mood state as if “stuck in a rut.” Subgenual ACC dysfunction is theorized to play a critical role in the inability to effectively modulate a negative mood state. Apart from the subgenual ACC, increased pregenual ACC activity has also been demonstrated in depressed subjects.93,94
In addition to the ACC, the OFC and dlPFC have exhibited dysfunction in depression. Consistent with OFC lesions linked to increased depression risk, depression severity inversely correlated with medial and posterior-lateral OFC activity in neuroimaging studies.95,96 PET neuroimaging after induction of sadness with autobiographical memory scripts revealed prominent deactivations in the medial OFC of acute and remitted depressed patients compared to rest conditions and healthy subjects.97 Given the efferent connections from the OFC to the amygdala, impaired OFC activations may disinhibit amygdalar output (including heighted activity in the bed nucleus of the stria terminalis) in depression. Meanwhile, the dlPFC may potentially exhibit a lateralized dysfunctional activation pattern in depression. While not consistently identified, depressed patients have demonstrated left dlPFC hypoactivity and right dlPFC hyperactivity98; left dlPFC hypoactivity was linked to negative emotional judgments, while right dlPFC hyperactivity was associated with attentional deficits. Modulation of the left dlPFC by repetitive transcranial magnetic stimulation (TMS) has been used to treat depression, and the degree of intrinsic anti-correlated functional connectivity between the targeted dlPFC site and the subgenual ACC may be linked to therapeutic efficacy.99 Such hemispheric-specific findings raise interesting questions about the importance of laterality in the study of depression circuitry, particularly in light of the fact, described above, that post-stroke depression tends to occur with left prefrontal lesions.
From a symptom-based perspective, decreased ventral striatum/nucleus accumbens activation has been linked to anhedonia. This is relevant in light of this region’s role in motivation, reward and reinforcement learning. Depressed patients exposed to positively valenced words in an emotional word fMRI study demonstrated significantly less bilateral ventral striatum activation to positive stimuli compared to healthy subjects; this study also observed a positive correlation between anhedonia and diminished ventral striatum activation (Fig. 2-9).100 This same cohort of patients in a task-based functional connectivity analysis demonstrated increased functional coupling between emotional processing regions (including the ventral striatum) and regions involved in linguistic, sensorimotor and self-referential (DMN) processing (Fig. 2-10).101 The lack of segregation of emotional processing from cognitive and sensorimotor functions potentially represented a systems-level neural substrate for a core phenomenon of depression: the interconnection of affective disturbance with perception, cognition and behavior. Hypoactivation of the ventral striatum/nucleus accumbens in patients with depression has been replicated using affectively valenced autobiographical prompts and a facial viewing task,102 as well as a monetary incentive reward processing task.103 Similar to previously discussed amygdala temporal activation abnormalities, the nucleus accumbens exhibited reduced duration of BOLD activation (enhanced habituation) during a cognitive, positive-emotion appraisal task.104
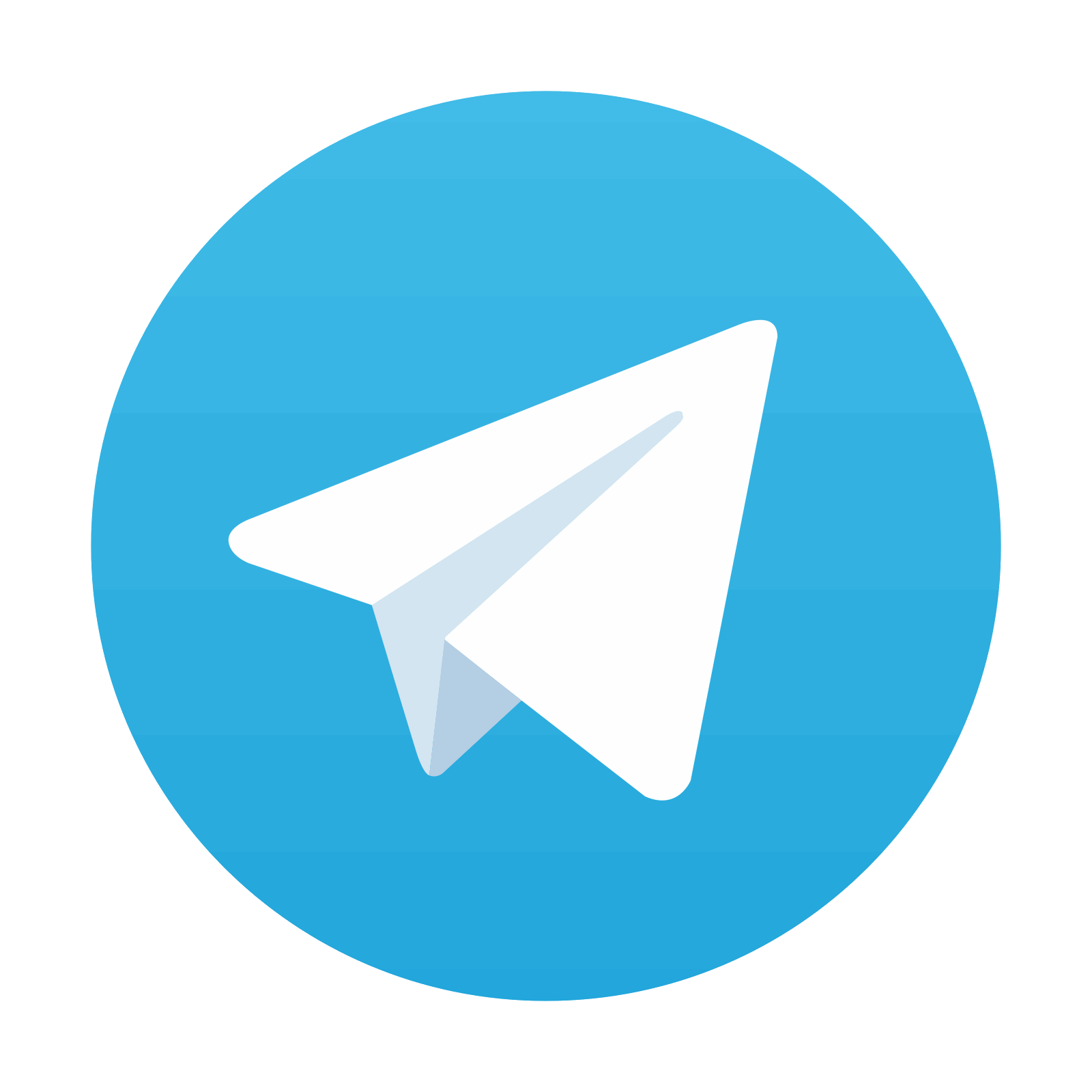
Stay updated, free articles. Join our Telegram channel

Full access? Get Clinical Tree
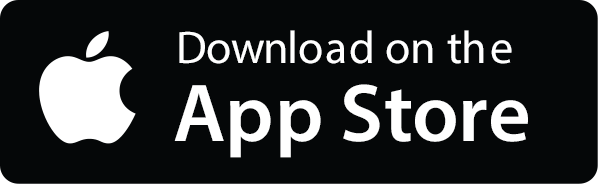
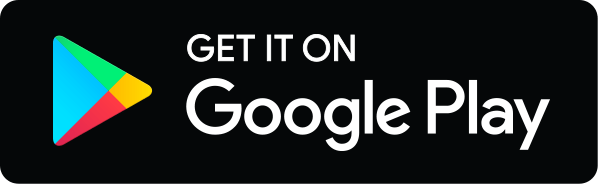