Fig. 4.1
Section stained for macrophage marker shows the presence of macrophages within the lesion border
White matter lesions in the brains and spinal cords of patients with progressive MS are predominantly chronically inactive in their appearance [7–10]. However, a significant number do show evidence of chronic inflammatory activity at the periphery of lesions. Lesions in the brains of patients with SPMS tend to have well demarcated edges separating them from surrounding white matter, a small number of perivascular or parenchymal T-cells and plasma cells, and few or no oligodendrocytes [8, 11]. One group has found patients with progressive MS to have a higher density of plasma cells within white matter lesions (as well as in the meninges and normal appearing white matter) than patients with acute or relapsing disease [12]. Astrocytes tend to be fibrous in appearance, though occasional large astrocytes are seen, and fibrous gliosis is evident within many lesions [8, 9]. Active inflammation tends to be in the form of a peripheral rim of foamy macrophages, in some cases containing myelin degradation products, while very few diffusely active white matter lesions are seen in the brains of patients with progressive MS [8, 9]. The white matter surrounding lesions tends to contain ramified microglia and foamy macrophages (without myelin degradation products) and a greater number of oligodendrocytes than within the lesion itself. In addition, however, the periplaque white matter also frequently contains clusters of microglia which are positioned along myelinated fibers and are associated with striking complement C3d deposition. These nodules appear as knots of several microglia/ macrophages which usually adopt a star-shaped configuration and surround individual myelinated or partially demyelinated nerve fibers. The microglial nodules are not seen within the white matter lesions themselves [8].
Moderate to intense IgG deposition can be seen throughout the demyelinated lesions of patients with both early and progressive MS, extending several centimeters into the surrounding white matter. IgG deposition is mostly associated with demyelinated axons and plasma cells, and seen within the plasma of blood vessels but is less often associated with microglia, macrophages, and astrocytes. However, this pattern of IgG deposition is a non-specific finding common to several neurological diseases where there is disruption of myelin. In a similar way, degenerating myelin in MS and other neurological diseases shows non-specific deposition of complement C3d and C9neo. The microglial nodules seen in periplaque white matter, on the other hand, appear specific to MS (though not specific to progressive MS) [8, 13].
Dispute exists over whether the immunological features of active demyelination differ between different patients and in particular between different disease courses. One group, who studied plaques showing evidence of active demyelination within an extensive collection of autopsy and biopsy specimens, reported four separate patterns of inflammation. The pattern of inflammation was reported to be constant within all the active lesions of one individual but to vary between individuals. While no straight forward relationship existed between lesion type and clinical course, one of the four lesion types was confined to three patients with PPMS. This lesion pattern was characterized by T-cell and macrophage infiltration, an absence of IgG and complement deposition, and an absence of oligodendrocytes within the lesions and periplaque white matter. The authors hypothesized that lesion type observed may relate to phenotypic differences between MS patients [14]. On the other hand, other groups have not found similar heterogeneity in early MS lesions and therefore speculate that any variation in histological appearance may simply reflect variations in lesion age [15, 16].
Certain white matter areas have been noted to exist in MS brains where axons have myelin sheaths which are thin in comparison to normal white matter but not completely absent. These pale areas are sometimes referred to as “shadow plaques.” At least some such areas are likely to represent areas of remyelination [17]. Pathology studies indicate that remyelination can be extensive during early MS but is less common at longer disease durations [8, 11, 13]. That said, one study analyzing white matter lesions in 36 patients with progressive MS found 42% of lesions to have some signs of remyelination. The degree of remyelination appeared fairly consistent across several lesions within the brain of one individual, suggesting that the capacity for remyelination may be determined individually [18].
