Fig. 6.1
Micrograph of an early outgrowth colony forming unit—endothelial cell (CFU-EC) isolated from an ischemic stroke patient in a phase-contrast microscope (×100). A CFU-EC consisted of a central cluster of rounded cells with elongated sprouting cells at the periphery
6.3.2 Endogenous Stimulation of EPCs
Restoring blood flow supply after ischemia and re-endothelization after hemorrhage may contribute to cell survival and tissue repair. Formation of new blood vessels in the adult brain after stroke is not only mediated by angiogenesis but also involves vasculogenesis mediated by EPCs, which are involved in processes of re-endothelization and repair of vascular endothelium in response to vascular trauma or tissue ischemia, promoted by biochemical factors that activate its proliferation . They have been described that EPCs migrate through the peripheral blood from bone marrow to sites of neovascularization where EPCs are able to differentiate into mature endothelial cells. Recruitment and incorporation of EPCs into ischemic or hemorrhagic tissues requires a coordinated multistep process including mobilization, chemoattraction, adhesion, migration , tissue invasion and in situ differentiation (Fig. 6.2) (Rodríguez-González et al. 2007). Many molecular and physiological-pathological factors, as well as drugs, are involved in these processes (For review: Arenillas et al. 2007; Brea et al. 2009; Sobrino et al. 2011a, b, 2012a, b; Zhao et al. 2013) (Table 6.1). For example, the activity of matrix metalloproteinase 9 (MMP-9), which causes a massive release of stem cell factor (SCF) and activation of membrane bound Kit ligand (mKitL) that favors the recruitment of progenitor cells, among which are EPC, from bone marrow. Likewise, active MMP-9 induces the release of cytokines that cause the mobilization of quiescent EPC (Rafii et al. 2002). Moreover, EPC release and mobilization are regulated by vascular endothelial growth factor (VEGF), stromal-cell-derived factor 1 (SDF-1), granulocyte colony-stimulating factor (G-CSF), erythropoietin (EPO), angiopoietin 1, endothelial NO synthase (eNOS), exercise, estrogens and several drugs such as statins, EPO or citicoline. In fact, clinical studies in IS and ICH patients demonstrated that serum levels of VEGF, SDF-1α and active MMP-9 increase in response to cerebral ischemia or ICH within the first 72 h from symptom onset, and that the magnitude of this increase is directly related to an EPC increment (Bogoslovsky et al. 2011a, b; Sobrino et al. 2011a, 2012b). On the other hand, the fact that serum levels of molecular markers at 24 h from stroke onset correlated with EPC increment during the 1st week, but not at admission, and that EPC increment during the 1st week, but not EPC counts at baseline, has been associated with better neurological outcome and reduced infarct growth supports the hypothesis that cerebral ischemia induces the activation of molecular pathways of EPC mobilization focused on promoting endogenous processes of vascular and neurorepair (Fig. 6.3). Furthermore, similar results were found in ICH patients (Sobrino et al. 2009, 2011a; Paczkowska et al. 2013). It has been reported in ICH patients a strong correlation between VEGF and SDF-1α serum levels and circulating concentrations of bone marrow-derived progenitor cells (BMPCs) at day 7 (Sobrino et al. 2011a). Given that the EPC is a subtype of BMPCs, it is tempting to hypothesize that similar molecular and cellular mechanisms are involved in the two major subtypes of stroke (ischemic and hemorrhagic stroke).
