
Figure 4.1 Schematic illustration of the time course of the processes involved in a computer-paced working memory span task in which letters must be remembered while the processing component requires successive retrievals (R). The three panels illustrate how variations in the time allowed to perform a fixed number of retrievals constrain the switching process between processing and maintenance. Adapted with permission from Barrouillet, P., Bernardin, S., and Camos, V. (2004). Time constraints and resource sharing in adults’ working memory spans. Journal of Experimental Psychology: General, 133, 83–100, APA Publisher.
Two main predictions issue from these proposals. First, working memory spans mainly depend on the rate at which the processing component of the task has to be performed, that is, in our example, on the Number of retrievals/Time ratio. Second, simple activities can have an effect on maintenance which is as detrimental as complex activities provided that they require attention and are also performed at a high rate.
Exploring the cognitive load as the Number of retrievals/Time ratio
The predictions concerning the effect of the Number of Retrievals/Time ratio were tested in a series of experiments using the reading digit span task (Barrouillet et al. 2004). The critical aspect of this task lies on the time constraints imposed on the participants, who are not allowed to read the digits at their own pace. In a first experiment (Barrouillet et al. 2004, Experiment 4), we manipulated the number of digits to be read while keeping constant the total time allowed to read them. After the presentation of each letter, either 10 or 6 digits were successively displayed on screen at a regular rhythm over a total period of 6 s. In each condition, the length of the series of letters to be remembered was progressively increased from 1 to 7 until the participant failed to recall the letters in the correct order. The time-based resource-sharing model predicts that a larger number of retrievals over a fixed period of time should result in a higher cognitive load and consequently on poorer recall. In line with this hypothesis, the slow-paced condition elicited a higher mean working memory span than the fast-paced condition (4.28 and 2.77 respectively).
(p.65) In the following experiment, the time allowed to read the digits was manipulated while their number was kept constant (Barrouillet et al. 2004, Experiment 5). In the slow-paced condition, participants were given 1s to read each digit, whereas in the fast-paced condition this time was reduced to 600 ms. We predicted that the latter condition would result in a higher cognitive load and thus in poorer recalls. This was exactly what we observed, the slow-paced condition resulting in higher spans than the fast-paced condition (4.76 and 3.01 respectively). This result was of particular interest because it ruled out one of the main predictions issuing from Towse and Hitch’s (1995) proposals. Indeed, the fast-paced condition corresponded to a shorter duration of processing. Their theory would predict better recalls because shorter durations of processing involve shorter delays of retention between storage and recall. This was not what we observed, suggesting that, as we surmised, attention must be shared by switching rapidly from processing to maintenance during the intervening activity and that the relative ease of this switching process determines what is called cognitive load.
Finally, we tested the hypothesis that cognitive load is a linear function of the Number of retrievals/Time ratio. Progressively increasing this ratio should result in a smooth and linear decrease in span. For this purpose, nine groups of adult participants were presented with nine different values of the ratio (from 0.4 to 2) resulting from the combination of three different numbers of digits presented (4, 8, or 12) with three total periods (6, 8 or 10 s). As shown in Figure 4.2, recall performance was highly correlated with the ratio, revealing a quasi perfect trade-off between processing

Figure 4.2 Mean working memory spans as a function of the Number of retrievals/Time ratio and the nature of the processing component (reading numerals or saying ‘baba’) from Barrouillet et al. (2004, Experiment 7). Conc. Art. = concurrent articulation. Adapted with permission from Barrouillet, P., Bernardin, S., and Camos, V. (2004). Time constraints and resource sharing in adults’ working memory spans. Journal of Experimental Psychology: General, 133, 83–100, APA Publisher.
Lépine, Bernardin and Barrouillet (2005) conducted an even more stringent test of the alternative hypothesis of an effect of the Number of retrievals/Time ratio that would only be due to articulatory suppression. In their Experiment 3, they manipulated the time allowed to perform a task that did not involve any articulatory suppression. As in the previous experiments, participants were presented with a task in which letters were to be remembered while digits were successively presented on screen. However, they were not asked to read these digits but to judge their parity by silently pressing identified keys on keyboard for odd and even. The time allowed to judge the parity of each number was 800 ms in the fast-paced condition and 1500 ms in the slow-paced condition. Once more, the former condition resulted in lower recall performance than the latter (mean spans of 3.81 and 5.10).
