Fig. 26.1
Hyaluronic acid and CD44 are essential components of brain matrix and glioma invasion. (a) Schematic of HA architecture in brain. Brain matrix structure is based on high molecular weight hyaluronic acid (HA), which forms a hydrated network that is crosslinked by other biomolecules. Lecticans such as aggrecan, neurocan, versican, and brevican, are chondroitin sulfate proteoglycans that bind to HA at the N-terminus. HA-lectican binding is stabilized by link proteins. At the C-terminus, lecticans bind to the arms of tenascin-R, -C, and -W, which exist as trimeric or hexameric structures. (b) CD44 expression in the tumor microenvironment. Alterations in CD44 expression with tumor grade, demonstrated by immunohistochemical analysis of CD44 expression in human brain tumors by immunoperoxidase, with nuclei counterstained by eosin. An aggressive GBM tumor (left panel) displays intense staining of CD44. At the leading edge of the tumor (middle panel), neoplastic astrocytes with enlarged nuclei (arrows) display intense CD44 expression, while non-neoplastic cells (arrowheads) display little CD44 expression. In contrast, a noninvasive meningioma (right panel) shows weak CD44 staining. Magnification 400× (Ariza et al. 1995)
The exceptionally high molecular weight of HA is made possible by its unique mechanism of synthesis, which is orchestrated by the three HA synthases (HAS). Other GAGs are typically synthesized in the Golgi apparatus into short sidearms of proteoglycans, whose molecular weights are limited by vesicular transport. HA synthases, in contrast, are channel-like transmembrane proteins that sequester monosaccharides from the cytoplasm, add them onto the HA chain via glycotransferase domains, and extrude the growing linear chain out of the cell as it is synthesized. Thus, restrictions on the size of the HA molecule are not imposed by biosynthesis and transport, and a single molecule can span several microns in length.
In the developing mouse cerebellum, HA organizes into fine web-like structures, which are hypothesized to aid in the migration of interneuron precursors and oligodendroglial cell types (Baier et al. 2007). Analysis of rat brain composition as a function of age shows that HA concentration peaks shortly after birth and then drops off in adulthood. However, in brain tumors, HA secretion is again elevated (Delpech et al. 1993), suggesting that glioma cells may hijack the natural HA-based motility mechanisms employed during development. While this hypothesis has not been clearly demonstrated in glioma cells, HAS2 overexpression in fibrosarcoma cells has been shown to have a direct link to tumorigenicity; cells transfected with HAS2 demonstrated increased proliferation in a soft agar assay, and produced larger tumors in a nude mouse model (Kosaki et al. 1999). Whether HA elevation in glioma is due to HAS overexpression per se or some expression-independent enhancement in HAS activity remains unclear.
In the early stages of neural crest and brain development, levels of hyaluronidases (encoded by six hyal genes), the primary enzymes that degrade HA, are also at their peak as matrix turnover is necessary for cell migration. Increased HAS expression is only beneficial for cell migration if hyaluronidase is concurrently secreted; increased HA adhesive contacts must also be released for productive cell movement to occur (Enegd et al. 2002). Animal studies reveal that Hyal-2 overexpression facilitates tumor angiogenesis and formation in the HA-rich brain, but not in an HA-poor subcutaneous environment (Novak et al. 1999). Upon degradation of high molecular weight HA chains by hyaluronidase secretion from tumor cells, low molecular weight HA by-products of roughly 20 or fewer disaccharides are released, which then stimulate endothelial cells in neighboring blood vessels to undergo angiogenesis (Liu et al. 1996). While this mechanism is also necessary for the initiation of wound healing and matrix remodeling, it is one of the many HA-based signals that gliomas co-opt for aberrant growth and invasion. HA-based signaling mechanisms within glioma cells themselves are discussed in the next section.