The low potential for remyelination in patients with progressive MS is likely to be explained by a failure of oligodendrocytes to repopulate demyelinated lesions. As mentioned above, chronic demyelinated lesions tend to be almost devoid of mature oligodendrocytes. Repopulation of the demyelinated area is dependent on migration and differentiation of oligodendrocyte precursor cells to the region. Although oligodendrocyte precursor cells are present in higher numbers than oligodendrocytes in the white matter demyelinating lesions of progressive MS, their numbers decrease with increasing age of the patient, increasing disease duration, and decreasing macrophage populations. Moreover, the oligodendrocyte precursor cells tend to appear quiescent [19, 20]. Interestingly, the number of oligodendrocyte precursor cells within white matter lesions appears to be even lower in the spinal cord than in the brain [20]. Immature oligodendrocytes are frequently seen within white matter demyelinating lesions in early MS [11]. On the other hand, patients with longstanding progressive MS show low numbers of immature oligodendrocytes. The majority of those oligodendrocytes that are present in lesions of progressive MS possess markers indicating maturity but have a small nucleus containing clumped nucleochromatin, little cytoplasm, and lack myelin forming processes. These oligodendrocytes are likely to be the few that survived the demyelinating process but no longer function as normal. The remainder of the visible oligodendrocytes have an immature appearance and are likely to be derived from oligodendrocyte precursor cells. However, few are engaged in myelination [19].
Leakage of the blood-brain barrier is another classical component of MS white matter demyelinating lesions, which is thought to cause the appearance of contrast enhancement on MR imaging. However, while blood-brain barrier leakage is a recognized feature of early demyelinating lesions, several histological studies have suggested that low-grade blood-brain barrier disruption persists in white matter lesions late into the disease [21–24]. Given that fibrinogen leakage can induce activation of microglia [25], chronic low-grade blood-brain barrier leakage could play a role in perpetuating low-grade inflammatory activity in progressive MS. However, the relationship between blood-brain barrier leakage and inflammation in chronic MS appears more complicated, as another study revealed apparent blood-brain barrier leakage in vessels which did not have perivascular cuffs of immune cells as well as blood vessels with perivascular cuffs which did not have evidence of blood-brain barrier breakdown [26].
Axonal loss is well recognized to occur within white matter demyelinating lesions in MS and may differ according to disease course. Markers of acute axonal injury suggest that this is most prevalent during the early stages of relapsing–remitting MS. Acute axonal injury does appear to occur within long-standing white matter lesions in progressive MS but with a lower incidence [27–31]. Although overall levels of inflammation are low in white matter lesions of patients with progressive MS, acute axonal injury still appears to correlate with the degree of inflammation even in this patient group [12]. Analyzing axonal number is best achieved in the spinal cord and in the corpus callosum where axonal orientation allows for easier quantification. Studies of axonal density in spinal cord lesions confirm that patients with progressive MS show striking axonal loss compared with surrounding white matter [10, 32, 33].
4.3.1 Diffuse White Matter Pathology
It is increasingly recognized that white matter beyond discrete demyelinating lesions is abnormal in multiple sclerosis. What is often referred to as the normal appearing white matter in MRI studies, can be demonstrated to possess features of disease when examined under the microscope. Diffuse changes within the normal-appearing white matter seem most extensive in progressive subtypes of multiple sclerosis. In particular, patients with progressive MS appear to have diffuse low-grade inflammation in the white matter comprising perivascular cuffs of monocytes, T-cell infiltration, and microglial activation (Fig. 4.2) [7]. One group has also found higher numbers of plasma cells in the non-lesional white matter in the brains of patients with progressive MS than in those of patients with acute or relapsing disease [12]. Perhaps related is the diffuse low-grade disruption to the blood-brain barrier [22, 23]. Furthermore, there is evidence of low-grade axonal damage which exceeds that seen in patients with relapsing–remitting MS [7]. Further, diffuse pathology in the white matter of patients with progressive MS includes relatively low density of myelin and excessive fibrillary gliosis [34].


