A 45-year-old man is admitted to the neurologic intensive care unit (NeuroICU) after resection of a craniopharyngioma. Three days into his ICU admission, he has a witnessed aspiration event with subsequent respiratory distress and hypoxemia requiring invasive mechanical ventilation. Over the next 24 hours, his hypoxemic respiratory failure worsens despite increasing mechanical ventilatory support, deep sedation, neuromuscular blockade, and a trial of prone positioning. His chest radiograph demonstrates diffuse bilateral infiltrates. With the patient receiving a fraction of inspired oxygen of 1.0, a positive end-expiratory pressure of 15 cm H2O, a tidal volume of 6 mL/kg of predicted body weight, and a respiratory rate of 35 breaths per minute, arterial blood gas analysis reveals a pH of 7.14, a PaCO2 of 70 mm Hg, and a PaO2 of 50 mm Hg. Plateau airway pressure, measured at end inspiration, is 37 cm H2O.
What is the role of venovenous extracorporeal membrane oxygenation (ECMO) in the management of the acute respiratory distress syndrome (ARDS)?
The ARDS is characterized by the rapid onset of hypoxemia and bilateral pulmonary infiltrates consistent with pulmonary edema that cannot be fully attributed to cardiac failure or fluid overload.1,2 The definition of ARDS was recently revised, using the ratio of the partial pressure of oxygen (PaO2) to the fraction of inspired oxygen (FIO2) to classify ARDS into mild (200 < PaO2/FIO2 ≤ 300 mm Hg), moderate (100 < PaO2/FIO2 ≤ 200 mm Hg), and severe (PaO2/FIO2 ≤ 100 mm Hg) forms, with a minimum positive end-expiratory pressure (PEEP) of 5 cm H2O. There is evidence that mortality correlates with severity of illness, with the lowest PaO2 to FIO2 ratios resulting in the highest mortality rates; however, this association requires further validation.2,3
Pathologically, ARDS is characterized by injury to the lung epithelium and capillary endothelium, resulting in increased permeability of protein-rich fluid.4,5 The resulting pulmonary edema and disruption of surfactant production and function lead to decreased lung compliance and impaired gas exchange.6 This lung injury may be further exacerbated by positive-pressure ventilation, so-called ventilator-associated lung injury (VALI), through the over-distention of less affected lung regions and the repeated opening and closing of alveoli and small bronchioles.7 The mainstay of management in ARDS is treatment of the underlying disease process and minimization of VALI while supplying adequate gas exchange support.5
There are few strategies that have been demonstrated to reduce mortality in ARDS. The most widely accepted approach to the management of ARDS is a lung-protective ventilatory strategy targeting low tidal volumes and plateau airway pressures. In a landmark trial by the ARDS Network, 861 patients with ARDS were randomized to a tidal volume of 4 to 6 mL/kg predicted body weight for a plateau airway pressure goal of ≤ 30 cm H2O or a tidal volume of 10 to 12 mL/kg predicted body weight for a plateau airway pressure goal of ≤ 50 cm H2O. Those in the low-volume, low-pressure group had a statistically significant reduction in mortality (31% vs 39.8%, P = 0.007), along with more ventilator-free days and days without nonpulmonary organ failure.8 These findings, in combination with results of other similarly designed trials, have resulted in the acceptance of a lung-protective ventilation strategy as standard of care in ARDS.9,10
Other strategies that have demonstrated a mortality benefit in ARDS include the use of neuromuscular-blocking agents (NMBAs) and prone positioning.11,12 In a prospective, multicenter double-blind, placebo-controlled trial, cisatracurium, implemented within the first 48 hours of ARDS onset in those with more severe forms of the syndrome (PaO2/FIO2 ≤ 120 mm Hg), was shown to reduce 90-day mortality compared with placebo (30.8% vs 44.6%, P = 0.04).11 Prone positioning was recently investigated in a multicenter, unblinded, randomized controlled trial of patients with ARDS with a PaO2/FIO2 ≤ 150 mm Hg.12 Twenty-eight day mortality was significantly lower in the prone group than the supine group (16.0% vs 32.8%; hazard ratio, 0.42; 95% confidence interval [CI], 0.26-0.66; P < 0.001), a difference that persisted at 90 days. Of note, all centers involved in the study had extensive experience with prone positioning. A subsequent meta-analysis demonstrated a consistent survival benefit in those studies where prone positioning was combined with low tidal volume ventilation, but not for those trials where low tidal volumes were not mandated. This reinforces the importance of a lung-protective ventilation strategy in ARDS.13