Adhesion and Signaling Effects of Hyaluronic Acid Receptors
Chief among the diverse family of HA-binding adhesion proteins is CD44, a single pass transmembrane receptor that is upregulated in a variety of solid tumors, including brain tumors, and whose expression correlates with high glioma grade and poor prognosis (Ranuncolo et al. 2002). While the field has not converged on a single, dominant “canonical” CD44 pathway, the effects of HA binding with CD44 converge on proliferation, cell survival, and anti-apoptotic fate decisions through a variety of pathways (summarized in Fig. 26.2). CD44 does not have any intrinsic kinase activity, but rather executes its signaling effects by binding to kinases and other signaling molecules via its cytoplasmic tail, thereby recruiting these molecules to the cell membrane. While some CD44-HA signaling effects can be initiated by a single binding event, the high molecular weight characteristic of HA also serves to organize an activity-rich signaling center by bringing together many receptors and their downstream signaling partners. Indeed, CD44 tends to aggregate in protein-rich caveolae or lipid rafts.
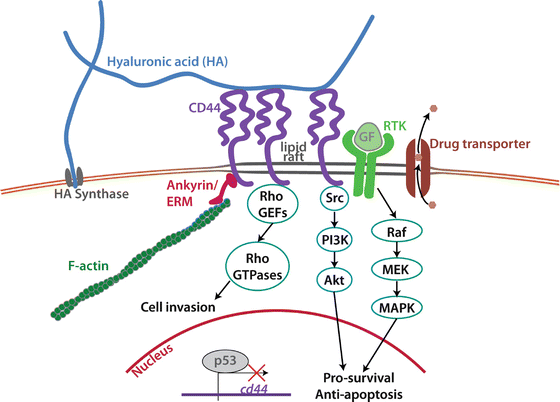
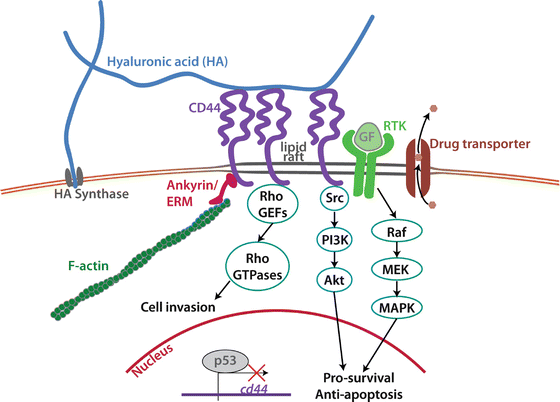
Fig. 26.2
Signal transduction mediated by hyaluronic acid (HA)-CD44 binding. HA is synthesized at the cell membrane by HA synthase, and extruded into the extracellular matrix. HA then binds to CD44, which can in turn associate with filamentous actin via ankyrin or the ezrin-ridixin-moesin (ERM) family proteins. RhoGTPases can also bind to the tail of CD44 via adaptor proteins. CD44 can associate in lipid rafts with receptor tyrosine kinases (RTKs) or multidrug efflux transporters on the cell membrane to promote their pro-survival and anti-apoptosis activity. All of these effects act in concert to promote cancer cell proliferation, motility, and chemoresistance. Transcription of CD44 is kept in check by the tumor suppressor protein p53
At the gene level, CD44 transcription is suppressed by binding of p53 to a non-canonical binding sequence in the CD44 promoter (Godar et al. 2008). Godar et al. showed that many of the oncogenic effects of p53 loss are mediated by an increase of CD44-based survival signaling. Once CD44 is translated and brought to the cell membrane, CD44 complexes with a variety of pro-tumorigenic receptor tyrosine kinases to promote their kinase activity, including EGFR and ErbB2, TGFβ receptor, and c-Met/HGFR (Jung et al. 2011).
