CLINICAL CASE | One-and-One-Half Syndrome
A 30-year-old woman suddenly developed double vision that became worse upon looking to the right. She also indicated that she was unable to look to her left. On examination, when asked to look to the left, her eyes remained fixed forward, as she described. She was unable to abduct the left eye, and the right eye, which normally adducts on looking to the left, remained fixed forward (Figure 12–1A). When she was asked to look to the right, her left eye did not adduct (Figure 12–1B).
She had an MRI that revealed a mid-pontine lesion located close to the midline, just under the floor of the fourth ventricle (Figure 12–1B1). Normal MRI is shown in Figure 12–1B2, and a myelin-stained section through the pons is shown in Figure 12–1B3. In addition to this lesion, the patient had additional white matter lesions. Based on these neurological and radiological signs, as well as additional laboratory tests, the patient was diagnosed with multiple sclerosis.
Based on your reading of this chapter, you should be able to answer the following questions.
1. Interruption of which components of the eye movement control circuit in this patient leads to the inability to look to the left?
2. Why does the left eye fail to adduct on looking to the right?
Key neurological signs and corresponding damaged brain structures Loss of ability to gaze to the leftThe lesion included the left abducens nucleus. This damaged abducens muscle motor neurons, thereby paralyzing that muscle. Adduction of the right eye was also absent. This is because the muscle control signals to look to the left originate in the left pons (Figures 12–1C and 12–7). When we want to look to the left, the paramedian pontine reticular formation, which receives commands from the cortex, sends signals to the left abducens nucleus. There are two neuron classes there: abducens motor neurons, which innervate the abducens muscle, and internuclear neurons, which project into the right MLF to command right medial rectus motor neurons to contract the medial rectus muscle to adduct the right eye. The lesion damages these neurons, as well as the motor neurons. Note that there is slight asymmetry to the left eye, showing a small amount of adduction, due to paralysis of the left lateral rectus muscle and the unopposed pulling of the intact right medial rectus muscle. Since multiple sclerosis is an inflammatory demyelinating condition, neurons are likely not degenerated but functionally impaired.
Inability to adduct the left eye on looking to the rightThe lesion includes the MLF on the left side. Internuclear neurons from the opposite abducens nucleus project their axons into this MLF to reach left medial rectus motor neurons. Note that typically there is nystagmus, an abnormal oscillation or bobbing, of the abducting eye (see Figure 12–13).
ReferencesBrust JCM. The Practice of Neural Science. New York, NY: McGraw-Hill; 2000.
Espinosa PS. Teaching NeuroImage: one-and-a-half-syndrome. Neurology. 2008;70:e20.
Shintani S, Tsuruoka S, Shiigai T. One-and-a-half syndrome associated with cheirooral syndrome. Am J Neuroradiol. 1996;17:1482-1484.
Uesaka Y, Nose H, Ida M. The pathway of gustatory fibers in the human ascends ipsilaterally. Neurology. 1998;50:827.
Wall M, Shirley HW. The one-and-a-half syndrome. A unilateral disorder of the pontine tegmentum: a study of 20 cases and review of the literature. Neurology. 1983;33:971-980.
FIGURE 12–1
One-and-one-half syndrome. A. Top. Position of eyes when the patient is attempting to look to her left. Note that both eyes are fixed forward. Bottom. Position of eyes when patient is attempting to look to her right. The right eye abducts, but the left eye is fixed forward; there is no left eye adduction. B. Images of the pons. B1. MRI (FLAIR) of patient, showing lesion (bright region) (Reproduced with permission from Espinosa PS. Teaching NeuroImage: One-and-a-half-syndrome. Neurology. 2008;70[5]:e20.) B2. Normal MRI. B3. Myelin-stained section showing the locations of key structures and the approximate location of the lesion. C. Ventral brain stem view showing the circuit for horizontal gaze. The red ellipse indicates the extent of the lesion, which disrupts the left abducens nucleus and left MLF. (B1, Reproduced with permission from Espinosa PS. Teaching NeuroImage: One-and-a-half-syndrome. Neurology. 2008;70[5]:e20. B2, Courtesy of Dr. Joy Hirsch, Columbia University.)