Fig. 4.2
T-cell marker shows the presence of lymphocytic cuffing around venules at the lesion border and in the normal-appearing white matter
4.4 Grey Matter Lesions
The presence of grey matter demyelination in MS has been reported since as early as the end of the nineteenth century [35–38]. Brownell and Hughes’ autopsy study, conducted on 22 cases of MS, acknowledged the presence of grey matter demyelination, although authors found most lesions involved both white matter and cortex at the leukocortical junction while only 5% of lesions seen in the cerebral hemispheres were truly intracortical [39]. Later, Lumsden also reported the existence of cortical demyelination with some individual subjects showing extensive regions of demyelinated cortex [40]. These studies are likely to have considerably underestimated the extent of grey matter demyelination, since more recent histopathological studies show that immunohistochemical methods targeting myelin basic or proteolipid proteins (MBP and PLP) are far more sensitive than histochemical methods [41, 42].
Grey matter demyelination affects the cerebral and cerebellar cortices, deep grey matter structures of the brain, and also the grey matter of the spinal cord. Several studies have revealed that the extent of cortical demyelination varies greatly between individuals [7, 43, 44]. Patients with relapsing–remitting disease appear to have a limited amount of cortical demyelination, around 2–3% of their cortical area [7, 44]. In contrast, patients with progressive MS demonstrate more extensive demyelination, involving around 12–25% of their cerebral cortical area [7, 43, 44]. Extensive demyelination of the cerebellar cortex has also been found to be a hallmark of patients with progressive versus relapsing–remitting MS [45]. In some patients with progressive MS, there is evidence of very extensive cortical demyelination of a subpial type (referred to as generalized subpial demyelination). In such patients, up to 50–60% of the entire cortical area may be demyelinated [42, 44].
Cortical demyelination appears to show regional variation being most extensive in the cingulate gyrus followed by the temporal cortex, frontal cortex, and parietal cortex [42, 44, 46]. This pattern of regional variation may relate to restricted CSF flow, as regions deep within the cortical folds are particularly affected [47]. Indeed, the hippocampus has been shown to be affected by demyelination in patients with MS [48]. The globus pallidus and thalami have also been specifically studied and noted to be also affected frequently by demyelination [44, 46]. However, compared with cerebral structures, the extent of demyelination has been found to be highest in the cerebellum and grey matter of the spinal cord where typically 40–50% of the grey matter is demyelinated in patients with long-standing MS (Fig. 4.3) [45, 46]. All levels of the spinal cord appear equally affected by grey matter demyelination [49].


Fig. 4.3
Cervical spinal cord section from a patient with progressive MS showing multiple white and grey matter lesions with varying degree of demyelination
Overall, the extent of grey matter demyelination, expressed as a proportion of tissue studied, appears greater than the extent of white matter demyelination, although there is a chance this may be affected to some extent by sampling bias. Interestingly, the extent of grey matter demyelination appears unrelated to the degree of white matter demyelination in individuals, and those subjects with a heavy burden of cortical demyelination may show little white matter demyelination [7, 42, 44, 49, 50].
The morphology of lesions within the cerebral cortex seems to be fairly stereotyped. A scheme of classification proposed by Peterson et al. categorizes grey matter lesions as falling into three main groups: type 1 lesions involve both grey and white matter, their center often appearing to fall within subcortical white matter; type 2 lesions are located within grey matter but do not extend to the surface of the brain nor to the leukocortical junction; type 3 are subpial lesions that often involve long stretches of cortex of several neighboring gyri [51].
In those studies using IHC, similarities have emerged in the relative contribution of the different lesion types to the overall burden of cortical demyelination. Less than a third of demyelination affecting the cortex appears to simultaneously involve white matter (type 1 lesions) while the remainder are entirely intracortical [9, 42–44, 46, 51–53]. Of those with purely intracortical demyelination, type 3 demyelination accounts for the vast majority of lesions (around 50–70% by number or 75% by area) [42, 43, 46, 51–53]. In contrast, in the cerebellum and spinal cord, more lesions involve both grey matter and white matter concurrently [46]. Nevertheless, the majority of cerebellar cortical lesions also adopt a subpial morphology with demyelination extending to a variable depth within the granular layer [45].