ECMO may be a complementary or alternative approach to the management of patients with severe ARDS. ECMO directly oxygenates and removes carbon dioxide from the blood via an extracorporeal gas exchange device, referred to as an oxygenator. The oxygenator consists of a semipermeable membrane that allows diffusion of gas between two compartments. Blood flows along one side of the membrane and sweep gas, which is typically composed of a blend of oxygen and ambient air, flows along the other side (Figure 40-1). In venovenous ECMO, deoxygenated blood is withdrawn from a central vein via an external pump and pushed through the oxygenator, with the oxygenated and decarboxylated blood reinfused into a central vein. ECMO should be considered when invasive mechanical ventilation alone is insufficient to support gas exchange.14 ECMO may also be considered when positive-pressure ventilation is able to provide adequate gas exchange, but only at the expense of excessively high airway pressures or when unacceptable levels of hypercapnia and acidosis limit the application of a lung-protective ventilation strategy.15
Figure 40-1.
Gas exchange in ECMO. Gas exchange in ECMO is accomplished by pumping blood through an oxygenator, consisting of two compartments divided by a semipermeable membrane. Venous blood passes through one compartment and fresh gas (referred to as sweep gas) passes through the other. Oxygen uptake and carbon dioxide removal occur across the membrane. The fraction of delivered oxygen is determined by a blender that typically mixes oxygen with room air.

Early randomized studies failed to show a benefit of ECMO on survival in severe forms of ARDS, in large part due to inexperience with its use and the high complication rates associated with older extracorporeal technology.16,17 ECMO circuits have since undergone significant advancements, including the development of centrifugal pumps, biocompatible circuit components, and more efficient oxygenator membranes, all of which have lessened the risks associated with ECMO.18-22 The impact of modern ECMO technology on survival in severe ARDS was studied in the Conventional Ventilation or ECMO for Severe Adult Respiratory Failure (CESAR) trial.23 One hundred eighty subjects with severe, potentially reversible respiratory failure were randomized to conventional mechanical ventilation or referral to a specialized center for consideration of ECMO. The group referred for consideration of ECMO had a significantly lower rate of death or severe disability at 6 months than the group that received conventional management (37% vs 53%; relative risk, 0.69; P = 0.03). However, it is important to note that, as part of the management protocol, only 76% of those referred for consideration of ECMO actually received ECMO. Beyond this, a notable flaw of the trial was that it did not mandate a lung-protective ventilation strategy in the control arm, and as a result, only 70% of the conventionally managed group received standard-of-care lung-protective ventilation at any time during the study. One may conclude that referral to a specialized, ECMO-capable center favorably affects disability-free survival. However, conclusions from this study about the impact of ECMO itself on survival in severe respiratory failure are limited.
Retrospective propensity analyses evaluating the impact of ECMO on survival in ARDS have shown conflicting results. A study out of the United Kingdom suggests a mortality benefit existed when patients were referred for ECMO in the context of severe influenza A(H1N1)-associated ARDS compared with a matched cohort in whom ECMO was not considered (24% vs 47%; relative risk, 0.51; 95% CI, 0.31-0.84; P = 0.008).24 However, a similarly designed propensity analysis of a French cohort did not demonstrate any mortality benefit from ECMO for severe influenza-associated ARDS compared with conventional management (odds ratio, 1.48; 95% CI, 0.68-3.23; P = 0.32).25 The inconclusiveness of these data prompted a randomized controlled trial comparing ECMO with standard of care management in patients with severe ARDS, which is currently underway (ECMO to Rescue Lung Injury in Severe ARDS [EOLIA]).26
There may be a benefit to reducing tidal volumes and plateau airway pressures beyond the currently accepted standard of care.27,28 This concept has been investigated in two recent studies, with ECMO-facilitated very low tidal volumes (approximately 3 to 4 mL/kg predicted body weight) resulting in a reduction in inflammatory cytokines associated with VALI and a greater number of ventilator-free days in patients with more severe forms of ARDS.29,30 Additional studies are needed to define the optimal ventilatory strategy for patients with severe ARDS who are receiving ECMO support and to determine whether ECMO-facilitated very low tidal volumes may offer a benefit in milder forms of ARDS.
