Figure 18-1. Summary of anterolateral system and anterior trigeminothalamic tract fibers conveying nondiscriminative tactile, thermal, and nociceptive inputs to the contralateral somatosensory cortex.
ANTEROLATERAL SYSTEM
Fibers within the lateral spinothalamic tract were considered to carry only pain and thermal information, whereas the anterior spinothalamic tract was thought to be concerned only with nondiscriminative touch. This older view of separate tracts conveying separate modalities of sensory information is not used in this chapter. Current thinking holds that all parts of the ALS carry all modalities (pain, temperature, and touch) but that there are direct and indirect pathways for this information to reach the brain. The direct pathway is the neospinothalamic pathway (spinal cord → lateral thalamus → somatosensory cortices), and the indirect pathway is the polysynaptic paleospinothalamic pathway (spinal cord → reticular formation → medial thalamus → cingulate, frontal + limbic cortices). Both of these pathways, plus other ascending fibers as defined later, collectively form the ALS.
The ALS is a composite bundle that includes spinothalamic, spinomesencephalic, spinoreticular, spinobulbar, and spinohypothalamic fibers. Spinothalamic fibers project directly from the spinal cord to the ventral posterior nuclei (ventral posterolateral [VPL] and ventral posterior inferior [VPI] nuclei) and the posterior nuclear group (including the ventral medial nucleus VMpo) of the thalamus. Collaterals to the reticular formation arise from some of these axons. Spinomesencephalic axons project to the periaqueductal gray (PAG) and to the tectum; the latter are spinotectal fibers. Although spinoreticular fibers project to the reticular formation of the medulla, pons, and midbrain, collaterals may ascend as reticulothalamic fibers to other targets, such as the intralaminar and dorsomedial nuclei of the thalamus. Spinohypothalamic fibers terminate in hypothalamic areas and nuclei, including some that give rise to hypothalamospinal axons. Projections of less relevance to the somatosensory system, such as spinoolivary fibers, are grouped under the category of spinobulbar fibers.
Cutaneous Nociceptors and Primary Neurons
The receptors for nondiscriminative touch, innocuous thermal stimuli, and nociceptive stimuli are distributed in glabrous and hairy skin as well as in deep tissues, including muscles, joints, blood vessels, and viscera (Table 18-1). Morphologically, these receptors are all free nerve endings (see Fig. 17-1); that is, they lack specialized receptor cells or encapsulations. Because of this lack of specialization, the basis for their submodality specificity is unclear. Nonetheless, these submodalities are transduced by activation of peripheral branches of either thinly myelinated Aδ (A-delta) fibers or unmyelinated C fibers. The density of free nerve endings and the corresponding size of receptive fields vary over the body surface in the same way as for other cutaneous receptors (see Fig. 17-3), being highest on the hands and in the perioral area. Regardless of size or location, however, each field is exquisitely sensitive to thermal, chemical, or mechanical stimuli.
Table 18-1 Classification of Cutaneous Mechanical, Thermal, and Nociceptive Receptors by Small-Diameter Fibers and Their Adequate Stimuli
Nondiscriminative touch results from the stimulation of free nerve endings that act as nonnoxious high-threshold mechanoreceptors (Table 18-1). These receptors respond to any rough stimulus, including tapping, squeezing, rubbing, and stretching of the skin, that does not result in tissue damage (Fig. 18-2A). Nerve fibers associated with these receptors generally have no background activity when they are unstimulated; when they are stimulated, they respond with a sustained discharge that signals stimulus duration (Fig. 18-2A).
Figure 18-2. Fibers conveying information from high-threshold mechanoreceptors (A) respond to the application of a punctate stimulus. Thermoreceptors show a graded response to increases in temperature (B, left), whereas burns produced by prolonged thermal stimulation evoke high-frequency response in thermonociceptors (B, right).
Nonnociceptive thermoreceptors fall into two classes: those activated by warmth (35° C to 45° C) and those activated by cooling (17° C to 35° C). They show a graded response to changes in ambient temperature (Fig. 18-2B). With repeated stimulation, these receptors become sensitized and have a decreased activation threshold and a larger response to the application of a stimulus.