The finding of different morphological lesion types has led some to hypothesize that different cortical lesions possess different mechanisms of pathogenesis [51]. It has been postulated that factors arising from the CSF or meninges invoke subpial demyelination [7, 51]. In keeping with this hypothesis is the more recent finding that B-cell follicles, possibly representative of tertiary lymphoid tissue, can be identified within the meninges of some MS subjects, particularly adjacent to subpial plaques [54, 55]. The aggregates comprise B-cells, T-cells, plasma cells, macrophages, and a network of follicular dendritic cells. Their morphological appearance, the presence of proliferating B-cells, and the expression of lymphoid homing chemokines, all strongly support the idea that these structures represent ectopic lymphoid follicles. Similar structures are seen in the thyroid, synovial tissue and salivary glands of patients with Hashimoto’s thyroiditis, rheumatoid arthritis, and Sjögren’s disease, respectively. It is proposed that lymphoid neogenesis in chronic autoimmune diseases leads to perpetuation of a compartmentalized immune response [56]. Interestingly, lymphoid follicles appear to occur in the brains of around 40–50% of patients with SPMS but have not been identified in the meninges surrounding the same patients’ spinal cords [54, 57]. Patients with lymphoid follicles seem more likely to have an aggressive disease course (younger ages of onset, irreversible disability, and death) and also demonstrate more extensive cortical pathology. In particular, there is a strikingly immediate proximity between meningeal lymphoid follicles and subpial cortical lesions. This translates to a fivefold higher extent of cortical demyelination in patients with versus without lymphoid follicles (most of which was accounted for by subpial lesions). The patients with lymphoid follicles also show more cortical microglial activation, and lower numbers of cortical neurons, oligodendrocytes, and astrocytes than patients who lack lymphoid follicles. While patients with meningeal lymphoid follicles seem more likely to have B-cells and plasma cells in the perivascular spaces of white matter lesions than patients who lack lymphoid follicles, they do not show any significant difference in their overall extent of white matter demyelination. Lymphoid follicles are almost always located within sulci, often at their deepest point [54, 57]. Similar structures suggestive of ectopic lymphoid follicles have been observed in one case of tuberculous meningitis but not in eight other cases of non-MS inflammatory neurological disease [57].
Interestingly, subpial demyelination may be specific to MS; patients with progressive multifocal leukoencephalopathy show intracortical and leukocortical lesions but no subpial lesions [47]. Another possible explanation for the stereotyped morphology of certain cortical lesions is their relationship to cortical veins. Kidd et al. found the majority of cortical lesions in the post mortem brains of 12 MS patients to arise within the territory of a principle vein [58].
The constituents of grey matter demyelinating lesions differ from those found in white matter. The main difference appears to be the relative hypocellularity of grey matter demyelinating lesions. Numerous authors document a higher degree of inflammatory infiltrates in white matter lesions versus grey matter lesions [42–44, 51]. In addition, MHC class-II positive cells in grey matter lesions often exhibit the appearance of active microglia, in contrast to the predominance of lipid-laden macrophages in comparable white matter lesions [43, 51]. A consequence of the low cellularity of grey matter lesions is that they are difficult to detect macroscopically in autopsy specimens and are also not highlighted by routine histological stains such as hematoxylin and eosin [59]. The differences in cellularity of white matter and grey matter demyelinating lesions may arise because of regional differences in pathogenesis, or as a result of the same pathogenesis occurring in different microenvironments.
