The purpose of this article is to update the neurosurgical community on the role of adjuvant radiation therapy in the management of patients with high-grade glioma. This information guides clinicians in the multidisciplinary management of these patients via a review of the literature describing current treatment paradigms as well as new avenues of investigation.
High-grade gliomas (HGGs) encompass the most malignant and the most commonly encountered primary brain neoplasms in clinical neuro-oncology practice, with an incidence of 5 cases per 100,000 people. They are commonly defined to include World Health Organization grade III and IV glial-based neoplasms, most frequently glioblastomas, anaplastic astrocytomas, and anaplastic oligodendrogliomas. A multidisciplinary approach to the management of these tumors includes surgical resection when feasible, chemotherapy, and radiation therapy. Each of these treatment components has contributed to increased survival in patients with HGGs, with an improvement in mean survival of 8 to 15 months over the past 40 years.
Radiation therapy has become a standard component of the management of HGGs since the 1970s. Advances in the understanding of radiobiology have led to refinements in the delivery methods of radiation, resulting in optimization of radiation therapy in terms of dosage, fractionation schedule, conformality, and treatment modalities. Further development of new radiation technologies such as stereotactic targeting and delivery has allowed physicians to investigate whether focal radiation treatment may improve patient outcomes as well.
Radiobiology
The primary physiologic mechanism of radiation therapy is through DNA damage; however, recent advances in the understanding of radiobiology have shown that ionizing radiation triggers a variety of complex and dynamic responses in both normal and neoplastic cells, leading to the concept of the cell damage response. There are 5 core concepts of radiobiology within this concept of the cell damage response (the 5 Rs of radiation): repair, repopulation, reoxygenation, redistribution, and radiosensitivity. These 5 concepts are being further refined at the tissue, cellular and molecular level as our understanding of radiobiology advances.
Repair
The molecular biology of repair includes responses to various different types of cell death, including mitotic death (within 1 to 2 cell cycles), interphase death (death of sensitive cells within hours), apoptotic death (programmed cell death), necrotic death (caused by a pathologic rather than physiologic process), and autophagy. Current studies support that in response to DNA damage, cells trigger elaborate signaling pathways to repair the most lethal DNA damage, including double-stranded DNA breaks and the induction of apoptosis. Complicated mechanisms of repair include base excision repair, single-strand repair, homologous and nonhomologous recombination of double-strand DNA breaks, and chromatin remodeling. Additional cell death pathways and modulators are manipulated to increase repair, including the p53-dependent apoptosis pathway, survival proteins such as survivin, and epidermal growth factor receptor (EGFR)-mediated signaling for increased repair.
Repopulation
Repopulation refers to the ability of neoplastic cells to repopulate after radiation treatment. This repopulation shows a lag period after radiation treatment followed by the triggering of an enhanced regenerative process or accelerated and aggressive repopulation. The onset time of this repopulation is variable, but growth factor signaling has been found to play an important role in accelerated regeneration of cancer stem cells (CSCs) after radiation.
Reoxygenation
Oxygenated neoplastic cells are more sensitive to radiation treatment secondary to the availability of oxygen to participate in the generation of DNA damage via the generation of free radicals. Reoxygenation of hypoxic tumor cell populations is arrested via the cell damage response, with hypoxia-induced genes, EGFR signaling, vascular endothelial growth factor (VEGF) upregulation, and neovascularization. Hypoxia inducible factor 1 also functions in a variety of pathways to create enhanced neoplastic cell survival mechanisms and radioresistance.
Redistribution
Redistribution of cells refers to the transition of cells through their natural cell cycle, with differences in their sensitivity to radiation at different points within the cycle. Cells within the mitotic phase are most sensitive to radiation damage, thus conveying radiosensitivity to dividing neoplastic cells over normal cell populations in other phases of the cell cycle. However, ionizing radiation has been shown to trigger cyclin-dependent kinase and EGFR signaling, which interferes with cell cycle progression and results in the arrest of neoplastic cells in relatively radioresistant phases.
Radiosensitivity
Radiosensitivity reflects how cells have different intrinsic genetic sensitivities to radiation and usually reflects the shoulder in cell survival curves that can be manipulated by various radioresistance pathways. The principles of radiobiology described earlier can all be manipulated to convey radioresistance, via enhanced DNA repair, increased repopulation responses, hypoxia, and inhibition of cell cycle redistribution.
Advances in Understanding Radioresistance: The Perivascular Niche, CSCs, and Linear-Quadratic Modeling
The tumor microenvironment, with its heterogeneous cell populations of fibroblasts, endothelial cells, reactive inflammatory cells and microvascular proliferating structures, is crucial to tumor expansion and response to radiation. Ionizing radiation induces a series of events that can lead to a microenvironment favorable to neoplastic growth and radiation resistance. These pathways include a composite of cell loss and damage, gene alteration, induced gene products, immunosuppression, and hypoxia. Recent evidence suggests that the glial microenvironment, also termed the perivascular niche, facilitates expansion and differentiation of brain tumor stem cells. Cell types, such as those listed earlier, are present in the perivascular niche and promote cell damage response and signaling pathways that initiate repair mechanisms resulting in radioresistance. The presence of hypoxia promotes the persistence of stem cells and induces the upregulation of proangiogenic factors such as VEGF. Complex immunosuppressive pathways are activated, including transforming growth factor β 1, which acts to suppress antitumor immune responses, enhance extracellular matrix production, and augment angiogenesis, making neoplastic cells more radioresistant.