Nociceptors (pain receptors) are found in cutaneous as well as in deep structures. Two major classes of cutaneous pain receptors have been identified. They are the Aδ mechanical nociceptors and C-polymodal nociceptors. These receptors are found at the end of peripheral processes of thinly myelinated (Aδ) or nonmyelinated (C) fibers (Table 18-1). The cutaneous receptive field of an Aδ nociceptor consists of a number of small sensitive spots (2 to 30) scattered over an area of skin. Each spot ranges from 50 to 180 µm in diameter. Aδ mechanical nociceptors respond to mechanical injury accompanied by tissue damage. C-polymodal nociceptors respond to mechanical, thermal, and chemical stimuli. The cutaneous receptive field of a C-polymodal nociceptor usually consists of one or two sensitive spots, with each spot covering an area of skin 1 to 2 mm2. For a comparable region of skin, the C fiber spots are larger but fewer in number than the Aδ spots, which are smaller but more numerous.
Other cutaneous receptors that respond to high-threshold or noxious stimuli have been identified. They include receptors that respond to extreme temperature changes (thermonociceptors) (Table 18-1) and receptors that respond to chemicals, irritants, or algesic (pain-producing) compounds (chemonociceptors) (Table 18-1). Extreme heat (>45° C) or cold (<17° C) that burns or freezes the skin produces high-frequency firing in both Aδ and C thermonociceptors (Fig. 18-2B). C fiber chemonociceptors (Table 18-1) are activated by the release of endogenous substances associated with tissue damage and inflammation, such as bradykinin and hydrogen ions, and foreign irritants such as insect venoms.
Transient Receptor Potential Channels and Thermal Sensations
Specialized surface membrane receptors called transient receptor potential (TRP) channels have recently been identified in cutaneous Aδ and C thermonociceptors. Several of these channels respond to application of either noxious heat or noxious cold. The most common of these receptors, the capsaicin receptor TRPV1, is activated by capsaicin (the active ingredient of chili peppers), noxious temperatures (>43° C), and protons. A second channel, the TRPV2 receptor, is activated by high intensities of noxious heat (>52° C). The cold receptor TRPA1 responds to very low temperatures (<16° C) and some chemical substances. When activated, these receptors can signal thermal pain.
Nonnociceptive thermal stimuli activate TRP channels in Aδ and C thermoreceptors. Warm thermoreceptors express TRP3 (>33° C) and TRP4 (24° C to 34° C) receptors. Cold thermoreceptors express TRPM8 (<23° C) receptors, which respond to cooling and menthol. Activation of these receptors can signal the range of innocuous thermal sensations.
Peripheral Sensitization and Primary Hyperalgesia
Pain receptors, unlike Meissner corpuscles or Merkel cells, demonstrate a unique phenomenon called sensitization. After an insult, these receptors become more sensitive (lower pain activation threshold) and thus more responsive (increases in firing rate) to noxious stimulation within their receptive fields. Although the mechanisms responsible for receptor sensitization are not completely known, irritating chemicals (capsaicin), inflammatory mediators (bradykinin, prostaglandins), and neurotransmitters (serotonin, histamine, norepinephrine) released from damaged skin or byproducts from plasma, or both, are thought to contribute to this phenomenon. As a result of this heightened sensitivity, the affected area is exquisitely sensitive to painful stimuli, and patients experience a sensory disturbance called hyperalgesia (exaggerated response to a painful stimulus). This condition can be differentiated into primary hyperalgesia and secondary hyperalgesia. Primary hyperalgesia occurs in the region of damaged skin and is probably the result of receptor sensitization. Secondary hyperalgesia occurs in the skin immediately bordering the damaged tissue. Although receptor sensitization may contribute to secondary hyperalgesia, there is likely to be a central (e.g., spinal) component as well.