In line with studies of white matter lesion pathology, grey matter lesions have been shown to exhibit neuroaxonal loss compared with surrounding myelinated grey matter. Investigation of post mortem brains of seven patients (of whom six had progressive MS) revealed transected dendrites and axons within cortical demyelinated lesions [51]. The frequency of transected neurites correlates with the degree of inflammation with actively inflamed lesions demonstrating a 500-fold increase in transected neurites in comparison with myelinated MS cortex. Apoptotic neurons are also seen within demyelinating cortical lesions, predominantly in layers III and V, with an incidence 100-fold higher than in myelinated MS cortex. The microglia in cortical lesions have an activated appearance and often extend processes which encircle adjacent neurites and neurons, though it is not clear whether this association is destructive or protective [51]. Subsequent studies have also found reductions in neuronal and synaptic density within cerebral and cerebellar cortical lesions as well as spinal cord grey matter lesions compared with local myelinated grey matter [44, 45, 53, 60].
As grey matter demyelination remains relatively difficult to detect using in vivo imaging methods, little can be said of the clinical implications of grey matter demyelination. Its higher prevalence in patients with progressive disease raises the possibility that it may relate to progressive disability. Limitations in clinical data that accompany most pathology studies have so far made it difficult to further address this hypothesis. However, one pathological study did find that patients with extensive cortical demyelination may have a higher incidence of cognitive impairment [42]. In another study, clinical signs suggestive of cerebellar involvement were found in medical records of patients who had extensive grey matter demyelination in the cerebellum regardless of the degree of white matter cerebellar disease [45].
4.5 Neuroaxonal Pathology
The most convincing candidate as the pathological substrate underlying chronic disability in MS is neuroaxonal loss. Indeed, if other pathological processes contribute to progressive disability in MS, it is likely that they do so by invoking a final common pathway of neuroaxonal dysfunction or death. We have recently shown that patients with higher motor disability before death had fewer corticospinal tract axons compared to more mobile MS patients when the spinal cord was examined post mortem.
Despite the fact that MS is largely regarded as a demyelinating disease, the presence of neuronal and axonal damage is well established. Early neuropathology reports of neuroaxonal injury were largely descriptive, whereas more recently, authors have attempted to quantify the degree of axonal loss in MS, identify its location and its association with inflammatory demyelination.
Histological methods such as silver staining or immunostains targeting neurofilaments both allow comparison of axonal number between the CNS tissue of MS subjects and controls. An alternative method that has been used to quantify axonal injury is the identification of axonal transection or interruption of axonal transport. Axonal stains themselves can facilitate the identification of axonal transection by demonstrating swollen axonal end bulbs – so-called spheroids or ovoids. Immunohistochemical staining of beta-amyloid precursor protein (βAPP), a protein that is anterogradely transported along axons can also be used as a marker of axonal injury, as it rapidly accumulates at sites of interrupted axonal transport. Positive staining for βAPP indicates recent damage but APP is thought to disappear some days after axonal injury, so is only sensitive in areas where there is ongoing axonal injury. Furthermore, interruption of axonal transport is not synonymous with axonal death; it may be transient and followed by axonal recovery [61]. TUNEL (Terminal deoxynucleotidyl Transferase Biotin-dUTP Nick End Labeling) is a method used to detect cells undergoing apoptosis. This technique labels fragments of DNA produced during apoptosis with biotin-dUTP; the biotin-dUTP can subsequently be visualized using standard IHC methods. Much like βAPP, TUNEL-positive neurons are likely to be cleared from the tissue within a short time of formation (perhaps 24 h [62]), and therefore reflect evidence of recent apoptosis.
Several groups have shown extensive axonal loss within the white matter tracts of patients with MS versus non-neurological controls (Fig. 4.4) [10, 33, 63–66]. Estimates of the degree of axonal loss vary from around 25–65% depending on the region studied and the methods employed. Furthermore, there appears to be pathological evidence of considerable axonal dysfunction even prior to axonal degeneration in progressive MS, which may contribute to disability [67].