There are no universally accepted criteria for the initiation of ECMO. However, potential indications that have been proposed include severe hypoxemia (PaO2/FIO2 < 80), uncompensated hypercapnia with acidemia (pH < 7.15), or excessive plateau airway pressures (> 35-45 cm H2O) despite optimal ventilator management (Table 40-1).1,31 Relative contraindications include prolonged exposure to high airway pressures and high fractions of inspired oxygen, difficulties in obtaining vascular access, extrapulmonary conditions that may limit the overall benefit of ECMO (including severe, irreversible neurologic injuries), and contraindications to anticoagulation, which is generally required to maintain circuit patency. Although there are no absolute contraindications to ECMO in ARDS, its use should not be offered in cases of irreversible, end-stage respiratory failure if lung transplantation will not be considered.
Indications Severe hypoxemia (eg, PaO2/FIO2 < 80) despite the application of high levels of PEEP (when tolerated), prone positioning (when available), and possibly neuromuscular blockade in patients with potentially reversible respiratory failure Uncompensated respiratory acidosis (pH < 7.15) despite optimal ventilator management Excessively high plateau airway pressures (> 35-45 cm H2O, depending on the patient’s body size) despite optimal ventilator management |
Relative Contraindications Prolonged exposure (> 7 days) to high airway pressures (plateau pressure > 30 cm H2O) or high amounts of oxygen (FIO2 > 0.8) Limitations in obtaining vascular access Any condition that would limit the overall benefit from ECMO, such as severe neurological injury or untreatable metastatic cancer Contraindication to anticoagulation |
Absolute Contraindications None |
ECMO is one of several so-called “salvage therapies” for patients with severe ARDS.
By directly oxygenating and removing carbon dioxide from the blood, ECMO may allow for less reliance on invasive mechanical ventilation to maintain adequate gas exchange, thereby facilitating lung-protective ventilatory strategies.
A prospective randomized controlled trial is underway to better define the role of ECMO in the management of severe ARDS.
The traditional configuration used for venovenous ECMO involves cannulation at two distinct venous access points, with a drainage cannula in the inferior vena cava via a femoral vein and a reinfusion cannula in the superior vena cava via an internal jugular vein (Figure 40-2).14 This cannulation strategy does not require image guidance (although ultrasound-guided vascular access is recommended) and may be performed rapidly at the bedside. However, because of the orientation of the drainage and reinfusion ports, this strategy lends itself to recirculation, a phenomenon in which reinfused oxygenated blood is drawn back into the circuit through the drainage cannula without having passed through the systemic circulation. Because recirculation does not contribute to systemic oxygenation, it decreases overall ECMO efficiency. Another drawback to a two-site cannulation strategy is the need for femoral access, which may complicate patient mobility if ambulation is anticipated. An alternative strategy became available with the development of the bicaval, dual-lumen cannula.32,33 The dual-lumen cannula permits venovenous ECMO via a single vascular access point, obviating the need for femoral access (Figure 40-3). It also has the potential to reduce the rate of recirculation when properly positioned.32 Placement should be performed by those experienced in its use, ideally under transesophageal echocardiographic and fluoroscopic guidance.34 The choice of cannula size depends on the physiologic needs of the patient, with particular consideration for the estimated cardiac output of the patient.14 In general, larger cannulas will allow for higher ECMO blood flow rates to be achieved, although placement of larger cannulae may be limited by the patient’s body habitus and size of the patient’s veins. The size of the drainage cannula is most important for achieving high flow rates.