Central Sensitization and Secondary Hyperalgesia
Sensitization of peripheral nociceptors causes an increase in spontaneous activity in the Aδ and C fibers. The central processes of these fibers enter the posterior horn of the spinal cord, where they activate posterior horn neurons in laminae I to V. Ongoing inputs from these injured peripheral nociceptors evoke a number of changes in the central processing of sensory information by the posterior horn neurons. These changes include a marked increase in the receptive field size of the posterior horn neurons (to include skin areas not involved in the initial injury), an increased response of the cells to the application of suprathreshold stimuli, a decreased threshold to stimulus application in the receptive field, and the activation of the cell by novel inputs (e.g., a light breeze). This phenomenon is known as central sensitization, and it represents a potentiated state in which the system has been shifted from one functional level (normal) to another (sensitized). In most patients, an innocuous stimulus, such as a gentle breeze or a light touch, can evoke pain sensation in the skin bordering the damaged tissue. The perception of an innocuous stimulus as painful is referred to as allodynia and can be the result of central sensitization.
Pain Receptors in Muscles, Joints, and Viscera
In addition to cutaneous pain receptors, pain receptors in muscles, joints, blood vessels, and viscera have also been identified (Table 18-2). Muscle pain is mediated by receptors of both group III (thinly myelinated) and group IV (nonmyelinated) afferent fibers. Excessive stretch or contraction after strenuous exertion may activate the muscle pain receptors of group III fibers. Receptors of group IV fibers may be activated in response to the release of algesic compounds after muscle injury or ischemia. Joint pain, including arthritis, may be caused by inflammation. This pain is mediated by receptors associated with group III and group IV fibers. Visceral pain is often described as being diffuse and difficult to localize and is frequently referred to an overlying somatic body location. In addition, visceral pain usually involves autonomic reflexes. Visceral pain receptors located in the heart, respiratory structures, gastrointestinal tract, and urogenital tract are poorly identified (Table 18-2). These receptors can be activated by intense mechanical stimuli including overdistention or traction, ischemia, and endogenous compounds, including bradykinin, prostaglandins, hydrogen ions, and potassium ions. Activation of these receptors produces pain.
Table 18-2 Classification of Deep and Visceral Nociceptors and Their Adequate Stimuli
5-HT, 5-hydroxytryptamine.
Of the two primary afferent fiber types carrying nociceptive sensations, Aδ fibers have a slightly faster conduction velocity (5 to 30 m/s) than that of C fibers. They carry well-localized acute sensations that do not evoke an affective component to the sensory experience. A pinprick, used clinically to test ALS function, is one stimulus that activates Aδ fibers. On the other hand, C fibers are smaller and conduct more slowly (0.5 to 2 m/s). They transmit poorly localized diffuse sensations that produce a noticeable affective response. For example, the dull, persistent ache that follows a muscle pull results from activation of C fibers. Both Aδ and C fibers are considerably smaller and conduct more slowly than fibers of the PCMLS. A nerve block or anoxia preferentially affects large-diameter, heavily myelinated fibers and thus usually results in loss of discriminative tactile, vibratory, and postural sensations to varying degrees. Local anesthetics, such as lidocaine and bupivacaine, preferentially affect small-diameter Aδ and C fibers by blocking sodium channels and thus result in loss of nociception (analgesia).
Cell bodies of C and Aδ fibers are generally small compared with other pseudounipolar neurons in the posterior root ganglion. The central processes of these cells enter the spinal cord via the lateral division of the posterior root (see Fig. 17-5). Many smaller fibers contain excitatory amino acids such as glutamate as well as peptides such as substance P and calcitonin gene–related peptide that may serve as neurotransmitters. The central processes of these fibers also contain surface membrane receptors. They include a γ-aminobutyric acid (GABA) receptor, a serotonin (5-hydroxytryptamine) receptor, and a mu opioid receptor. Pharmacologic therapies as well as descending fibers (see Figs. 18-20 and 18-21) can act at these presynaptic sites and suppress the initiation of the pain signal or block transmitter release from primary afferent terminals. In addition to their normal trajectory into the posterior horn, a small number of C fibers enter the spinal cord through the anterior root of a spinal nerve. It is possible that these fibers provide a basis for the return of pain after posterior rhizotomy, a procedure in which posterior roots are sectioned in an attempt to alleviate intractable pain.
