Fig. 8.1
Periventricular germinal cells line the ventricular cavity, seen at the bottom of the image. Asymmetric divisions of germinal cells provide for daughter cells that migrate to the cortex following a glial scaffold. Once in the cortex the neuroblasts will continue their differentiation to pyramidal cells. These excitatory cells provide the core of the cell minicolumn. Inhibitory cells, in their majority, will develop in synchrony with pyramidal cells but migrate to the cortex following a tangential path
Using a semiautomated computerized imaging method to analyze minicolumnar morphometry based on pyramidal cell arrays, Casanova et al. (2002a) studied Brodmann areas 9, 21, and 22 in postmortem tissues of autism spectrum disorder (ASD) patients. The population consisted of 9 ASD individuals (7 males and 2 females; mean age of 12 years) and 11 control subjects (7 males and 4 females; mean age of 14 years). Photomicrograph images were processed with a Gaussian gray-scale distribution and thresholded in order to define cellular kernels (Otsu 1979). A Euclidean minimum spanning tree was used to parcellate the kernels so as to minimize the total length of all of the lines joining them (Fig. 8.2). Minicolumnar width was defined as the mean distance between the tangential axes of pyramidal cell arrays. Other measured parameters included the relative dispersion of cells around the radial axis of each minicolumn, and the mean intercellular distance within minicolumns . Results of the study showed a significant reduction in minicolumnar widths with the greatest reduction found within their peripheral neuropil compartment.


Fig. 8.2
Panel on left shows a Nissl stained cross section of the cerebral cortex. The inset area, measuring 0.8 mm × 1.1 mm, is magnified on the right panel wherein a computerized algorithm identifies the presence of minicolumns. The method eliminates minicolumnar fragments usually less than 8 cells in depth
Casanova et al. (2002b) used the same patient population (vide supra) and analyzed the photomicrographs using a modification of the method developed by Schleicher et al. (2005) to assess thresholded images according to the gray level index (GLI). Intercolumnar distances, as defined by the intercluster width of GLI maxima values, were decreased and the mean number of clusters increased per given image. The results were consistent with previous findings indicating a greater number of narrower minicolumns in ASD.
The prior studies were followed by a multicenter international attempt to corroborate the minicolumnar findings. The study centered on an independent sample provided by the Autism Tissue Program (6 patients DSM-IV -TR and ADI-R diagnosed and an equal number of age matched controls). Serial whole hemispheric coronal sections embedded in celloidin were available for examination. Digital photomicrograph montages were taken of cortical Brodmann areas 3, 4, 9, and 17. Computerized image analysis with both the Euclidean minimum spanning tree and the GLI method were done blind to diagnoses. Minicolumnar widths were significantly diminished across all examined areas. Mean neuronal (cell body) and nucleolar cross sections were smaller in autistic individuals as compared to controls. Analysis of inter- and intracluster distances of edges obtained from a Delaunay triangulation suggested an increased number of minicolumns each one containing a normal number of cells. The authors indicated that the smaller cell bodies created a bias in corticocortical connectivity favoring shorter connections at the expense of longer ones. Also, the findings of an increased number of minicolumns, each one of appropriate cell density, suggested an increased number of symmetrical divisions of periventricular germinal cells during embryogenesis (Casanova 2013).
The nature of the proposed minicolumnopathy was furthered characterized by comparing minicolumnar widths across cortical laminae (Casanova et al. 2010). The study used postmortem material from 7 ASD individuals and an equal number of age matched neurotypicals sampled at 9 different locations: Brodmann areas 3b, 4, 9, 10, 11, 17, 24, 43, and 44. Each area was assessed at supragranular, granular and infragranular levels. Minicolumnar width diminution was equally observed across all examined levels. The findings suggested to the authors the involvement of an anatomical element in common to all levels. Given that the described minicolumnopathy is marked by a diminution of the peripheral neuropil space (i.e., the compartment holding most of the interneurons), a possible abnormality of the inhibitory elements surrounding the minicolumns is suggested.
