Fig. 24.1
General schematic of cellular GSH biosynthesis, utilization, export, and salvage. Glutathione (GSH) biosynthesis requires two ATP-dependent reactions: the glutamate cysteine ligase (GCL)-mediated formation of γ-glutamylcysteine (γGC) from glutamate (Glu) and cysteine (Cys) and the glutathione synthetase (GS)-mediated formation of GSH from γGC and glycine (Gly). GSH functions as a feedback inhibitor of GCL, but not GS. Cellular peroxides are detoxified through the actions of GSH peroxidase (GPx) resulting in the formation of oxidized GSH (GSSG) that can be salvaged by GSH reductase (GR). GSH also serves as a cofactor in glutathione-S-transferase (GST)-mediated detoxification of xenobiotics resulting in the formation of GSH conjugates (GS-Drug). Multidrug resistance protein (MRP) transporters are capable of effluxing GSH, GSSG, and GS-Drug conjugates into the extracellular space
Although maintaining cellular redox homeostasis is critical to protect against oxidative damage, GSH is also closely involved in the detoxification of a number of xenobiotic compounds and toxicants. The family of glutathione-S-transferase (GST) enzymes employs GSH as a co-factor in the Phase II metabolism of a number of chemotherapeutics, resulting in the formation of a covalent linkage between GSH and the drug (Fig. 24.1). GST-mediated GSH conjugation usually leads to the inactivation of the parent compound, while enhancing its water solubility and facilitating the transporter-dependent efflux of the GSH-conjugate from the cell (Townsend and Tew 2003). This leads to the depletion of cellular GSH stores. Cells are unable to effectively uptake intact extracellular GSH so replenishment of cellular GSH requires de novo GSH biosynthesis.
GSH is synthesized via two successive ATP-dependent reactions catalyzed by glutamate cysteine ligase (GCL) and glutathione synthetase (GS). GCL catalyzes the first and rate-limiting step in the process: the formation of γ-glutamyl cysteine (γ-GC) from glutamate and cysteine (Fig. 24.1). GCL is a heterodimeric holoenzyme complex consisting of two subunits. The catalytic subunit (GCLC) contributes all of the enzymatic activity and contains all of the substrate and cofactor binding sites. The modifier subunit (GCLM) possesses no enzymatic activity alone, but formation of GCL holoenzyme by heterodimerization of the two subunits dramatically increases GCL specific activity and affinity for glutamate and ATP while simultaneously lowering affinity for GSH, which functions as a feedback inhibitor of GCL (Franklin et al. 2009). Although GS expression levels and activity do play a role in GSH biosynthesis, GCL enzymatic activity generally dictates cellular GSH levels and GSH biosynthetic capacity. The major determinants of cellular GCL activity are the relative expression of the GCL subunits, availability of the amino acid substrates (particularly cysteine), and the degree of negative feedback inhibition by GSH (Franklin et al. 2009).