Figure 40-2.
Two-site venovenous ECMO. In venovenous ECMO, blood is drained from a central vein, pumped through an oxygenator, and reinfused into a central vein. Venovenous ECMO provides gas exchange support only, without providing any hemodynamic support. Inset: When drainage and reinfusion ports are in close proximity, some reinfused blood may be drawn back into the circuit without having passed through the systemic circulation, referred to as recirculation (purple arrow).

Venovenous ECMO may be performed with single-site or two-site cannulation.
Single-site cannulation with a jugular, bicaval, dual-lumen cannula may reduce the amount of recirculation within the circuit, but it requires imaging guidance to ensure proper placement.
What are the recommended management strategies for patients receiving ECMO for respiratory failure?
Ventilation, anticoagulation, transfusion, and sedation practices vary widely between ECMO centers, without any universally accepted guidelines. The concern over the implication of VALI on outcomes in ARDS has led many ECMO centers to adopt a very lung-protective ventilation strategy, with reduction of tidal volumes to achieve the lowest possible plateau airway pressures, while maintaining a moderate amount of PEEP to sustain alveolar patency and minimize atelectasis. This strategy has yet to be studied in a controlled trial. With the potential for ECMO to sufficiently support gas exchange on its own, there may be the possibility for removal of invasive mechanical ventilation altogether.35 Discontinuation of invasive mechanical ventilation would definitively eliminate the risk of VALI. However, the effect of spontaneous breathing on lung injury in ARDS requires further study.36-38
Anticoagulation strategies in ECMO have been evolving concurrently with advances in extracorporeal technology, particularly with the development of increasingly biocompatible circuit components. Historically, ECMO required high levels of systemic anticoagulation in order to prevent the development of thromboses within the circuit that result from activation of platelets and the clotting system by the foreign material.39 However, with newer materials, ECMO circuits are less prone to thrombosis, allowing for more modest levels of anticoagulation. Many centers have adopted a strategy targeting low-level anticoagulation, in the range of activated partial thromboplastin times of 40 to 60 seconds.22 However, both the parameters used to gauge anticoagulation levels and the target levels themselves are not universally agreed upon and vary from center to center.40 In patients with neurologic conditions that may have substantial risk of intracerebral hemorrhage, there must be a careful consideration of the risks and benefits of ECMO and the accompanying need for systemic anticoagulation.
Transfusion thresholds likewise vary greatly between ECMO centers. Because the ability of ECMO to deliver oxygen is dependent, in part, on the oxygen-carrying capacity of the blood, normal hemoglobin levels have traditionally been sought to maximize oxygen transfer and ECMO efficacy.41 However, achieving normal hemoglobin levels may require as many as 2 to 3 transfusions of packed red blood cells per day,42-45 which has been associated with increased morbidity and mortality in critically ill patients.46-50 Therefore, a more restrictive transfusion strategy, targeting hemoglobin levels traditionally accepted amongst the general critical care population, may be reasonable in patients receiving ECMO. However, because of the lower oxygen-carrying capacity of blood that results from lower hemoglobin levels, arterial oxygen saturation goals may need to be adjusted to ensure adequate oxygen delivery to the tissues.