Fig. 4.4
Sections from the corticospinal tract of a patient with MS (a) and a non-neurological control (b) stained for neurofilament. Axonal loss is apparent in section (a), particularly the loss of small-diameter axons. Scale bar represents 100 μm
Using various methods mentioned above, several studies have demonstrated that neuroaxonal injury appears to be centered on demyelinating lesions compared with the same subject’s non-demyelinated parenchyma [10, 12, 27–30, 44, 51, 53, 68, 69]. Furthermore, there is substantial evidence that the degree of neuroaxonal damage relates to the amount of inflammation within demyelinating lesions [12, 28, 30, 51, 57, 70]. Nevertheless, acute axonal injury has been demonstrated within the normal appearing white matter and seems to be more prevalent in progressive disease than in acute or relapsing MS [7, 12].
The suggestion of a causal association between inflammatory demyelination and axonal loss is also supported by the finding of a strong correlation between lesion load in cerebral white matter areas and axonal number in their relevant projection areas within the corpus callosum [71].
4.5.1 Possible Mechanisms for Neuroaxonal Pathology in MS
The available evidence suggests some interplay between inflammatory demyelination and neuroaxonal injury, raising several possibilities in terms of mechanism. First, the correlation between the degree of inflammation in demyelinated lesions and the degree of axonal injury found by several authors, lends support to the theory that bystander effects of inflammation cause axonal damage. Possible mediators of damage include cytokines such as TNFα or IFNγ or compounds capable of inducing oxidative damage such as the nitric oxide released from activated macrophages [72, 73]. Other proposed mechanisms are neuronal excitotoxicity resulting from the production of glutamic acid at sites of inflammation [74] or alternatively, the mechanical compression of axons due to local oedema.
A second possibility to explain the co-localization of demyelination with axonal damage is that oligodendrocyte death occurring during inflammatory demyelination has repercussions on axonal viability. Oligodendrocytes have been recognized as fulfilling roles of axonal support above and beyond their contribution to CNS myelination [75]. It seems possible that they help to sustain local axons by means such as the production of trophic factors or the mediation of axonal metabolism. Oligodendrocyte precursor cells are known to exist in the adult CNS and represent a possible pool of cells capable of replenishing local oligodendrocyte population in demyelinated areas. However, evidence that OPCs have a limited capacity for repopulating demyelinated CNS lesions, particularly in older subjects [19, 20, 76], suggests that neurons and axons within demyelinated areas may become chronically depleted of trophic support.
An alternative theory to explain the co-localization of demyelination and axonal damage is that demyelinated axons are more susceptible to autoimmune attack. The exposure of cryptic epitopes from previously privileged sites is known to be associated with the production of autoimmune attack in some circumstances. This mechanism would be compatible with exposure of antigens on the axolemma previously covered by myelin. The identification of intrathecal antibodies against axonal proteins in patients with MS [77–80], along with the finding of ectopic lymphoid tissue in close proximity to cortical lesions [54], could support the concept of a humoral contribution to chronic neuroaxonal injury in MS. However, there is no evidence that the antibodies are pathogenic. Furthermore, small axons, which tend to lack a myelin sheath [81], are preferentially lost in MS [65, 66]. This implies that many of the axons that are lost in MS are unmyelinated to begin with and thus injury may not be attributed to loss of their myelin sheath per se.