The GSH System in the Brain
The brain is composed of a diverse panoply of morphologically and functionally distinct cell populations, constituting a rather unique model of cell-cell interaction. Two major cell types, neuronal and glial cells, are present in the brain. Neurons are the electrically excitable cells responsible for the generation of action potentials that constitute the mechanism by which the central nervous system (CNS) signals to the rest of the body. Glial cells include oligodendrocytes, which are responsible for the formation and maintenance of the myelin sheath insulating neuronal axons, and astrocytes, which provide structural and metabolic support to neurons. Astrocytes play a critical role in maintaining neuronal GSH levels by providing neurons with lactate for ATP production, cysteine for GSH synthesis, and assist with the removal of glutamate from the synaptic cleft of glutamatergic neurons (Anderson and Swanson 2000). The inability of most cell types to effectively import intact GSH underscores the importance of substrate availability and uptake, as well as de novo GSH biosynthesis, to the maintenance of neuronal GSH homeostasis. Substrate availability is a major determinant of GSH content in the brain and cysteine is typically the rate-limiting substrate for neuronal GSH biosynthesis (Dringen and Hirrlinger 2003). The majority of the cysteine utilized for neuronal GSH synthesis is derived from GSH that is exported from astrocytes and broken down into its constituent amino acids (Fig. 24.2) (Dringen and Hirrlinger 2003). The γ-glutamyl moiety is released by γ-glutamyltranspeptidase (γGT) activity and cysteine and glycine are released from the remnant CysGly dipeptide by the actions of membrane-bound neuronal dipeptidases (Dringen and Hirrlinger 2003). The Na+-dependent excitatory amino acid transporter (EAAT) family is responsible for the majority of cysteine uptake, with glial cells expressing EAAT1 and EAAT2 isoforms and neurons expressing EAAT 3 and EAAT4 (Anderson and Swanson 2000). Cysteine is rapidly oxidized in the extracellular environment to the dipeptide cystine so the majority of dietary cysteine is packaged into GSH by the liver for systemic transport (Lu 2009). Interestingly, while neuronal cells utilize cysteine for GSH biosynthesis, glial cells have the capacity to use either cysteine or cystine, which is imported via the Na+-independent glutamate-cysteine antiporter (Xc −). Although cysteine can also be derived from methionine via the trans-sulfuration pathway for astrocytic GSH synthesis, it does not appear to be a major source of cysteine for neuronal GSH biosynthesis (McBean 2012).
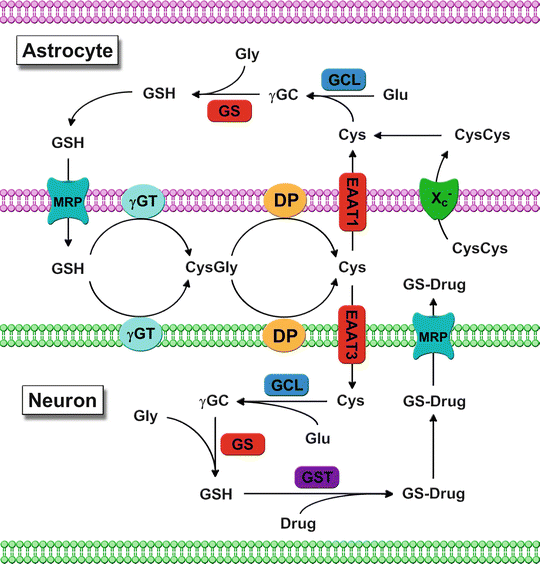
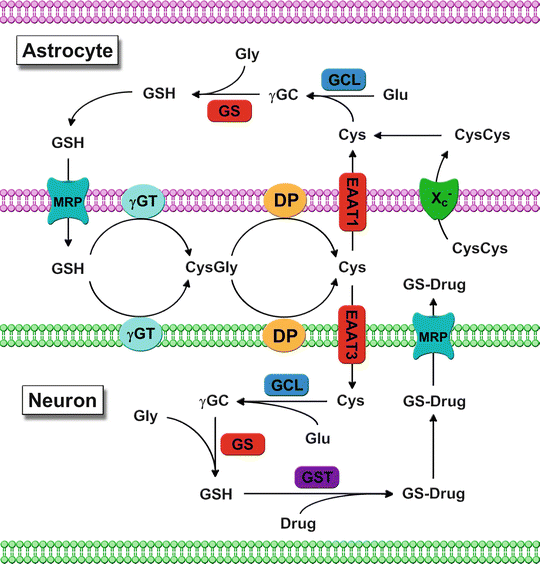
Fig. 24.2
Schematic of GSH-mediated interactions between astrocytes and neurons. Multidrug resistance protein (MRP)-mediated GSH efflux into the extracellular space by astrocytes is processed by γ-glutamyl transpeptidase (γGT) into glutamate (Glu) and cysteinylglycine (CysGly). CysGly is further hydrolyzed by cellular dipeptidases (DP) followed by excitatory amino acid transporter (EAAT)-mediated uptake of the constituent amino acids that can serve as substrates for either protein synthesis or cellular GSH production via the glutamate cysteine ligase (GCL)-catalyzed formation of γ-glutamylcysteine (γGC) from cysteine (Cys) and Glu and the glutathione synthetase (GS)-catalyzed formation of GSH from γGC and glycine (Gly). GSH is utilized as a cofactor in the glutathione-S-transferase (GST)-mediated formation of GSH-drug conjugates (GS-Drug) that are removed from the cell via MRP-mediated efflux. Additional Cys for GSH biosynthesis in astrocytes may also be obtained via the glutamate-cystine antiporter (Xc −)-mediated uptake of extracellular cystine (CysCys) which is rapidly reduced to Cys within the intracellular compartment