The presence of inhibitory elements within the peripheral neuropil space of minicolumns has drawn the analogy to a strong vertical flow or shower curtain of inhibition (Szentágothai and Arbib 1975; Mountcastle 1998). Many of the symptoms (e.g., seizures, hyper- hypo sensitivities) attributed to an excitatory/inhibitory imbalance within the cerebral cortex of autistic individuals may be rooted in an abnormality of the peripheral surround of minicolumns. Electrophysiological experiments indirectly corroborate the presence of such a deficit in ASD. Casanova (2013) has proposed that the most parsimonious explanation to the minicolumnar findings is the forced and abnormal division of periventricular germinal cells during brain development . Heterochronic divisions of germinal cells would provide for the migration of neuroblasts to the cortical plate at a time when their positioning off from the radial glial scaffold would not be coordinated with the tangentially derived interneurons (Casanova et al. 2013).
8.5 Cerebellum
Neuropathological reports have often stressed the presence of histological abnormalities of the cerebellum in autistic individuals. Many of these abnormalities are of a dysplastic nature, suggesting a deviation during brain development in the organization of this anatomical structure. In general, dysplasias are often noted in the context of widespread cerebral malformations (Soto-Ares et al. 2000). Clinical information is seldom useful for diagnosing cerebellar dysplasias and classification is largely based on morphological assessments performed either by structural imaging methods or postmortem examination (Patel and Barkovich 2002).
Within the cerebellar cortex, Purkinje cells are linearly arranged and buttressed between a cell-poor molecular layer and the underlying granular layer. Several studies have called attention to diminished numbers of Purkinje cells in autism. Given that these cells are the only source of efferent projections of the cerebellar cortex, it is claimed that this abnormality could account for some autistic symptoms . Bauman and Kemper’s (1985) original case had bilateral Purkinje cell loss in the neocerebellum. Purkinje cell loss was more widespread when taking into consideration the ten cases in their series (Bauman 1991; Kemper and Bauman 1993; Bauman and Kemper 2005). Cell loss was observed in the posterolateral neocerebellar and archicerebellar cortices. Only two autistic individuals within their series showed pallor when staining for granule cells. Contrary to neuroimaging studies of vermal abnormalities in autism, Bauman and Kemper have reported no histological abnormalities in this region (Arin et al. 1991; Bauman and Kemper 1996). The lack of concomitant gliosis and absence of retrograde olivary cell loss made Bauman and Kemper suggest that Purkinje cell loss is most consistent with a lesion early during brain development .
Bauman and Kemper (1985) conclusions of an early developmental lesion have been questioned. Screening for the presence of gliosis using Nissl staining is unreliable and should be avoided. More recent studies using immunocytochemistry or Western blotting for GFAP suggest the presence of astrocytosis in the cerebellum of autistic individuals (Bailey et al. 1998; Laurence and Fatemi 2005; Vargas et al. 2005). GFAP belongs to a family of intermediary filament proteins that is expressed during the process of reactive astrocytosis and glial scar formation (Sofroniew and Vinters 2010). This upregulation of GFAP expression in autism is characterized by the presence of hypertrophic cell bodies and processes in a narrow and compact spread along the Purkinje cell layer. There is no reorganization of tissue architecture and no intermingling of processes across different astrocytic domains to suggest a glial scar formation or a severe focal lesion. Thus, the presence of a GFAP astrocytic response appears to be a reactive process to the loss of Purkinje cells.
Many autistic individuals suffer from epilepsy and may receive the anticonvulsant phenytoin as treatment. In addition some patients may engage in self -injurious behaviors, such as head banging, leading to contusions. Both seizures and phenytoin can provide for cerebellar atrophy and reduced Purkinje cell densities as a result of excitatory damage by the cerebro-cerebellar pathways (Crooks et al. 2000). Fronto-temporal contusions are associated to posterior lobe atrophy via a posttraumatic mechanism rather than to excitatory damage (Crooks et al. 2000). It seems possible that Purkinje cell loss in autism is, in part, the result of either or all of the above related conditions rather than a neurodevelopmental condition. In effect, Bailey et al. (1998) claimed that Purkinje cell loss during development was puzzling in lieu of the normal development of the cerebellar cortex. Moreover, these investigators also claimed that olivary cell loss is hard to assess as the cells normally show large variations in density and lie relatively far apart. It is therefore unsurprising that a later study by the Bauman and Kemper group comparing immunocytochemistry and Nissl staining indicated the possibility that agonal conditions and postmortem handling could account for inadequate Nissl staining and the lower Purkinje cell counts reported in their prior studies (Whitney et al. 2008).