CD44 is capable of indirectly engaging the actin cytoskeleton through its cytoplasmic tail via the adaptor proteins ankyrin, and the ERM (ezrin-ridixin-moesin) family proteins. This interaction is essential for cell motility stimulated by phorbol ester, an analogue of diacyl glyceride (DAG). Legg et al. (2002) showed that the resulting activation of protein kinase C (PKC) triggers dephosphorylation of CD44 on Ser325 and phosphorylation on Ser291, then used fluorescence resonance energy transfer (FRET) to show that this dual change in phosphorylation reduces interaction between CD44 and ezrin. This dynamic control of ezrin association and dissociation via modulation of phosphorylation state is necessary for rapid chemotactic response to DAG gradients. Aside from anchoring to filamentous actin, CD44 also promotes cell motility by activating RhoGTPases. This occurs via recruitment of guanine nucleotide exchange factors (GEFs) to the cell membrane, facilitating interaction with their effectors, the Rho family of GTPases. Addition of soluble HA to cell culture medium quickly activates Rac-1 by recruiting the GEFs Tiam1 and Vav2, and induces lamellipodia formation within several minutes (Oliferenko et al. 2000) in both primary astrocytes and mammary epithelial cells. Similarly, in mammary epithelial cells, CD44-HA binding activates RhoA by recruiting p115 RhoGEF and myosin-mediated cell motility (Bourguignon 2008).
Other molecular mechanisms suppress the tumor-promoting effects of CD44 association with F-actin and activation of Rho GTPases. The most notable of these CD44-antagonizing molecules is merlin, the tumor suppressor protein encoded by the NF2 gene, which blocks association with actin when bound to CD44. This also decreases CD44 affinity for HA, and thus reduces the intensity of downstream pro-tumorigenic signaling (Bai et al. 2007). In this way, CD44 acts as a molecular switch that promotes proliferation when ERM proteins are bound and quiescence when merlin is bound. Significantly, merlin is an essential antagonist of the Hippo signaling pathway, which attenuates apoptotic responses to oxidative stress and cytotoxic drugs. CD44 knockdown in glioma cells reduces merlin phosphorylation (which is required for CD44 binding) and blocks the cell survival signals downstream of Hippo, thereby inducing p53 expression and increasing survival in an animal model (Xu et al. 2010).
In addition to interacting with actin binding proteins and RhoGTPases to promote cell motility, the cytoplasmic tail of CD44 also facilitates the formation of signaling complexes that ultimately promote cell survival. Namely, CD44 recruits several Src family non-receptor kinases such as Lyn (Lin 2001), Lck, Fyn, and c-Src (Bourguignon et al. 2001), which activate PI3K-Akt signaling. Integrins are perhaps the best known and widely studied cell adhesion receptors, so it is worth noting that the cytoplasmic tail of CD44 also interacts with integrin-based focal adhesion proteins. For instance, CD44 has been shown to complex with focal adhesion kinase (FAK) in a lung cancer cell line, and cells stimulated by HA express increased activated (FAK), which in turn augments both the PI3K and MAPK pathways (Fujita et al. 2002). Thus, this extensive set of interactions allows CD44 to exert its signaling effects even in the absence of any intrinsic kinase activity.
Finally, RHAMM (receptor for hyaluronic acid mediated motility) is another HA receptor that has been studied for its pro-tumorigenic effects. The functions of RHAMM and CD44 appear to be inextricably linked; many roles of RHAMM involve supporting CD44-HA binding and downstream signaling. Additionally, in an arthritic mouse model, increased RHAMM expression has been shown to phenotypically complement CD44 gene suppression, as CD44 knockout mice develop normally (Nedvetzki et al. 2004). While the two receptors share many of the same binding partners, RHAMM does have other tumorigenic roles independent of CD44; for example, its other primary role is to facilitate the assembly of mitotic spindles required for cell division by assisting in microtubule nucleation. This role, independent of HA binding functions, is known to be especially active in breast cancer cells, where it is proposed that overexpressed RHAMM drives aberrations in mitotic spindles and thus supports genomic instability.