GSH efflux from astrocytes provides cysteine for neuronal GSH biosynthesis and the export of GSH conjugates from neurons and astrocytes is critical in the ultimate removal of conjugated cytotoxic xenobiotics. The multidrug resistance-associated proteins (MRPs) mediate the ATP-dependent export of GSH, GSSG, and GSH conjugates from the cell (Dallas et al. 2006). MRP-mediated efflux of GSH-drug conjugates is thought to play an important role in the development of resistance to various chemotherapeutics in most human CNS tumors (Bredel and Zentner 2002), with MRP overexpression directly correlating with drug resistance in gliomas and inversely with clinical outcome in neuroblastoma (Backos et al. 2012).
The expulsion of GSH-drug conjugates can lead to the depletion of intracellular GSH and a disruption of the GSH:GSSG ratio and cellular antioxidant capacity. These conditions promote the inducible expression of enzymes involved in the de novo GSH synthesis and GSH salvage pathways. The GCL subunits are regulated at the transcriptional, post-transcriptional, and post-translational levels in response to oxidative stress (Backos et al. 2011; Franklin et al. 2009), with the Nrf1/2, AP-1, and NF-κB transcription factors regulating the inducible and constitutive expression of both subunits (Lu 2009). The Nrf2 transcription factor mediates the induction of multiple cytoprotective enzymes involved in GSH biosynthesis, utilization, and export, including GCLC, GCLM, GR, γGT, GPx, GSTs, and MRPs (Lu 2009). Interestingly, Nrf2-dependent upregulation of glial cell GSH biosynthesis is both necessary and sufficient to protect neurons from oxidative stress by providing cysteine for neuronal GSH biosynthesis. Nrf2 activation may also coordinate astrocyte release of GSH and neuronal GSH biosynthesis via transcriptional upregulation of astrocyte GCL and neuronal EAAT3 expression, respectively (Escartin et al. 2011). Although the GCL subunits are often coordinately induced, enhanced GCLM expression has the potential to contribute more significantly to the inducible increase in GCL activity due to the massive increase in activity resulting from heterodimerization with GCLC. However, both GCL subunits are essential for the viability of cultured primary cortical neurons and overexpression of GCLC protects against glutamate- and NO-induced apoptosis (Diaz-Hernandez et al. 2005). Like other GSH metabolic enzymes, the GCL subunits are overexpressed in many tumor cell types and may play a central role in the development of chemoresistance (Lu 2009).
Human Brain Tumors
Tumors of the brain and CNS are among some of the fastest growing, most drug resistant, and most lethal human cancers. In contrast to most other human cancers, malignant brain tumors do not distally metastasize to other organs or tissues, generally remaining limited to the brain and CNS. The blood–brain barrier (BBB) is an additional hurdle unique to brain tumors which limits the number of chemotherapeutic options to those capable of significant partitioning into the neural compartment. Most studies from the literature examining drug resistance in CNS neoplasms have focused on those tumors with the highest incidence: gliomas in adults and medulloblastomas in children. Consequently, the discussion will focus mainly on these tumor types.