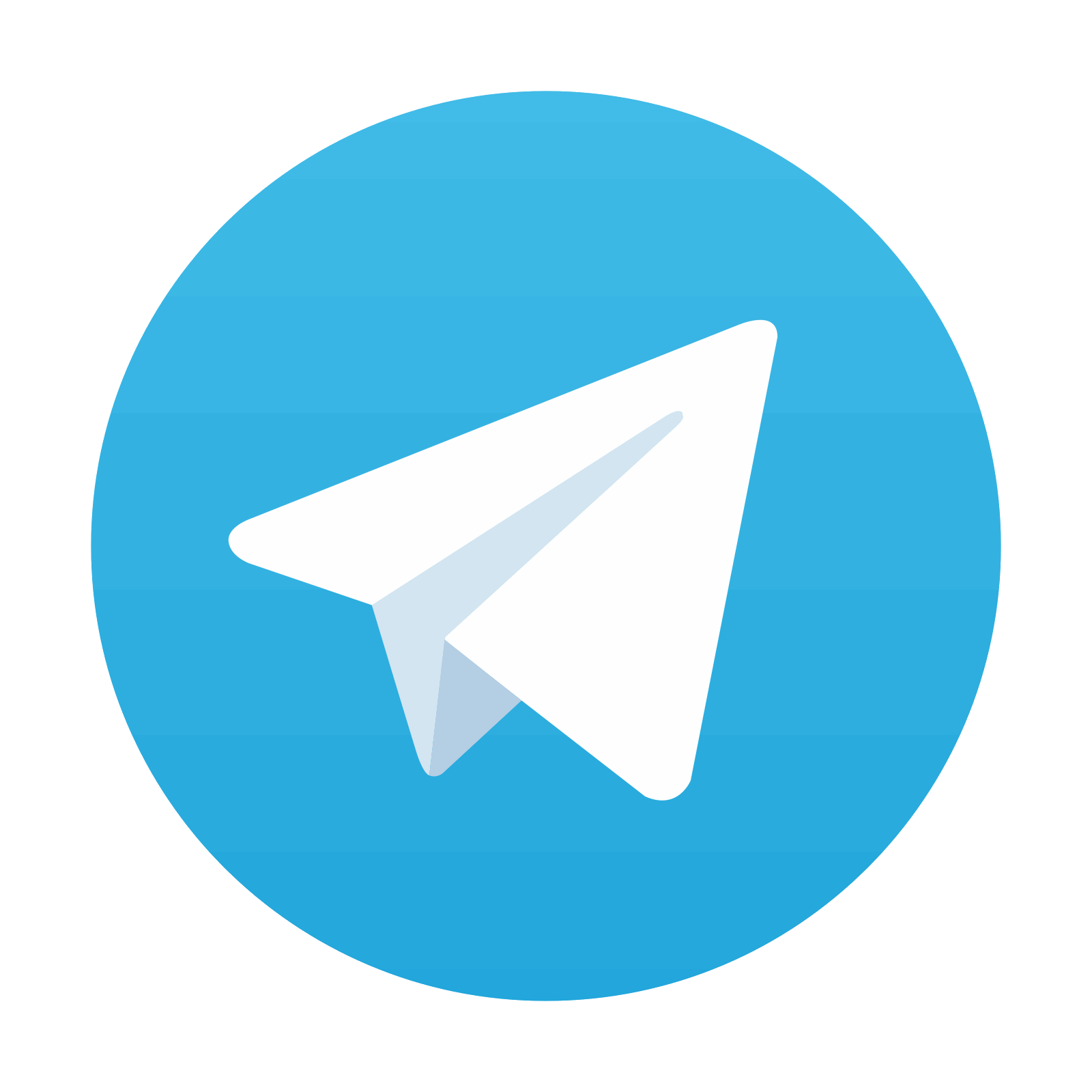
Stay updated, free articles. Join our Telegram channel

Full access? Get Clinical Tree
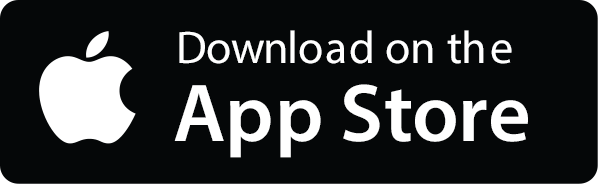
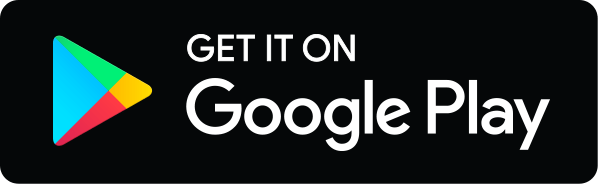