8.6 Brainstem
Rodier et al. (1996) examined sections though the lower cranial nuclei of a single autistic individual. There were multiple abnormalities including a shortened distance between the trapezoid body and the inferior olive, and an absence of both superior olivary and facial nuclei. The authors concluded that, at least for the reported case, there was some type of injury that occurred around the time of neural tube closure. Congenital facial palsy is uncommon and when present it may be characteristic of the Moebius syndrome. Approximately 40 % of children with Moebius syndrome show symptoms typical of autism spectrum disorders (Gillberg and Steffenburg 1989).
Kulesza and Mangunay (2008) pursued Rodier’s finding and studied the superior olivary nucleus of 5 autistic subjects and 2 non-age matched controls. According to the ATP’s portal, one of the cases used in this study had a diagnosis of Fragile X-syndrome. Kuleszca and Mangunay (2008) concluded that there were significant morphological differences in the medial superior olivary nuclei in each of their 5 autistic cases as compared to controls. The age of their two controls (26 and 29 years) did not match the ages of the younger autistic patients (8 and 13 years). A more recent study by Thevarkunnel et al. (2004) showed no abnormalities of the superior olivary or facial nuclei in autistic individuals. Details of this work remain unknown as the original abstract presentation has not been published in a peer-review journal.
Another nucleus of the brainstem that has received attention in autism is the locus coeruleus. In Bailey et al. (1998) study on 6 autistic patients, one was described as having a widely dispersed nuclei and another one as having loosely grouped neurons. Martchek et al. (2006) used non-age matched hemisections (also not matched for hemisphere side) to perform a stereological study of this nucleus. They reported no significant between group differences in total cell counts, volume or numerical density of the locus coeruleus.
8.7 Axons
Hof et al. (1991) reported the neuropathological findings of a microcephalic autistic patient with premature closure of the cranial sutures. During life the patient exhibited a variety of self -injurious behaviors including head banging, eye-gouging, and self-biting. The gyral pattern at autopsy was normal but the brain exhibited generalized atrophy. At microscopic examination neurofibrillary tangles were found in the superficial layers of the perirhinal and entorhinal cortex and layers II and III of the neocortex. There was no evidence of concomitant amyloid accumulation. Given the severity of the patient’s self-injurious behaviors the authors suggested that the presence of neurofibrillary tangles was the results of a degenerative condition promoted by repeated brain trauma, i.e., dementia pugilistica. If this was the case, the patient must have exhibited thinning of the corpus callosum and diffuse axonal injury. Curiously the neurofibrillary tangle distribution in this case followed the same distribution as people with Alzheimer’s rather than mimic the location of contusive injuries (Jordan 2009).
Weidenheim et al. (2001) reported 2 cases of neuroaxonal dystrophy with secondary autism. Axonal swellings forming varicosities or spheroids were found within catecholaminergic and serotonergic nuclei. According to the authors, the topography of neuropathological changes suggested that limbic and other deep grey matter structures play an important role in the pathophysiology of autistic behaviors.
The density and thickness of axonal projections were studied using stereology in 5 autistic individuals and 4 age matched controls (Zikopoulos and Barbas 2010). Sampled sites included the white matter below the anterior cingulate cortex, orbitofrontal cortex, and lateral prefrontal cortex . Increased axonal density was reported below the anterior cingulate cortex and an excessive number of thinned axons below the orbitofrontal cortex. The findings indicate that at least anatomically there seems to be a bias towards shorter projections in autism (Casanova et al. 2006b).
The temporal lobes and telencephalic subcortical structures (i.e., medial and lateral forebrain bundles) were examined for serotonergic axons with immunocytochemistry (Azmitia et al. 2011). Thirteen autistic individuals and nine age matched controls were used in this study. Dystrophic serotonin positive axons were described in both forebrain bundles, amygdala, and in the piriform, superior temporal, and parahippocampal cortices. In analyzing the results the investigators did not take into account the preagonal and agonal conditions of the patients, length of fixation or postmortem interval.