Gliomas
Malignant gliomas, including glioblastoma (GBM), anaplastic astrocytoma, and anaplastic oligodendroglioma, are the most common type of CNS malignancy in adults and comprise approximately 35 % of the total number CNS tumors diagnosed in the United States (Kohler et al. 2011). Standard of care for malignant gliomas usually involves surgical resection followed by radiation and concomitant chemotherapy (Stupp et al. 2009). However, in spite of these intensive therapies, the prognosis for patients diagnosed with malignant glioma is poor. The overall 5-year survival for adults ranges from about 30 % for anaplastic astrocytoma to about 8 % for GBM, while the median survival time after GBM diagnosis is typically 12–14 months, or <3 months without treatment (Kohler et al. 2011). Tumor relapse after an initially successful course of treatment is a common occurrence in gliomas and typically results in greater overall tumor resistance to subsequent therapy. Nullification of this resistance often requires intensifying the chemotherapeutic dosage regimen or incorporating adjuvant and/or experimental therapeutics. Both intrinsic and acquired drug resistance mechanisms have been implicated in the poor response of both primary and recurrent glial tumors to chemotherapy (Bredel and Zentner 2002).
The originating cell type of a brain tumor generally has a substantial impact on the behavior and relative susceptibility of tumors to chemotherapy. GBM and anaplastic astrocytoma are thought to arise from mature astrocytes or from astrocyte and/or glial cell precursors (Fig. 24.3) (Huse and Holland 2010). Astrocytes possess significantly higher levels of GSH compared to neurons and, in keeping with their role in protecting neurons from both oxidative damage and xenobiotic toxicity, astrocytes have a robust GSH biosynthetic and efflux capacity (Dringen and Hirrlinger 2003). Xenobiotic detoxification enzymes are also enriched in astrocytes as these cells are involved in the initial uptake and metabolism of compounds capable of crossing the blood–brain barrier. This characteristic cellular GSH machinery is likely to survive neoplastic transformation and may play a role in the inherent resistance of GBM and astrocytomas to chemotherapy and radiation (Backos et al. 2012).
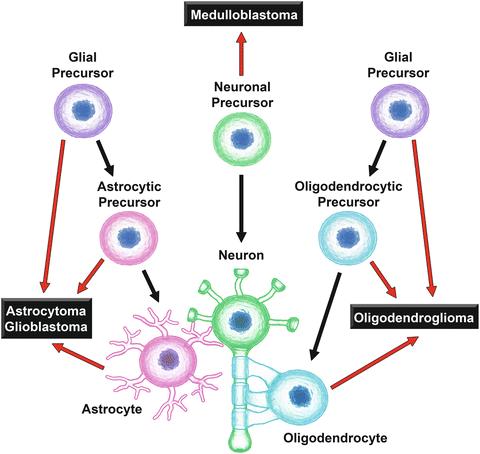
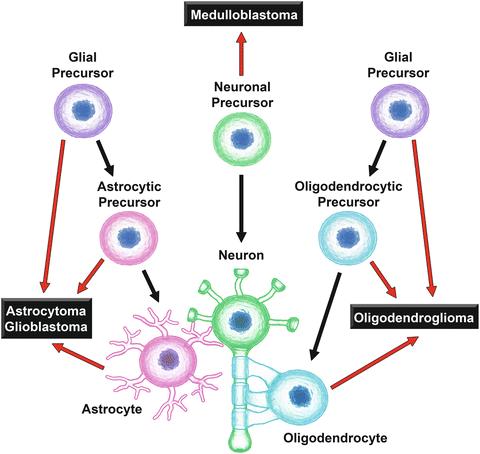
Fig. 24.3
Origins of the major cell populations in normal brain and the proposed origins of the most common types of malignant brain tumors. Black arrows indicate the developmental origins of astrocytes (magenta), neurons (green), and oligodendrocytes (cyan), the three major cell types present in normal brain tissue. Red arrows indicate the proposed originating cells giving rise to the indicated types of human brain neoplasms (Huse and Holland 2010)