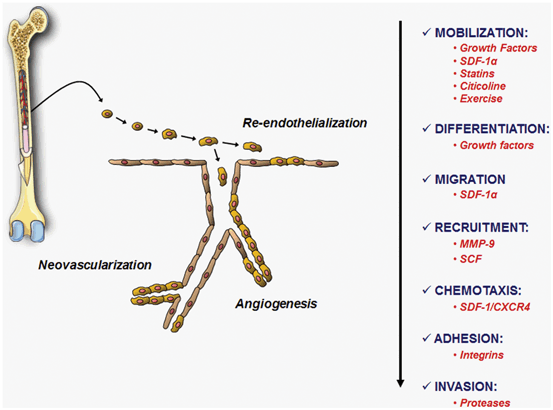
Fig. 6.2
Factors involved in the mobilization, differentiation, migration, recruitment, chemotaxis, adhesion and invasion of EPCs in stroke. These mechanisms are necessary in order that EPCs exert their beneficial functions such as re-endothelialization, angiogenesis and neovascularization
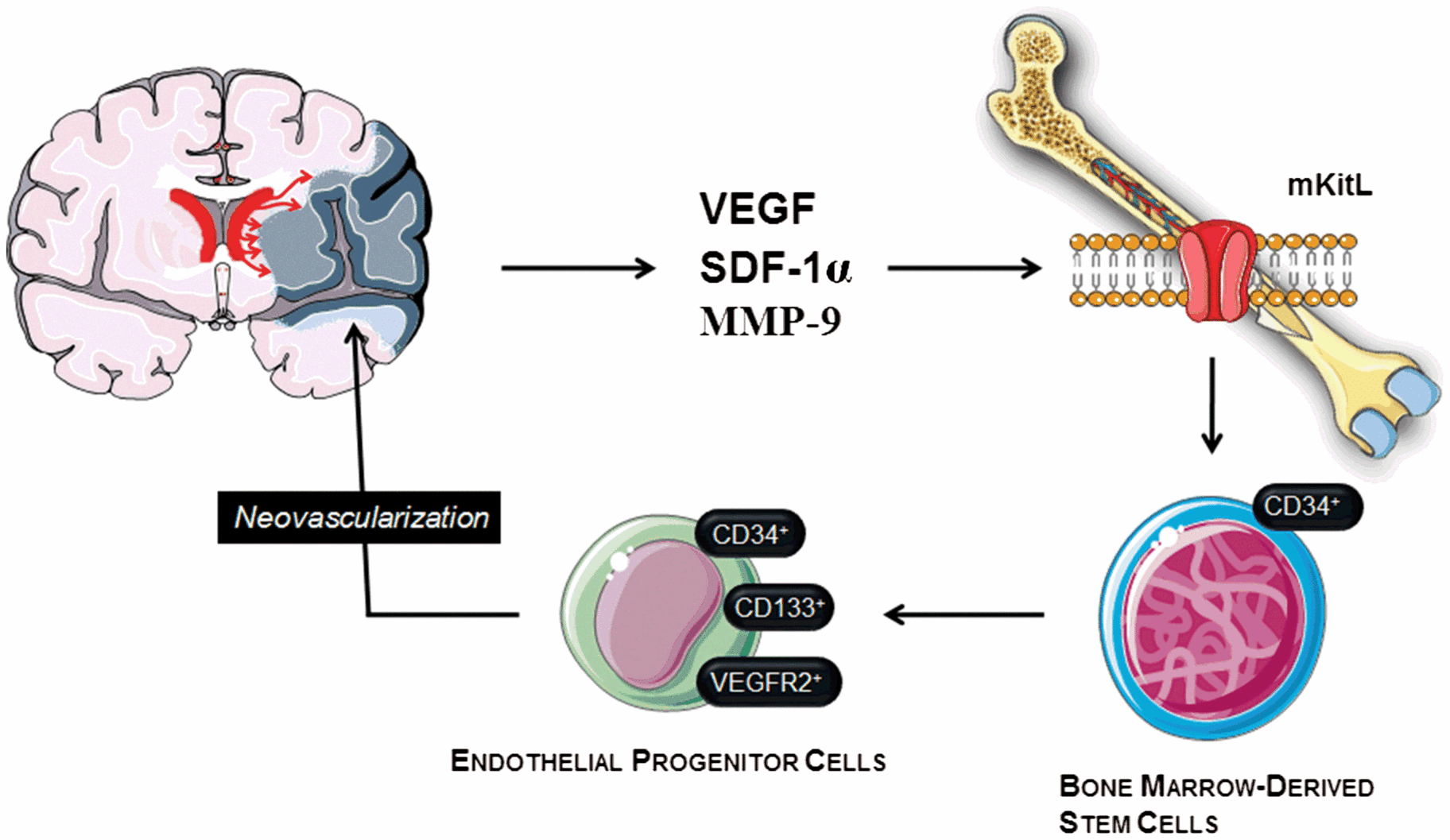
Fig. 6.3
Mechanism of endothelial progenitor cells (EPC) mobilization in stroke. Stroke is a potent inducer of endogenous repair mechanisms, which are initially activated by a massive expression of growth factors such as vascular endothelial growth factor (VEGF), and other molecules such as matrix metalloproteinase 9 (MMP-9) and stromal cell-derived factor 1α (SDF-1α). These molecular factors, especially MMP-9 activity, induce the mobilization of bone marrow-derived stem cells, including EPCs, through membrane bound Kit ligand (mKitL) activation. These EPCs may migrate into the areas of brain injury, mediating repair mechanisms. Likewise, these mechanisms also enhance the endogenous neurogenesis
Release and/or Mobilization | |
---|---|
Chemokines/growth factors | |
Hypoxia-inducible factor 1 (HIF-1) | > |
Stromal cell derived SDF-1 | > |
Vascular endothelial growth factor (VEGF) | > |
Insulin-like growth factor 1 (IGF-1) | > |
Granulocyte-colony stimulating factor (G-CSF) | > |
Angiopoietin 2 | > |
Drugs | |
Statins | > |
Angiotensin II type 1 receptor blockers | > |
Angiotensin-converting enzyme inhibitor | > |
Erythropoietin (EPO) | > |
Berberine | > |
Morphine | < |
Citicoline | > |
Recombinant tissue plasminogen activator (r-tPA) | > |
PPAR-γ agonist | > |
Proteins/hormones | |
Estrogens | > |
Nitric oxide (NO) and eNOS (endothelial NO Synthase) | > |
Aldosterone | < |