Significance of CD44 in Cancer Stem-Like Cells
Historically, the progression of GBM has been framed in terms of clonal evolution models, in which cellular heterogeneity arises from the stochastic accumulation of different mutations by different cells. More recently, a new prevailing paradigm is emerging in which GBM is thought to progress according to a cancer stem cell hypothesis, in which a specialized and perhaps rare subpopulation possessing the hallmark stem cell characteristics of self-renewal and multipotency give rise to a heterogeneous bulk tumor population. While components of this model remain somewhat controversial, a preponderance of evidence now supports that a stem-like subpopulation of cells exists within the heterogeneous tumor population that has much higher tumorigenic potential than the other cells. For example, only specific cell subpopulations of primary tumors are capable of histologically recapitulating human tumors when orthotopically implanted at low numbers in immunocompromised mice. These subpopulations often share key features of normal neural stem cells such as expression of neural stem cell markers (e.g. nestin), the ability to form clonal neurospheres, strong preference for laminin-based ECMs, and, most critically, the ability to self-renew and differentiate into cells positive for neural, astrocytic, and oligodendrocytic markers. As is commonly done in the field, we will refer to this stem-like population as brain cancer stem cells (BCSCs).
BCSCs can be selected from a primary tumor by culturing tumor explants in neurobasal medium supplemented with growth factors, which is also used as a neural stem cell growth medium. Some fraction of the tumor cells will form clonal neurospheres under these conditions, and these clones satisfy specific criteria including expression of specific markers, multipotency, and – critically – the ability to histologically recapitulate GBM when orthotopically implanted into immunocompromised mice. These cells are designated as BCSCs, and significantly, this population is enriched in CD44 expression. One possible functional consequence of CD44 overexpression is the promotion of multidrug resistance proteins on the cell membrane, which confer chemoresistance by pumping cytotoxic agents out of the cancer cell (Toole and Slomiany 2008). An alternative method of isolating cancer stem cell populations involves collection of “side population” cells, which are defined by low uptake of Hoechst dyes due to high expression of ABCG2 (BCRP) and ABCB1 (MDR1) drug transporters of the ABC (ATP-binding cassette) family (Hirschmann-Jax et al. 2004). This expression pattern partially accounts for the highly chemoresistant characteristics of BCSCs compared to their bulk tumor cell counterparts. BCSCs also divide, or cycle, less rapidly than bulk tumor cells, reducing the uptake and efficacy of anti-cancer drugs whose mechanism of action requires cell division.
Flow cytometry and histological analysis of human brain tumors reveals that glioma cells express a variety of these drug transporters, which are expressed more frequently in high grade tumors (Calatozzolo et al. 2005). In a malignant peripheral nerve sheath tumor cell line, CD44 forms a stable complex with ABCG2 in the plasma membrane (Slomiany et al. 2009). This complex is disrupted by adding oligomeric HA, which competes with endogenous high molecular-weight HA for CD44 binding, and leads to internalization of both CD44 and ABCG2. Finally, cells treated in this way are rendered more susceptible to apoptosis induced by the anti-cancer DNA intercalating agent doxorubicin. Since ABCG2 is highly expressed in BCSCs (Bleau et al. 2009), strategies to disrupt the association of CD44 and multidrug transporters expressed on BCSCs may prove to be effective ways of improving the effectiveness of chemotherapy treatments for malignant gliomas. The role of HA in the tumor matrix on promoting multidrug resistance in BCSCs has not yet been investigated.
While CD44 is increasingly accepted as a BCSC marker, the functional significance of CD44 enrichment in BCSCs remains unclear. Recently, Jijiwa et al. (2011) began to investigate these questions through the identification and characterization of CD44 variants in BCSCs. While CD44s is the standard isoform of the receptor, variant isoforms can be formed by alternative splicing of exons encoding the middle stalk region of the receptor; all isoforms contain the same intracellular domain and HA-binding domain. Expression of the variant isoform CD44v6 has long been known to be exclusively expressed by cancer cells of other tissues where it promotes cancer malignancy and invasiveness, but now it is known that CD44v6 is also expressed by BCSCs (Jijiwa et al. 2011). CD44v6 promotes cell survival signals by Akt phosphorylation when it binds to its secondary ligand, osteopontin. Thus, the further exploration of the functional role of CD44 expression in the BCSC specialized cell population will surely unlock greater understanding of the mechanisms of GBM progression.