Oligodendrogliomas, the third most common type of glioma, are diffusely infiltrating brain tumors arising from oligodendrocytes or oligodendrocyte precursors (Fig. 24.3) and represent 3–5 % of primary brain tumors (Kohler et al. 2011). The majority (60–90 %) of oligodendrogliomas exhibit combined chromosome loss of 1p and 19q, which predicts a less aggressive tumor phenotype and enhanced sensitivity to chemotherapy (Backos et al. 2012). Cultured oligodendrocyte precursors have high levels of iron, lower levels of cellular GSH, and higher levels of baseline oxidative stress compared with astrocytes or neurons. In addition, oligodendrocyte precursors are more sensitive to oxidative stress-induced apoptosis in response to GSH depletion than their mature counterparts (Back et al. 1998). Interestingly, while mature oligodendrocytes also contain elevated iron levels and diminished levels of intracellular GSH, they exhibit enhanced hydrogen peroxide metabolic capacity due to increased enzymatic activity of GPx, GR, and catalase (Butts et al. 2008). The low level of cellular GSH in these cells likely plays a central role in the enhanced susceptibility of oligodendrogliomas to chemotherapy due to decreased GSH availability for non-enzymatic and/or GST-mediated detoxification. The abundance of intracellular iron contained in these cells, which has the capacity to participate in metal-catalyzed oxidation reactions leading to the formation of highly reactive free radical species, also vastly enhances their sensitivity to the chemotherapeutic-mediated generation of ROS (Backos et al. 2012).
Medulloblastoma
While pediatric gliomas, including astrocytoma, glioblastoma, and oligodendroglioma, make up a sizable percentage of brain tumors diagnosed in children under 20 years of age, medulloblastoma is the most common type of childhood CNS malignancy and is the second leading cause of cancer-related death in this age group (Kohler et al. 2011). Medulloblastoma is a rapidly growing and highly invasive brain tumor that arises from the posterior fossa, typically the cerebellum, capable of metastasis to the spinal column and cerebrospinal fluid (CSF) (Gilbertson and Ellison 2008). Currently, 5-year survival stands at roughly 60 % although clinical outcomes vary with age, histological subtype, and metastatic status (Kohler et al. 2011). In contrast to the gliomas, medulloblastomas are thought to originate from immature neuronal progenitors (Fig. 24.3) (Huse and Holland 2010), with tumor cells exhibiting less differentiated and more stem cell-like characteristics, including robust drug resistance (Backos et al. 2012). The GSH detoxification system has been implicated in the resistance of medulloblastoma to alkylating agents. Treatment with GSH or the GSH precursor substrate N-acetylcysteine (NAC) protects medulloblastoma cells against camptothecin (CPT)-induced apoptosis and medulloblastoma cells resistant to cyclophosphamide (CPA) and 4-hydroperoxy-CPA (4-HC) exhibit enhanced cellular GSH levels and γGT expression (Backos et al. 2012). The expression of catalytically inactive polymorphisms of the GST isozymes in a tumor would be expected to result in impaired metabolism of some cytotoxic drugs, rendering these tumors vulnerable to treatment. However, medulloblastoma patients with somatic inactive GST null polymorphisms have an increased risk of adverse events during therapy, including myelosuppression, ototoxicity, nephrotoxicity, and cognitive impairment, as well as lower rates of survival due to the fact that the null phenotype is not strictly limited to the tumor and results in enhanced chemotherapy-associated toxicity to normal tissues (Backos et al. 2012).