Angiotensin II | < |
Endostatin | < |
Heme-oxigenase 1 | > |
Matrix metalloproteinase 9 (MMP-9) | > |
Physiological/pathological factors | |
Exercise and physical training | > |
Wound | > |
Ischemic events | > |
Aging | < |
Obesity | < |
Smoking | < |
Hypertension | < |
Diabetes | < |
Hypercholesterolemia | < |
Homocysteine | < |
Recruitment | |
Chemokines/growth factors | |
SDF-1/CXCR4 | |
CCL5/CCR5 | |
CXCL1 | |
CXCL7/CXCR2 | |
VEGF/VEGFR2 | |
IL-8/Gro | |
CCL5/CCR5 | |
IGF2/IGFR2 | |
Other molecular factors | |
Caspase-8 | |
Hyaluronic acid | |
Thrombin | |
CD9 | |
Alpha6 integrin subunit |
On the other hand, because EPCs express functional CXCR4 and VEGF receptors (Salcedo et al. 1999; Yamaguchi et al. 2003), an interaction between the SDF-1α/CXCR4 pathway and VEGF might form a positive-feedback loop which would increase the therapeutic effects of EPCs in cerebral neovascularization. Consequently, growth factors and SDF-1α might be an effective therapy in IS and ICH because of their role mediating the mobilization of circulating EPCs, as well as in restoring endothelium integrity and decreasing brain edema, inflammation , and perihematoma cell death (Krizanac-Bengez et al. 2004).
As a clinical implication, the number of circulating EPCs is inversely correlated with vascular risk factors such as diabetes, hypertension, hypercholesterolemia or smoking (Hill et al. 2003; Vasa et al. 2001; Werner et al. 2005), and also with molecular markers of endothelial dysfunction and inflammation such as homocysteine or C-reactive protein. Therefore, it can be rationally speculated that environment of circulation is essential for the living and functionality of EPCs, which would raise the perspective on the demand in managing risk factors of stroke (Zhao et al. 2013).