Overall, these observations lent strong support to the main assumptions of the time-based resource-sharing model. First, time appears to play a major role in determining the impact of the processing component on the concurrent maintenance of information, but in a subtle manner. For example, reducing the total duration of the processing component did not lead to better but to poorer recalls when the amount of stimuli to be processed is kept constant. Keeping this total duration constant led to poorer recalls when the amount of processing was increased. The linear trend reported in Figure 4.2made clear that as we hypothesized, what matters is the proportion of time during which the processing component captures attention and impedes the retrieval and refreshment of the decaying memory traces. Second, the smooth decrease in span when the difficulty of the processing component increases suggests that there is a quasi perfect trade-off between processing and storage, exactly as the resource-sharing theoretical framework predicts. Taken together, these two points suggest that the resource-sharing that occurs between processing and storage is time-based.
Though these first results were in line with our theory, several counterintuitive claims still needed to be tested. For example, one of the predictions of our theory is that any simple task that captures attention can disrupt concurrent maintenance as efficiently as a complex activity provided that it has to be performed under sufficient time pressure. The studies reported above demonstrated that time pressure has an effect on recall performance, but they did not directly compare the effect of simple processing under time-pressure with the effect of complex activities such as reading or equation solving. Are simple activities as disruptive as complex tasks used in traditional span tasks? The next section addresses this question.
Is cognitive load a matter of complexity?
Intuitively, reading sentences for comprehension or solving complex equations is more demanding than identifying letters or browsing in the numerical chain by adding or subtracting one. (p.67)Indeed, we often experience the former activities as laborious, requiring all our attention, and we are well aware of many possible mistakes. By contrast, we rarely experience the latter activities as arduous but instead as undemanding, nearly automatic, the correct answer coming into our mind without any apparent effort. Cognitive psychology echoes these intuitions based on introspective experiences through the widespread notions of controlled and automatic processes, this opposition underpinning most of the theoretical constructs related to working memory. On the one hand, reading sentences and solving equations undoubtedly pertain to those activities that require selection of relevant information among the flow of incoming stimuli and inner knowledge, to select the appropriate and inhibit the irrelevant strategies, and to control their process, that is activities that are known to involve the central executive and to consume cognitive resources (Engle et al. 1999a). On the other hand, identifying letters or browsing in the numerical chain probably rely on direct and automatic retrievals from long-term memory that are often considered as leaving the pool of resources intact (Rosen and Engle 1997). However, according to the time-based resource-sharing model, this distinction would only hold when considered in a temporal vacuum. When taking time into account, reading letters or adding 1 to small numbers could become as demanding and consume the same amount of cognitive resource as reading sentences and solving equations! Should we really believe that the former activities can produce the same disruptive effect on concurrent maintenance in short-term memory as tasks that we experience as being so demanding?
This was tested by Lépine, Bernardin and Barrouillet (2005). The authors compared recall performance in either self-paced traditional working memory span tasks involving complex processing components or new computer-paced tasks involving processing components as simple as those we evoked above. They predicted that simple processing components presented at a slow and comfortable pace should have a low impact on concurrent maintenance, thus resulting in higher spans than the complex processing component involved in traditional working memory span tasks. However, according to the time-based resource-sharing model, they predicted that the difference in spans should disappear when simple processing components have to be performed at a fast pace.
In a first experiment, they used a traditional operation span task inspired from Turner and Engle (1989) in which participants were asked to remember series of letters, each letter being followed by an equation to be verified (e.g., ‘6 + 7 + 2 = 13?’). The equations remained on screen until the participant pressed one of the two keys identified as ‘true’ or ‘false’ on keyboard without any limit of time. This task was compared with the continuous operation span task, first introduced by Barrouillet, Camos and Bernardin (2001; see also Barrouillet et al. 2004), in which each equation of the former task was replaced by a root (a number from 1 to 9) followed by a series of sign operand pairs (i.e., + 1 or − 1). Such a series can for example take the following form: 5 / + 1 / + 1 / − 1. Participants were asked to read aloud the root, the sign-operand pairs, and to give all the answers aloud (i.e., ‘five, plus one six, plus one seven, minus one six’). In the slow-paced condition, participants had 2 s to process each sign-operand pair, but only 1 s in the fast-paced condition. Many studies in cognitive arithmetic have established that adding or subtracting one to small numbers just involves direct retrievals of the answer from memory, retrievals that have been described as automatic (Aschcraft and Battaglia 1978; Barrouillet and Fayol 1998; LeFevre, Bisanz and Mrkonjic 1988). The authors were thus not surprised to observe that the slow-paced condition resulted in higher spans than the traditional operation span task (3.65 and 2.30 respectively). However, and as the time-based resource-sharing model predicted, when the participants were subjected to a fast pace, their continuous operation span dramatically dropped to the same level as the operation span (Table 4.1).