Brain Metastases
Distal metastasis to the brain is the final stage of a variety of human neoplasms, including lung cancer, malignant melanoma, renal-cell carcinoma, and breast cancer (Barnholtz-Sloan et al. 2004), and is often responsible for the ultimate fatality of these diseases. Metastases to the brain generally occur due to the colonization of cancer cells separated from the tumor and transported through the bloodstream. The first step in the initiation of metastasis involves the direct contact between tumor cells and the capillary endothelial cells lining the microvasculature. This results in the release of pro-inflammatory cytokines and increased ROS production, which is generally lethal to the metastatic cells (Estrela et al. 2006). However, elevated cellular GSH levels protect against the cytotoxic effects of this initial burst of ROS, with GSH levels directly correlating with tumor cell metastatic potential and pharmacological depletion of GSH substantially reducing metastatic invasiveness (Estrela et al. 2006). Interaction of the tumor with the host cells of the brain microenvironment, the astrocyte population in particular, also plays a significant role in protection from chemotherapy-induced toxicity (Fidler 2011). Astrocytes form direct contacts with melanoma cells in vitro and these interactions result in the upregulation of survival genes, including GSTs, and heightened resistance to chemotherapeutic agents (Kim et al. 2011). This enhancement of tumor cell drug resistance by astrocytes has also been demonstrated in lung and breast cancer cells (Fidler 2011). Upregulation of γGT protein expression has also been observed in many brain tumors and this is associated with enhanced drug resistance due to the increased availability of substrates necessary for GSH synthesis. Thus, metastatic cell populations may be able to exploit the supportive nature of astrocytes and utilize their chemoprotective resources, such as GSH, to support the growth of the tumor and confer greater resistance to therapy.
GSH and GSH-linked Enzyme Contributions to Chemoresistance
Role of GSH as an Antioxidant
Increased ROS production, and the resulting dysregulation of cellular redox homeostasis, plays an integral role in the cytotoxicity of various chemotherapeutic compounds towards tumor cells, many of which are not known to involve formation of free radical species (Wondrak 2009). Numerous studies have demonstrated that the generation of ROS is an underlying mediator of tumor cell apoptosis induced by many chemotherapeutics, including cisplatin, paclitaxel, bleomycin, and etoposide (Wondrak 2009). Although depletion of cellular GSH due to metabolic detoxification of chemotherapeutic compounds can result in the disruption of cellular redox balance and the induction of apoptosis, chemotherapy-induced ROS production is not necessarily related to the loss of GSH per se. For example, cisplatin-induced apoptosis has been shown to be associated with enhanced generation of ROS and not with the induction of nuclear DNA damage, which suggests that oxidative stress may be the central mechanism responsible for cisplatin-induced tumor cell death (Wondrak 2009). Similarly, temozolomide (TMZ), an alkylating agent currently comprising the standard of care for the treatment of adult gliomas (Stupp et al. 2009), is also known to induce ROS production in glioma cells and the acquisition of a TMZ-resistant phenotype in these cells involves a decrease in endogenous ROS production due to an increase in mitochondrial coupling (Oliva et al. 2011). This remodeling of the mitochondrial electron transport chain serves to conserve cellular GSH pools which can then be employed to suppress TMZ-induced ROS production. Thus, the chemoprotective role of GSH in drug-resistant tumor cells is not limited to its functions as a cofactor in the GSH conjugation-mediated metabolism and detoxification of chemotherapeutics, but also includes detoxification of ROS generated by chemotherapeutic agents.