8.8 Brain Weight
The fact that the brain, even after death, has the capacity to react to its environment is poorly appreciated by the research community. Within minutes after death autolysis, resulting from the release of enzymes within cells, is evident as part of the postmortem decomposition process. Within less than 4 h the gray matter shows a rapid increase in water and sodium and a fall in potassium content.
Later on bacterial proliferation leads to putrefaction. Several factors influence how rapidly these processes take place including environmental temperature, obesity, and the presence of septicemia.
All of the above mentioned factors influence fresh brain weight and my lead to spurious conclusions when taking this parameter as an index of pathology. It is therefore of importance that studies of fresh brain weights take into consideration the possibility of postmortem edema before attributing heftier specimens to either true macrocephaly or in vivo brain swelling. Usually convolutional flattening along with diminished ventricular size could suggest an in vivo phenomenon. However, histologic examination is of little use in ascertaining the presence of edema (Yates et al. 1975). It is usually agreed that neuroimaging studies in vivo allow for a more accurate determination of brain swelling than does post-mortem assessment: “The assessment of diffuse brain swelling at autopsy on the basis of convolutional flattening, obliteration of sulci and small symmetrical ventricles is complicated by the fact that some swelling of the brain is normally present post mortem. Neuroimaging in vivo allows more accurate and sensitive measurement of brain swelling than does macroscopic post-mortem assessment” (Blumbergs et al. 2008, p. 767).
Brain weight is also collected after formalin fixation. Depending on time of fixation and concentration of fixative solution (usually 10 % formalin solution, the equivalent of a 3.7 % formaldehyde solution) there will be a variable increase in brain weight . This increase will be more noticeable in children as compared to adults (Ludwig 2002). The findings are of significance when researchers perform studies on cellular density measurements instead of total cell counts.
Most studies on brain weight in autism have failed to disclose the conditions under which this parameter was collected. In some cases it is not possible even to say whether fresh or post-fixation brain weights were used alone or mixed together within the same sample. Furthermore, useful clinical indices to judge the validity of brain weights are usually not reported, e.g., cause of death, duration of terminal illness, terminal state of hydration (Itabashi et al. 2007). Given all of the above mentioned limitations the few available studies on postmortem brain weight in autism are of arguable significance.
In Williams et al. (1980) study the brains of their 4 autistic subjects (4 to 33 years of age) fell within 2 standard deviations of the mean for age. Hof et al. (1991) patient was microcephalic with a brain weigh of 773 g. Bauman and Kemper (1994) collected fresh brain weight s from 12 autistic children, aged 5–13 years, and 8 adults, aged 18–54 years. The investigators concluded that their data were in agreement with that of others suggesting an early acceleration followed over the course of ageing by an apparent deceleration in brain weight. The conclusions may stand to be corrected as the patient series included 3 obvious outliers.
Bailey et al. (1993) reported heavier brain weight s in 3 out of their 4 autistic subjects (age range 4–27 years). Microscopic examination did not reveal an increase in neuronal density. Two additional cases, with tissue available for microscopic examination, were added to their series (Bailey et al. 1998). Three of the six patients evidence brain swelling without herniation most possibly as a result of postmortem edema. One of the latter brains also showed signs of putrefaction.
Courchesne et al. (1999) reported on brain weight s of 5 new cases and 16 previously reported ones and compared them to 6 normative series. They concluded that brain weights for autistic subjects are usually within the normal range with rare cases of megalencephaly. The series was later pursued with a metanalysis on 55 specimens (Courchesne et al. 1999; Redcay and Courchesne 2005). The researchers concluded that there is divergence in brain weights early on in life that tends to normalize with ageing.