However, the authors acknowledged that this result was not so surprising. As we stated above, the cognitive demand of complex activities such as equation solving lies on the time pressure (p.68)
Table 4.1 Mean spans (and standard deviations) as a function of the nature of the processing component involved in the working memory span tasks
Nature of the processing component | |||
---|---|---|---|
Simple computer-paced tasks | Complex self-paced tasks | ||
Slow | Fast | ||
Operation solving | 3.65 | 2.30 | 2.30 |
(0.92) | (0.78) | (0.81) | |
Reading | 4.17 | 3.11 | 3.38 |
(1.00) | (0.93) | (0.78) |
Adapted with permission from Lépine, R, Bernardin, S and Barrouillet, P (2005). Attention switching and working memory spans. European Journal of Cognitive Psychology, 17, 329–346.
In a further experiment, they compared a reading span task inspired from Daneman and Carpenter (1980) in which participants were asked to read aloud and evaluate the plausibility of sentences displayed on screen (e.g., ‘A cow lays eggs’) with a reading letter span task in which the processing component consisted in reading series of letters displayed successively on screen at a fixed pace. In both tasks, each sentence or series of letters was preceded by a to-be-remembered number presented on screen. Of course, identifying letters is one of the elementary components of reading, but reading series of letters even under time pressure can not be considered as mimicking the activity of reading comprehension. Analyzing the latter activity, Siegel (1994) pointed out that triggering grapheme–phoneme conversion rules, retrieving information about word meaning and processing syntax are cognitive processes involving executive components, all activities that are obviously not needed to read letters. Nonetheless, the authors made the same predictions as in the first experiment because reading letters require attention and a sufficiently fast-paced presentation would prevent attentional switching. In line with this prediction, they observed that the traditional reading span was lower than the reading letter span only when letters were presented at a slow rate (one letter every 1300 ms), but this difference disappeared when letters were presented at a rate of one letter every 675 ms (Table 4.1).
This last result demonstrated that an activity that does not require any memory load or algorithmic process but only the retrieval of overlearned information from memory such as reading letters is sufficient to disrupt concurrent maintenance. As Barrouillet and Camos (2001) surmised, even a fairly simple task that continuously captures attention has the same detrimental effect on span as the complex activities involved in the traditional working memory span tasks. Thus, even if complex activities often involve a high cognitive load, cognitive load is not a matter of complexity. Lépine, Bernardin and Barrouillet (2005) noted that it could be considered as (p.69) surprising that the simple activities they used in their computer-paced tasks have so great an effect on concurrent maintenance because these activities have been considered as automatic and non-demanding. It has often been suggested that solving simple additions and subtractions used in the continuous operation span task would rely on a process of direct and automatic retrieval of the answer from memory (LeFevre et al. 1988; Winkelman and Schmidt 1974; Zbrodoff and Logan 1986). However, they also noted that it had already been pointed out by Kahneman (1973) that a conception of the cognitive demand conflated with complexity was probably wrong. Kahneman reported that when measuring mental effort by arousal and pupillary dilations, easy tasks like the recall of thoroughly overlearned information or retaining five digits for immediate recall induced larger pupillary dilation than apparently more complex activity. More importantly, Kahneman noted that the amount of effort that is required to perform a task could not merely depend on intrinsic characteristics of this task because it is also obviously determined by the rate at which it is performed. The present results confirmed this supposition: even simple tasks can become as, and probably more, demanding than activities considered as complex.
Beyond predicting this counterintuitive result, the time-based resource-sharing model accounts for the fact that we do not usually experience simple activities as demanding. Remember that what matters is time. One of the main properties of the simple tasks we use (identify a letter, finding the answer of 4 + 1) is to extend over very short periods of time. Thus, they can easily be inserted in any activity without interrupting the thread of one’s thoughts. These activities capture our attention for such short periods of time that, most of the time, we fail to notice them. They are in fact so fast that we have the impression of performing them in parallel with other more complex activities that obviously solicit our attentional capacities. However, this is an illusion, as their effect on concurrent activities when performed under time pressure made clear.