Finally, it has also been demonstrated that several drugs can modulate endogenous EPC behavior (Table 6.1). Statins treatment during acute phase leads to an increase in EPCs in IS patients (Sobrino et al. 2012a; Martí-Fàbregas et al. 2013). In view of the fact that statin treatment during the acute phase increases circulating EPC and statin withdrawal is associated with poor outcome in IS patients (Blanco et al. 2007), the positive effects of statin treatment during the acute phase on functional outcome in ischemic stroke could be mediated by EPC. Moreover, patients treated with statins showed also higher serum levels of VEGF, active MMP-9 and nitric oxide (NO)x at 24 h (Sobrino et al. 2012a). Statins induce the production of NO by eNOS, the expression of angiogenic factors such as VEGF, and the mobilization and proliferation of EPC (Endres 2005) , so these mechanisms may be interrelated. G-CSF is one of the early drugs discovered to be able to enhance EPC mobilization into the circulation after venous administration (Powell et al. 2005). Afterwards, other drugs such as Angiotensin II type 1 receptors blocker, Angiotensin-converting enzyme inhibitors, EPO, berberine, citicoline, recombinant tissue plasminogen activator (r-tPA) and PPAR-γ agonist have been shown to increase the number and functional activity of EPCs in vitro and in vivo (Arenillas et al. 2007; Rodríguez-González et al. 2007; Sobrino et al. 2011b, 2012a, b; Zhao et al. 2013). As these drugs are commonly used in clinical treatment of vascular diseases, all these clinical data may help to interpret the beneficial effects of these drugs on top of their known pharmacological actions. However, further studies are needed in order to facilitate the discovery of new drugs targeting EPCs.
6.4 Promising Strategies Related to EPCs
As a promising strategy for cellular-based therapies for stroke , induced pluripotent stem cells (iPSC) technology (Takahashi and Yamanaka 2006; Takahashi et al. 2007), which enables the reprogramming of a wide variety of cell types isolated from humans into embryonic stem cell-like pluripotent cells, offers a novel strategy for the patient-specific derivation of a lineage-specific cells from iPSC, such as EPCs (Choi et al. 2009; Park et al. 2010; Xu et al. 2012; Yoo et al. 2013). Moreover, the therapeutic potential of transplanted human iPSC-derived EPCs (hiPSC-EPCs) has been shown in animal disease models of hind-limb ischemia (Park et al. 2010; Rufaihah et al. 2011; Lai et al. 2013). Therefore, it is tempting to postulate that hiPSC-EPCs may represent a strategy for patient-specific EPC therapies in stroke.
Moreover, new strategies are necessary in order to increase the local number of EPCs in the ischemic or hemorrhagic areas. In this regard, nanomedicine may be useful to achieve this goal. A recent study has demonstrated the potential role of superparamagnetic iron oxide nanoparticles (SPION)-loaded EPCs by using a magnetic guidance to the ischemic tissue in animal models of cerebral ischemia . The authors demonstrate ex vivo cellular viability and maintained function following SPION load as well as successful guidance of the EPCs to the target site via magnetic resonance imaging (MRI) (Carenza et al. 2014). On the other hand, another recent study from our group (Agulla et al. 2014) has report a new theranostic nano-platform vectorized towards peri-infarct tissue, the key target for the treatment of cerebral ischemia . Anti-HSP72 (72 kDa heat shock protein) stealth immunoliposomes containing MRI probes were used to allocate the peri-infarct region in vivo and to achieve a superior therapeutic effect in comparison to other non-targeted drug delivery means (Fig. 6.4). Thus, despite the challenge of crossing the blood-brain barrier, this study demonstrates that theranostics inside the brain parenchyma is feasible and represents a good example of the potential that nanotechnology offers for the treatment of neurological disorders such as stroke . In this regard, these anti-HSP72 immunoliposomes, containing in their membrane one or more of the proteins involved in EPC recruitment shown in Table 6.1, could be used to locally increase the number of EPCs in the ischemic area after their exogenous transplantation or endogenous stimulation. Finally, another strategy in order to increase the local number of EPCs may be offered through interventional therapy for stroke, such as percutaneous transluminal angioplasty and stenting (PTAS) and thrombectomy. In addition, application of a bio-engineered EPC-capture stent, which accelerates re-endothelialization and reduces thrombogenicity, may reduce the rate of restenosis after PTAS (Larsen et al. 2012).