The concept of the neural stem cell has also further refined concepts of radiobiology and resistance. Recent insights into tumor biology suggest that in many cancers only a small subset of cells, defined as CSCs, have the potential to survive and proliferate indefinitely. This small subpopulation of cells resists the exponential cell kill that is generated during fractionated radiation therapy. Consequently, these CSCs are believed to be a primary reason that HGGs recur and are resistant to known forms of therapy. Research has shown that CD133+ CSCs increase after tumor radiation, conveying radioresistance secondary to increased activation of DNA damage response. Further identification and research of these CSCs is required to determine their role in glioma pathophysiology.
The cumulative impact of the classic concepts of radiobiology as well as new concepts of the perivascular niche and the CSC collectively form the unique response profile of a tumor to radiation as well as the response of normal surrounding tissues. Advances in mathematical modeling of the radiation responses of HGGs have led to the application of the linear-quadratic (LQ) model. The LQ model is used to calculate biologically effective doses (BEDs) to compare various treatment modalities or fractionation schedules.
This model can be used to extract BEDs from historical treatment cohorts to compare across radiation modalities and fractionation schemes. In addition, the LQ model can also be used to gain understanding about how specific tumor types respond to radiation and to predict how this response might vary with alterations in fractionation and dosage.
[Tags: Radiobiology, repair, repopulation, redistribution, reoxygenation, radioresistance, CSCs, perivascular niche, linear-quadratic model].
Development of the current radiation treatment paradigm
Initial Studies and Proof of Efficacy
Radiation therapy was initially observed to provide survival benefit in patients with gliomas in a clinical trial performed in 1967. Subsequently, a randomized controlled trial in 1978 confirmed this survival benefit. In this trial, patients who received treatment with BCNU chemotherapy and radiotherapy displayed a 16-week survival advantage over patients receiving chemotherapy alone (34.5 weeks vs 18.5 weeks, P = .001). This trial established the efficacy of radiation treatment in the multimodal therapy for gliomas, with a treatment model of 50 to 60 Gy delivered to the entire brain via 5 fractions per week for 5 to 7 weeks. Subsequent randomized controlled trials have confirmed the survival benefit conveyed by radiation therapy.
Effect of Cumulative Radiation Dose on Efficacy
With the knowledge that adjuvant radiation is efficacious, additional studies aimed to address the effect of cumulative radiation dose on survival. These studies displayed an increase in overall survival as the cumulative dose is increased up to 60 Gy. As the cumulative dose is increased past 60 Gy to doses up to 70 Gy, no additional survival benefit is identified. In addition, analysis of patient outcomes treated with dose escalation as high as 90 Gy has shown that recurrence still occurs locally, within radiation delivery. Because of these negative studies, 60-Gy radiation therapy for HGG has remained the standard cumulative dosage.
Effect of Hyperfractionation on Efficacy
Fractionation of radiation therapy represents another variable that has been manipulated in the attempt to optimize outcomes in patients treated with radiation. Hyperfractionation is the use of a larger number of smaller-dosed radiation fractions and can be performed on a standard or accelerated schedule. Hyperfractionation has the positive effects of allowing repair of radiation damage in normal nonneoplastic tissues, redistribution of neoplastic cells to radiosensitive portions of the cell cycle, and reoxygenation of tumor cell populations that renders them more radiosensitive. Hyperfractionation also carries the negative effect of allowing time for the neoplastic cells to repopulate. Standard conventional radiation therapy is currently delivered in a hyperfractionated format, with 30 fractions delivered over 6 weeks.
With the goal of combating fraction-related neoplastic cell repopulation, multiple trials have analyzed the survival outcomes with hyperfractionated radiotherapy into even smaller and more frequent fractions, with most as well as pooled meta-analyses showing no survival advantages (1 smaller trial reported a 10-week survival benefit with further hyperfractionation). A follow-up Radiation Therapy Oncology Group (RTOG) phase III trial also showed no efficacy from hyperfractionation of 72 Gy delivered in 2 daily fractions of 1.2 Gy over 6 weeks.
Effect of Radiation Volume on Efficacy
Analysis of failures from radiation therapy has shown that recurrence occurs locally, within 2 cm of enhancing tumor. This finding is in contrast to many other malignancies that spread via metastasis or cerebrospinal fluid dissemination. The pattern of local failure has led to investigations of varying radiation volumes, which have guided the transition from whole brain radiation therapy to more regionally focused treatment plans with the goal of maximizing radiation delivery to tumor cells while limiting delivery to normal tissue. A randomized controlled trial reported no survival difference between patients receiving 6020 cGy of whole brain radiation and those receiving 4300 cGy of whole brain radiation plus 1720 cGy delivered to enhancing tumor volume plus a margin of 2 cm. The results of this trial have formed the standard regional delivery of radiation in patients with HGG, as opposed to whole brain radiation that was previously delivered. A recent RTOG phase I study used dose escalation with conformal radiation therapy ranging between 66 and 84 Gy and reported no increase in rates of radiation injury.