Although this study demonstrated that our computer-paced tasks can become as difficult as traditional working memory span tasks involving complex activities, an important issue concerning these tasks remained to be addressed. The main interest of the complex span tasks is not that they are more difficult than simple span tasks, or that they allow us to improve our understanding of working memory functioning, but that they provide us with spans that are good predictors of high-level cognition and academic achievement. However, do the spans provided by our new tasks have the same properties as the traditional reading or operation spans? After all, it remains possible that, contrary to the complex activities of reading sentences and solving equations, the simple activities we use disrupt maintenance by affecting peripheral and unimportant processes. In this case, the spans collected through the new tasks would not reflect individual’s cognitive capacities and would not provide valid measure of working memory capacity, validity being judged by the correlation between the measure and higher level measures of cognition (Engle, Tuholski, Laughlin et al. 1999). In other words, our tasks could lack the predictive power that makes working memory span tasks important for psychology.
Are the new time-constrained tasks as predictive of high level cognition as the traditional working memory span tasks?
This question was addressed by Lépine, Barrouillet and Camos (2005). The authors noted that two alternative hypotheses could be put forward to account for the well-known relationship between working memory spans and performance on high level cognitive activities. According to the first hypothesis, traditional working memory tasks would evaluate some general cognitive capacity that our model describes as an attentional capacity involved in any cognitive process requiring access and maintenance of items of knowledge. This limited attentional capacity would thus underpin and constrain most of the processing steps of the complex activities involved in (p.70) high-level cognition, such as reasoning or problem-solving. According to this view, complexity is an unnecessary characteristic of the processing component of a valid working memory task because what is required is an activity that solicits and captures attention. Moreover, the temporal constraints of our computer-paced tasks hamper the use of possible strategies for coping with the demands of the dual-task paradigm. It is worth to note that these strategies are allowed by most of the traditional self-paced working memory tasks in which participants are free to interrupt and resume their activity as they wish (Baddeley, Logie, Nimmo-Smith et al. 1985; Case, Kurland and Goldberg 1982; Daneman and Carpenter 1980; Turner and Engle 1989). According to the attentional capacity hypothesis, these strategies may produce biased measures of the fundamental capacity by allowing the most skillful participants to overcome their cognitive limits. Thus, this hypothesis predicts that the spans provided by the new tasks will be more predictive of performance in high-level cognition than the traditional tasks.
An alternative hypothesis would be that the ability to plan and use the strategies described above is the basis of the relation between working memory spans and high-level performance. High working memory span individuals would be those who are better able to plan and monitor their activity in complex situations strategically, achieving better performance in working memory dual tasks and in higher-level cognition. In this account, working memory spans are predictive because the traditional tasks mimic high-level cognitive activities, which require the individual to simultaneously maintain goals and intermediary results and to run complex operations. This hypothesis predicts that the traditional spans will have a greater predictive value because the new tasks involve less strategic factors and involve only elementary processes.
The authors tested these two hypotheses by comparing the correlations between academic achievement of 11-year-old children on the one hand, and working memory spans evaluated either by traditional or new span tasks on the other. The tasks were the same as those used by Lépine, Bernardin and Barrouillet (2005) and described above: that is a reading and an operation span task for the traditional tasks and the reading letter and the continuous operation span task for the new tasks. The level of academic achievement was provided by individual scores from the national academic achievement test that each French sixth grader takes at the beginning of the academic year. This test gives compound scores in literacy and mathematics, as well as a global scholastic score in terms of percentage of success. The results were particularly clear and eloquent (Table 4.2).
Table 4.2 Correlations between the traditional and the new working memory span tasks on the one hand and the scholastic scores on the other for 93 French sixth graders
Working memory tasks | Scholastic score | ||
---|---|---|---|
Literacy | Mathematics | Global | |
Traditional | |||
Reading span | 0.30 | 0.33 | 0.34 |
Operation Span | 0.32 | 0.35 | 0.36 |
Traditional compound score | 0.34 | 0.38 | 0.39 |
Computer-paced | |||
Reading letter span | 0.46 | 0.48 | 0.50 |
Continuous operation span | 0.39 | 0.41 | 0.42 |
New compound score | 0.50 | 0.52 | 0.54 |
Adapted from Lépine, R, Barrouillet, P and Camos, V (2005). What makes working memory spans so predictive of high-level cognition? Psychonomic Bulletin and Review, 12, 165–170, Copyright Psychonomic Society Inc.