In Vitro Culture Models of Brain Tumor Invasion
Any cell-level in vitro study of GBM must strike a balance between interpretability and physiological mimicry. On one extreme, cell culture paradigms employing two-dimensional tissue culture polystyrene (TCPS) surfaces are convenient and widely used, but they do not recapitulate the myriad microstructural, biophysical, and biochemical features of the in vivo brain microenvironment. When one’s goal is to systematically test the regulatory role of specific, defined features of this microenvironment, it may be advantageous to use these highly defined albeit reductionist platforms. In other cases, it may be desirable to incorporate as many brain matrix characteristics as possible. We now briefly review strategies that have been used to study GBM in a cell-scale in vitro setting, with a focus on the incorporation of HA in these systems. In addition to the models described here, there are also a variety of in vivo animal models that are available for studying heterotypic cell interactions (e.g. with endothelial cells) or more clinically relevant endpoints (e.g. tumor size or animal survival).
2D Culture Models
The simplest approach for studying the effects of soluble factors such as HA is to place them in the cell culture medium itself. This format has been vitally important in identifying the many biochemical signaling effects of HA. However, from a biophysical standpoint, it does not mimic important structural aspects of large matrix molecules such as full-length adhesive proteins or full-length HA. To study the adhesive effects of these matrix macromolecules, they can be adsorbed onto glass or TCPS surfaces. However, the disadvantages to this approach are that the stiffness of these surfaces is orders of magnitude higher than most tissues including brain, that adsorption can alter the biological activity of the adsorbed ligand, and that the adsorption is non-specific. To mitigate many of these disadvantages, polyacrylamide and other polymer hydrogel substrates have been widely used to create matrix materials with finely tunable stiffness (Wang and Pelham 1998) by varying the ratio of the monomer and crosslinker (e.g. acrylamide and bis-acrylamide). Matrix proteins such as fibronectin or collagen can be covalently attached to the hydrogel surface, often with heterobifunctional coupling agents such as sulfo-SANPAH (sulfosuccinimidyl-6-(40-azido-20-nitrophenylamino) hexanoate) which may be conjugated to the gel surface with UV irradiation and to ECM proteins via NHS-ester chemistry. Aside from the selective covalent attachment of desired proteins using this chemistry, serum proteins do not adsorb onto polyacrylamide, allowing for a highly controlled biochemical and biomechanical surface.
More recently, soft lithographic techniques have emerged as a powerful method to selectively pattern precise 2D geometries of adhesive proteins set on an adhesion-resistant background (Chen et al. 1997). Typically, a molded stamp of polydimethylsiloxane (PDMS) is coated with adhesive protein, and stamped onto a bioinert layer. The effects of cell shape, cell size, and cell-cell interactions have been well explored with this technique, as well as “1D” studies of cell migration on thin confined paths.