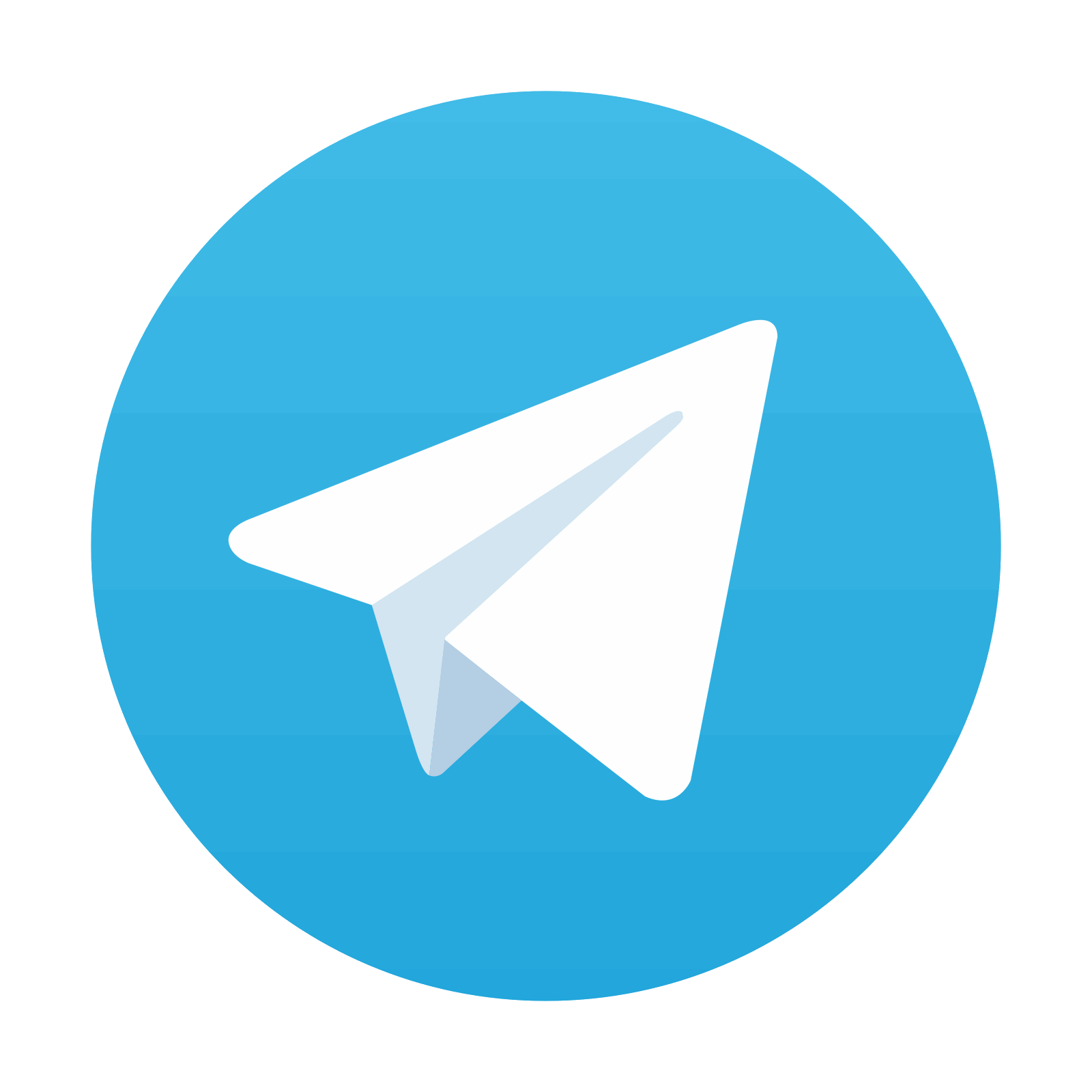
Stay updated, free articles. Join our Telegram channel

Full access? Get Clinical Tree
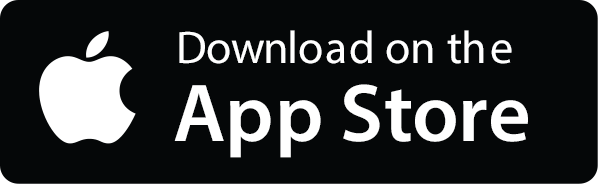
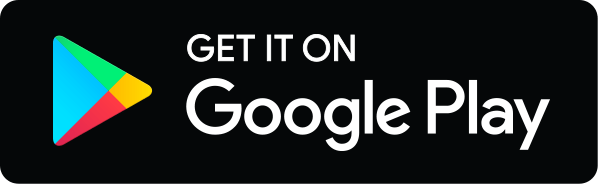