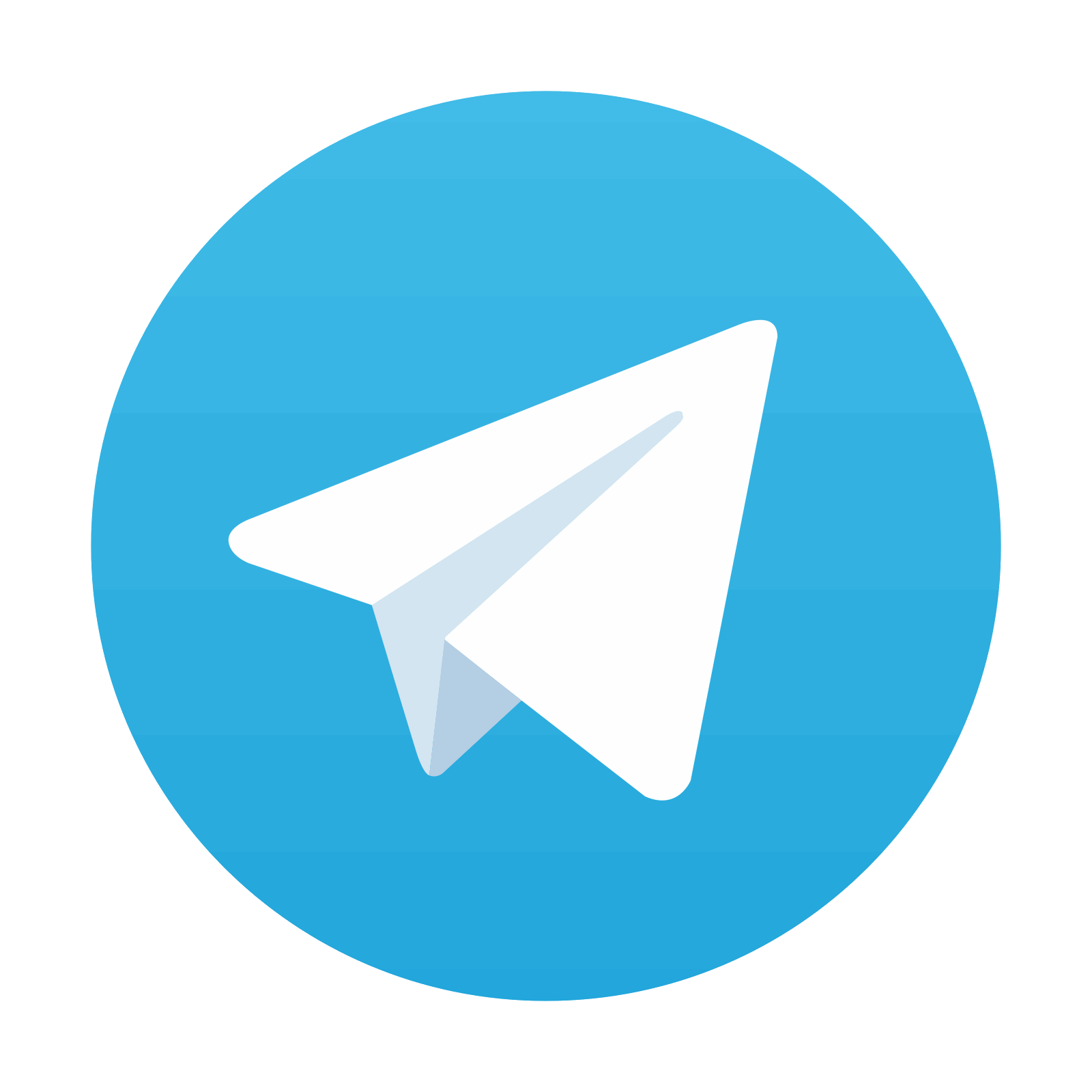
Stay updated, free articles. Join our Telegram channel

Full access? Get Clinical Tree
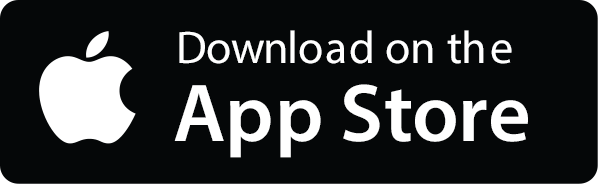
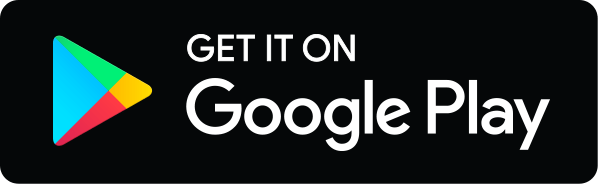