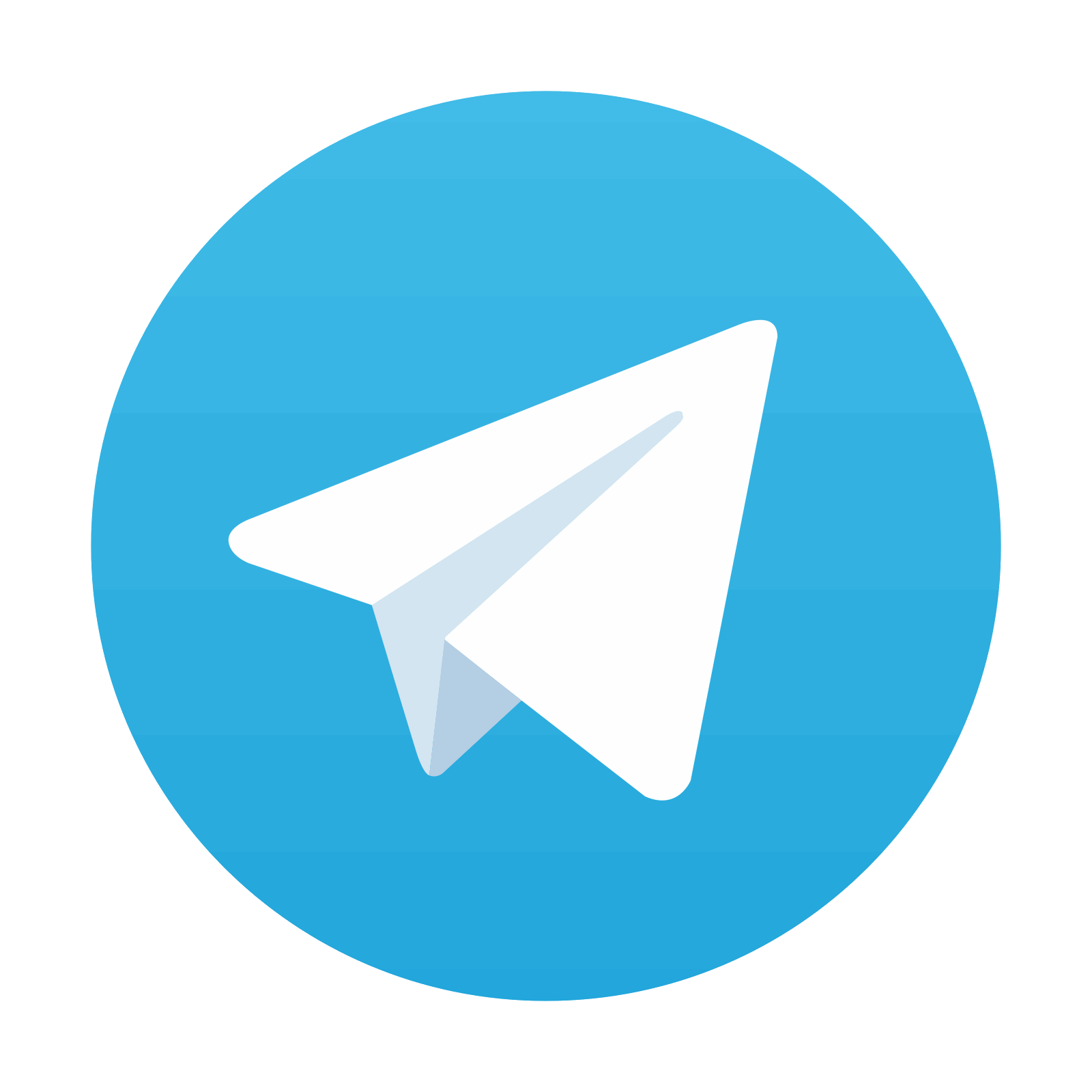
Stay updated, free articles. Join our Telegram channel

Full access? Get Clinical Tree
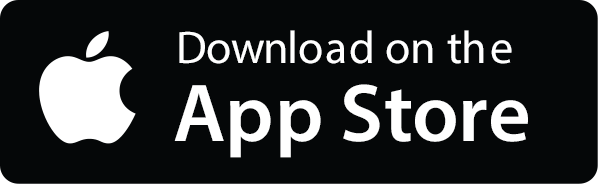
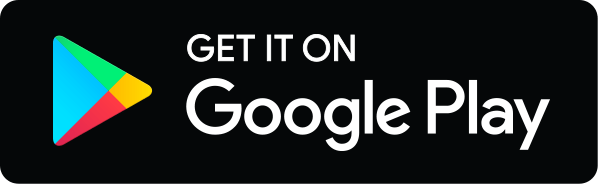