The strip of skin that is innervated by the peripheral cutaneous branches of a given spinal nerve is called a dermatome (Figs. 18-3 and 18-4). The central processes of these nerves that convey cutaneous input terminate in the posterior horn. It is clinically useful to examine dermatomes that have relationships to landmarks on the body: for example, C7 for the index finger, the T4 to T5 border at the nipples, T10 at the navel, L1 along the pelvic rim, L5 for the big toe, and S4 and S5 for the genitalia and anus (Fig. 18-4). There is overlap between the peripheral and central distribution of adjacent spinal nerves and consequently between their dermatomes (Fig. 18-3). This overlap reduces the effects of injury to a single spinal root.
Figure 18-3. The dermatomes formed by the peripheral processes of adjacent spinal nerves overlap on the body surface (see Fig. 18-4). The central processes of these fibers also overlap in their spinal distribution.
Figure 18-4. A representation of the dermatomal maps on the anterior (A) and posterior (B) surfaces of the body, on the face (C), and on the hands and feet (D). The patterns of the body may vary slightly from patient to patient, but the more commonly used landmarks are shown here and include the shoulder (C5-C6), hand (C6-C8), nipple (T4), umbilicus (T10), inguinal region (T12-L1), knee (L3, L4), and great toe (L4). More can easily be filled in from these landmarks.
Shingles (herpes zoster) is a disease of viral etiology that is noteworthy for its dermatomal distribution (Fig. 18-5). Subsequent to a bout of chickenpox, viral DNA may infect and become latent in trigeminal and posterior root ganglion cells. The virus may reactivate periodically, producing infectious virions that travel down the peripheral processes of the neurons to produce a painful skin irritation in the dermatomal distribution of the ganglion (Fig. 18-5). When an injury or disease process affects a series of nerve roots, the result is diminished sensibility (hypesthesia) over the dermatomes served by those roots. The borders of the hypesthetic region correspond to dermatomal boundaries. However, the most debilitating aspect of this disease is a poorly understood recurrence of pain. Known as postherpetic neuralgia, this condition falls under the category of neuropathic pain.
Figure 18-5. A case of herpes zoster (shingles) in an adult man. The ophthalmic division of the trigeminal nerve is involved; note that the lesions do not cross the midline. The border between the ophthalmic and maxillary divisions on the lateral aspect of the nose may vary slightly among patients.
Clinically, it is important to test patients for intact ALS and PCMLS function. In testing of the ALS, a single pinpoint applied to the skin should evoke a response (perception of pain) from the patient. ALS modalities are also evaluated by comparing pinprick of one side of the face with the other side or by comparing more distal portions of the extremities (toes and fingers) with more proximal portions (knees and elbows). Patients with diabetes mellitus may experience a sensory loss, including pinprick, that starts distally and proceeds proximally; this is a stocking-glove sensory loss because it initially affects the feet and hands first. Function of the intact PCMLS is tested by the simultaneous application of two points spaced at measured intervals. As the points are moved closer, the ability to identify them as separate stimuli (two-point discrimination) decreases and eventually disappears. The PCMLS is also tested by applying a 128-Hz tuning fork (vibratory sense) to a bone prominence or the tip of a finger or toe. The patient perceives this as a buzzing sensation. It is common to test both pain/thermal sense and discriminative touch/vibratory sense on both sides of the face and body to see if there are asymmetries.
Central Pathways from the Body
Aδ and C fibers enter the spinal cord via the lateral division of the posterior root entry zone. The fibers travel in the posterolateral fasciculus (Lissauer tract) and bifurcate into ascending and descending branches (see Fig. 17-5). Some fiber collaterals terminate on interneurons in the spinal gray matter. These connections participate in the circuits that mediate spinal reflexes, such as the flexor withdrawal reflex (see Chapter 9).
The central target of nociceptive primary afferent fibers includes laminae I, II, and V of the posterior horn (Fig. 18-6). Aδ fibers target laminae I and V. Rexed lamina I, the posteromarginal nucleus (or zone), receives mainly input from Aδ fibers (Fig. 18-7A). Neurons in this nucleus (or zone) project to other spinal cord laminae, the brainstem reticular formation, and the thalamus (Fig. 18-7B). Lamina II, the substantia gelatinosa, is divided into outer (IIo) and inner (IIi) layers. Input to IIo and IIi is derived primarily from C fiber primary afferents, and IIi also receives input from collaterals of nonnociceptive afferent fibers. Lamina II contains excitatory and inhibitory interneurons that project to other laminae of the posterior horn. In addition, some neurons in IIo are involved in relaying sensory information to supraspinal sites, including the thalamus (Fig. 18-7B).