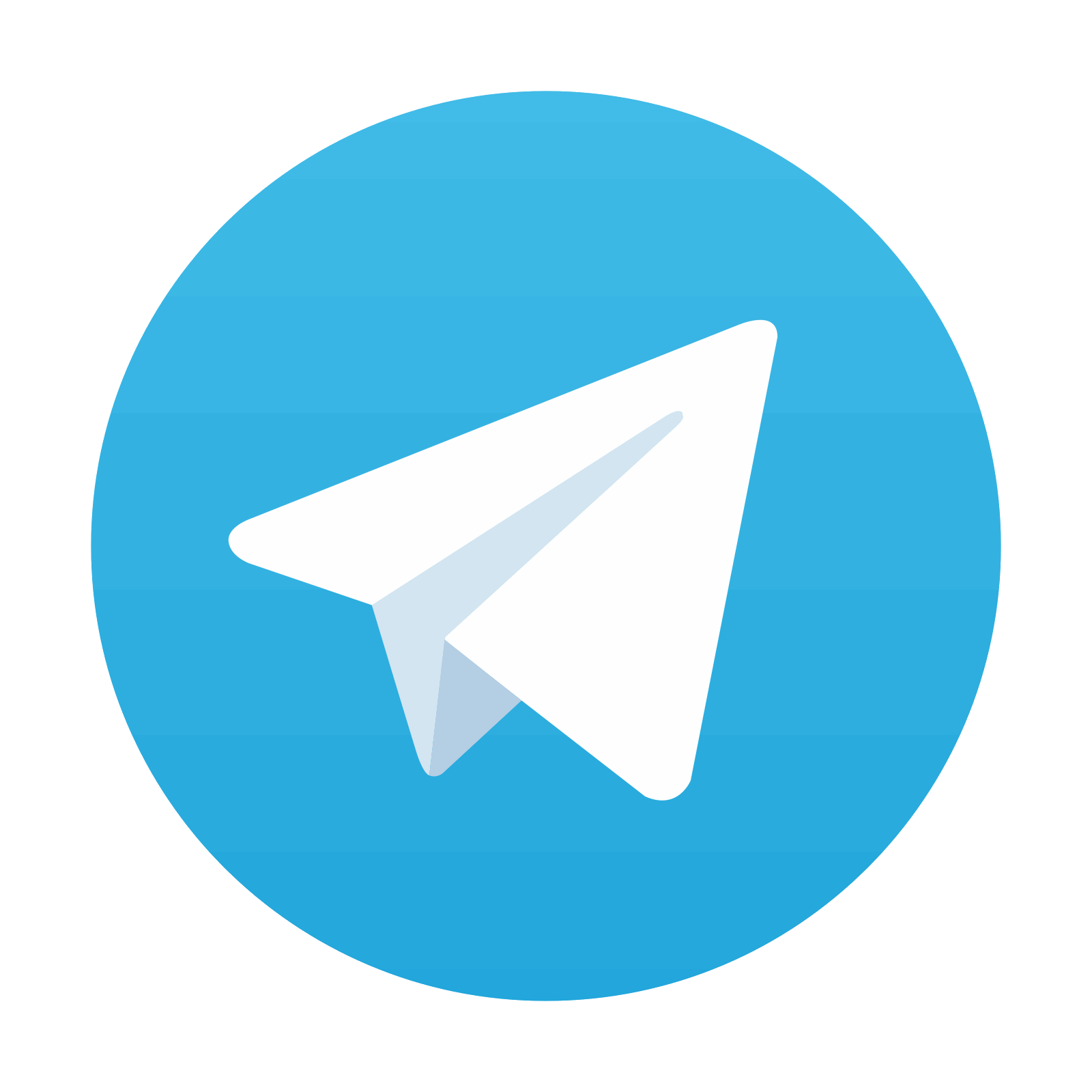
Stay updated, free articles. Join our Telegram channel

Full access? Get Clinical Tree
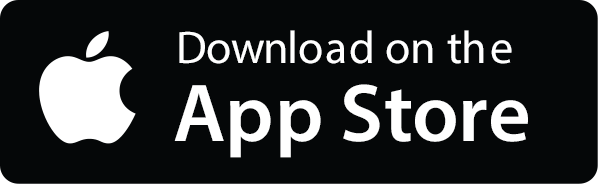
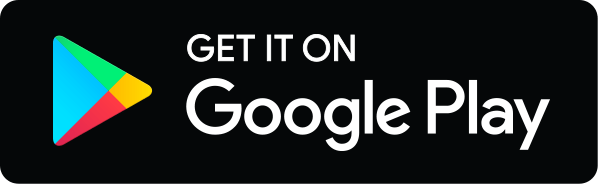