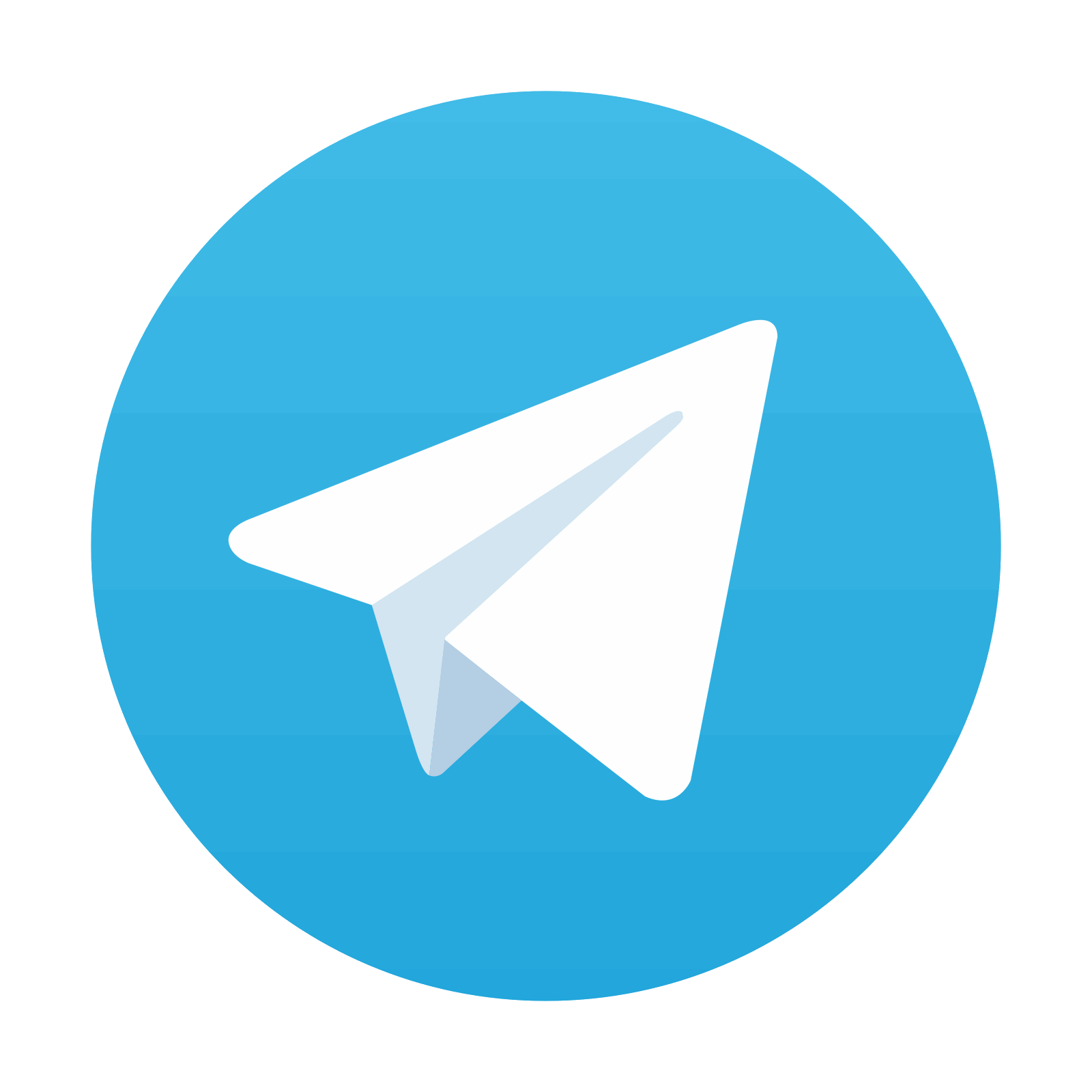
Stay updated, free articles. Join our Telegram channel

Full access? Get Clinical Tree
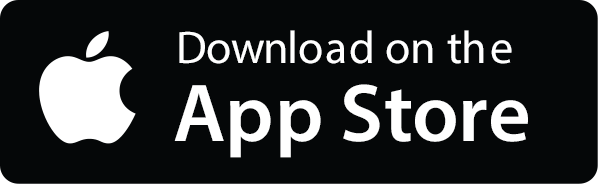
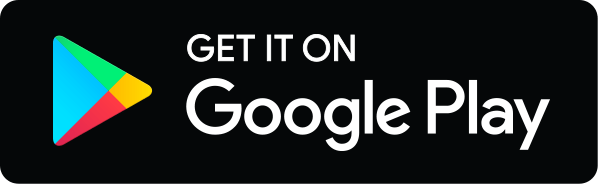