Figure 18-6. Weil (myelin)–stained section of the medullary posterior horn (A) at the level of the pyramidal decussation and the spinal posterior horn (B) at levels C7 to C8. The spinal laminae that correspond to medullary structures are labeled.
Figure 18-7. Summary of posterior horn laminae and their major sensory inputs (A) and major outputs (B).
Neurons in laminae III and IV, the nucleus proprius (posterior proper sensory nucleus), receive nonnoxious inputs from the periphery. Cells in these laminae project to deeper laminae of the spinal cord, to the posterior column nuclei, and to other supraspinal relay centers including the medulla, pons, midbrain, thalamus, and hypothalamus (Fig. 18-7B).
Lamina V neurons receive both noxious and nonnoxious (nociceptive and nonnociceptive) inputs and project to the medullary and mesencephalic reticular formation, thalamus, and hypothalamus (Fig. 18-7B). Neurons in deeper laminae of the spinal gray receive (directly and indirectly) noxious and nonnoxious inputs and connect with neurons in other spinal cord levels (propriospinal connections).
Posterior horn neurons exhibit numerous membrane receptors. These include a GABA receptor, a serotonin receptor, a mu opioid receptor, and the AMPA and NMDA receptors for glutamate. Descending fibers from the raphe nuclei and locus ceruleus can act on postsynaptic sites and can effectively block forward transmission of the pain signal.
The functional properties of posterior horn neurons reflect the type of primary afferent fiber input received. These neurons are classified as low threshold (nonnociceptive), nociceptive specific (noxious), wide dynamic range (nonnociceptive and noxious), or deep on the basis of their responses to different stimulus modalities.
The fibers of the ALS participate in both direct and indirect spinothalamic pathways (Fig. 18-1). Most Aδ fibers contribute to the direct (neospinothalamic) pathway, which carries nondiscriminative tactile, innocuous thermal, and nociceptive signals. When these Aδ fibers enter the posterolateral fasciculus and bifurcate, their branches travel rostrocaudally for three to five spinal levels. The descending branches terminate on interneurons within the spinal gray and contribute to the execution of segmental spinal reflexes. The ascending branches terminate on second-order neurons (tract cells) in lamina I of the posterior horn (Fig. 18-7A). These tract cells, in turn, project to the lateral region of the thalamus. The great majority of their axons cross the midline of the spinal cord obliquely via the anterior (ventral) white commissure and ascend in the contralateral ALS. A few ascend in the ipsilateral ALS. The thalamic (third-order) neurons of these pathways are located mainly in the VPL, the VPI, and the posterior group including VMpo. This laterally organized, direct pathway underlies rapid and precise localization and discrimination of nociceptive stimuli.
The polysynaptic indirect (paleospinothalamic) component of the ALS relays noxious and innocuous mechanical and thermal information to the medial brainstem reticular formation. The input to this pathway originates chiefly from C fibers. Branches of these fibers ascend and descend one or two levels in the posterolateral fasciculus to synapse on interneurons in laminae II and III (Fig. 18-7B). These interneurons influence tract cells in laminae V to VIII, which send axons that cross obliquely through the anterior white commissure (over a distance of one to three segments) to join the contralateral ALS. These spinoreticular fibers terminate in the brainstem reticular formation, which in turn projects to the medial thalamic nuclei. This medially organized indirect pathway contributes to the perception of dull pain sensations as well as to behavioral and motivational changes associated with nociceptive stimulation.