[Tags: Glioma, glioblastoma, radiation, whole brain radiation, radiotherapy, dose escalation, fractionation, hyperfractionation].
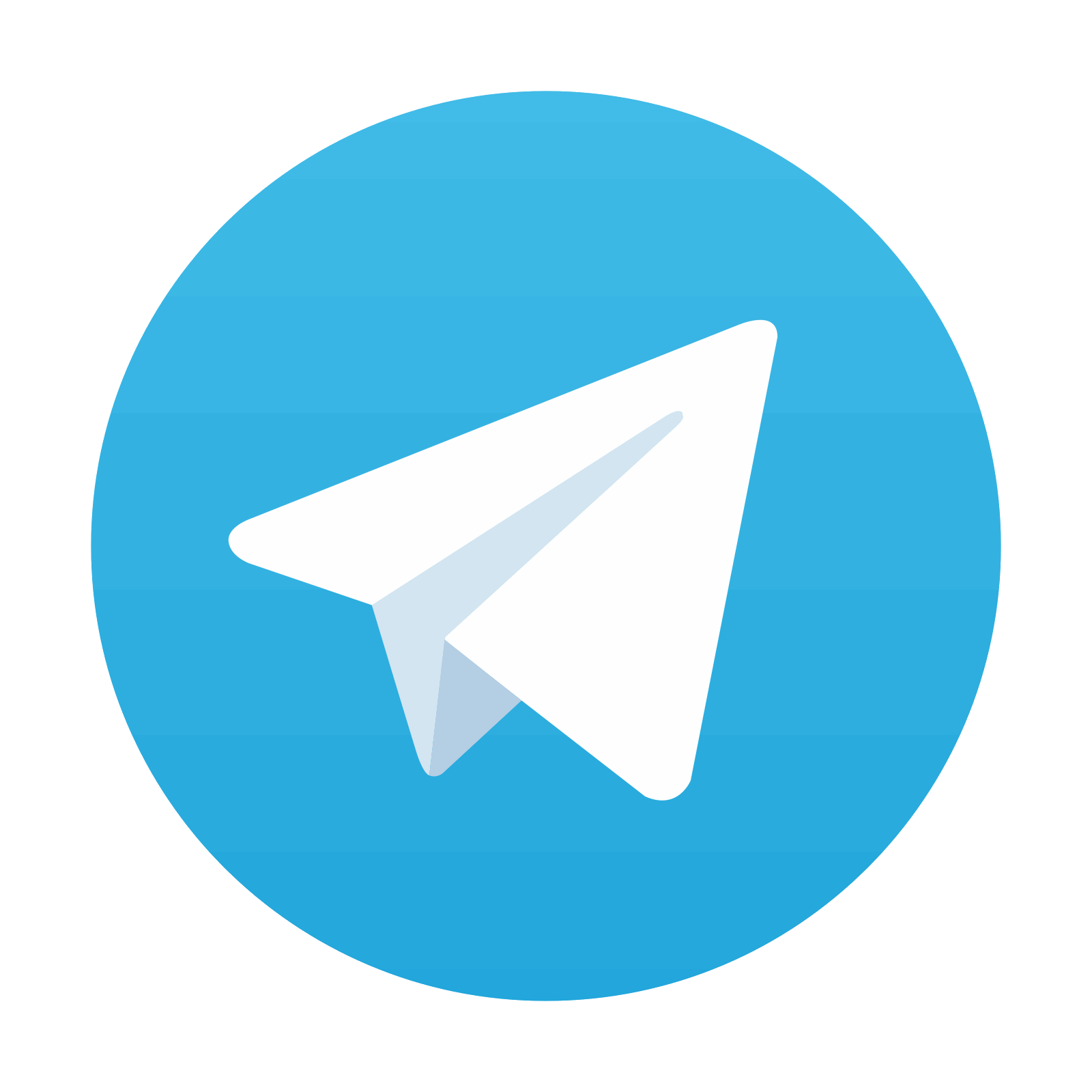
Stay updated, free articles. Join our Telegram channel

Full access? Get Clinical Tree
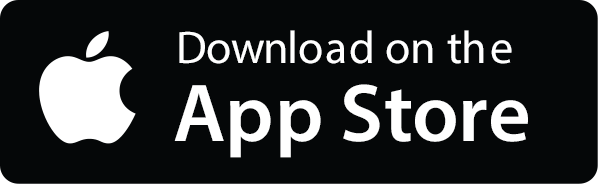
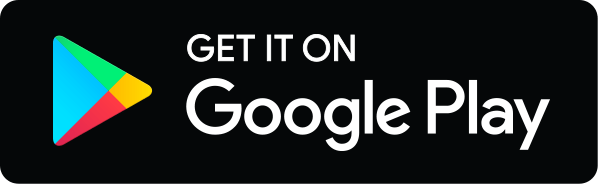