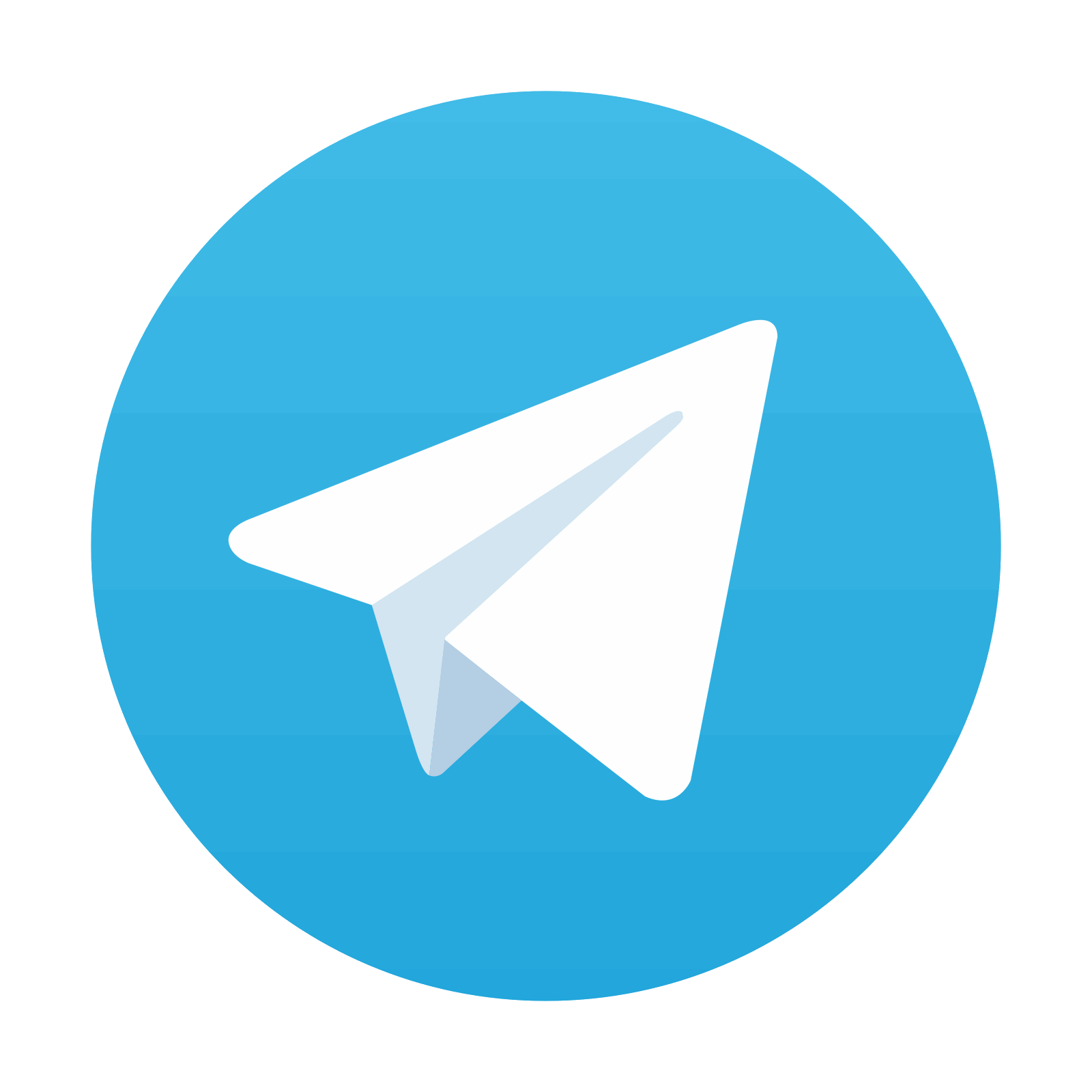
Stay updated, free articles. Join our Telegram channel

Full access? Get Clinical Tree
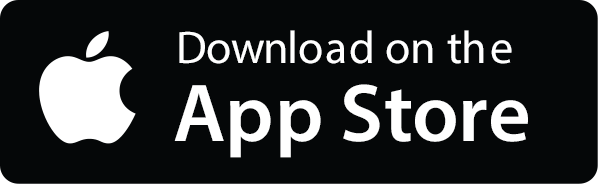
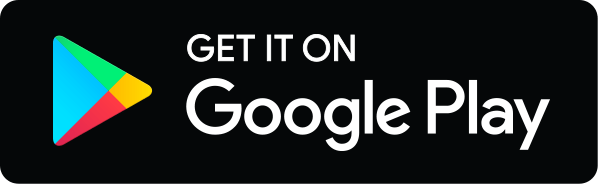