Fibers in the ALS are arranged somatotopically
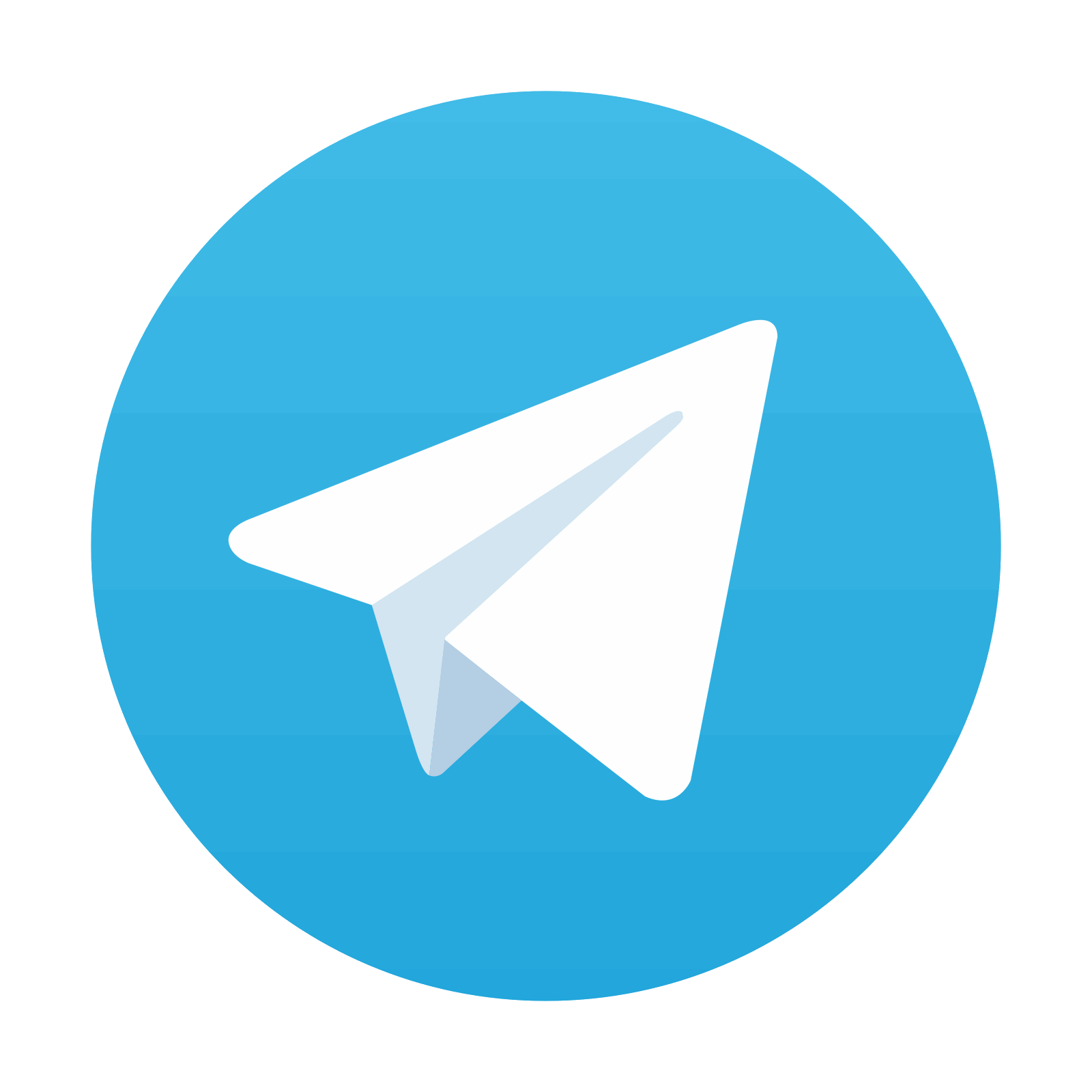
Stay updated, free articles. Join our Telegram channel

Full access? Get Clinical Tree
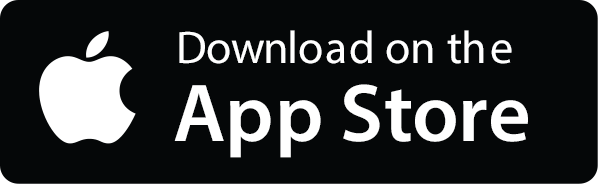
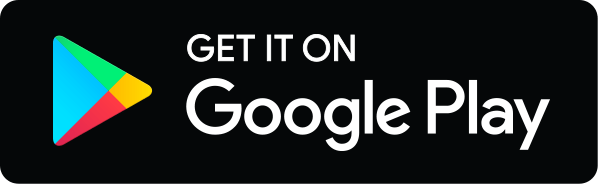