During takeoff in a jet you experience a particularly salient function of the vestibular system: sensing body acceleration. Although perception of signals from the vestibular sensory organs occurs only under special circumstances, this system is operating continuously by controlling relatively automatic functions, such as maintaining balance when we are walking on uneven terrain or adjusting blood pressure when we stand up quickly. The vestibular system shares many brain stem circuits and functions with the neural systems for controlling the extraocular muscles that move the eyes. Eye movement must be precisely controlled to position the image of an object of interest over the fovea, where visual acuity is best (see Chapter 7). The vestibular and oculomotor systems coordinate the head and eyes during head movement. Consider your ability to maintain gaze on a friend’s face in a crowded airport terminal as you run toward her. Your head bobs up and down, and from side to side, but you can maintain fixation effortlessly. The vestibular system detects your head motion, and the oculomotor system makes compensatory eye movements to stabilize the image of your friend on the retina. The actions of the two systems are coordinated automatically by the vestibuloocular reflex. In addition, the medial descending motor pathways (see Chapter 10) assist in this action by adjusting head position by controlling neck muscles. This happens without conscious awareness, apart from knowing where to fixate your gaze.
From a clinical perspective, the vestibular and eye movement control systems are also very tightly linked. An important part of testing the integrated functions of the brain stem involves careful assessment of a person’s ability to coordinate eye movement during head motion. Assessment of vestibuloocular reflexes is an important part of examination of the comatose patient. This chapter also continues the examination of the cranial nerve nuclei, through which greater knowledge of brain stem regional anatomy will emerge, and the descending motor pathways. Such knowledge is essential for clinical problem solving, for example, in understanding behavioral deficits and identifying the locus of central nervous system damage after a stroke. Because each of the cranial nerve nuclei has a clearly identifiable sensory or motor function, the clinician can thoroughly test the integrity of such functions.
Vestibular receptors sense head motion, both linear—such as that experienced during fast acceleration in an elevator or a jet—and angular—such as during turning. These receptors are located in five peripheral vestibular organs (Figure 12–2, inset): the three semicircular canals, which signal angular acceleration, and the utricle and saccule, which signal linear acceleration. Vestibular receptors are hair cells, which are innervated by bipolar neurons whose cell bodies are located in the vestibular ganglion. The axons of these bipolar neurons travel to the brain stem in the vestibular division of cranial nerve VIII and terminate in the vestibular nuclei. There are four separate vestibular nuclei: inferior, medial, lateral, and superior (Figure 12–2). The vestibular system comprises distinctive circuits that have the following major functions, each considered below: (1) perception, (2) blood pressure regulation, and (3) descending control of proximal and axial muscles. The vestibular system has a fourth major function in eye movement control; this is considered in the next section, on gaze control.
An Ascending Pathway From the Vestibular Nuclei to the Thalamus Is Important for Perception, Orientation, and Posture
Originating primarily from the superior, medial, and inferior vestibular nuclei, the thalamic path ascends bilaterally to several sites within and around the ventral posterior nucleus (Figure 12–3B). Three major sites within the parietal lobe and insular cortex receive vestibular information (Figure 12–3A). The vestibular cortex in the (1) retroinsular cortex and (2) posterior parietal lobe play roles in conscious awareness of vestibular sensory activation and in sensing body orientation and the orientation of the world around us. A separate region, (3) area 3a (part of the primary somatic sensory cortex) is thought to participate in sensing head position in conjunction with proprioceptive afferents in neck muscles (see Chapter 4). Each of these cortical regions is also involved in controlling proximal muscles and posture, not directly like the corticospinal tract, but rather through their descending connections with vestibulospinal tract neurons, an indirect corticovestibulospinal tract.
Blood pressure regulation is an integrated response involving primarily heart rate and vascular smooth muscle control. When we sit up quickly, blood must now flow against gravity. Maintenance of adequate blood flow to the brain is accomplished by a pressor reflex response, in which there are compensatory increases in heart rate and vascular smooth muscle tone. These responses are mediated by the autonomic nervous system (see Chapter 15). When this response is inadequate, such as when a person is taking certain medications for reducing blood pressure or diuretics, orthostatic hypotension can result. Vestibular regulation of blood pressure is accomplished through connections with the brain stem visceral integrative centers—the solitary, vagal, and parabrachial nuclei—that, in turn, regulate autonomic nervous system function (Figure 12–3A; see Chapters 6 and 15).
FIGURE 12–3
A. General organization of the vestibular system revealed in cross section at different levels through the brain stem and in coronal section through the diencephalon and cerebral hemispheres. B. The medial and lateral vestibulospinal tracts, together with the descending corticovestibular projection. The inset is a lateral view of the cerebral hemisphere, showing the locations of three key areas that receive vestibular inputs from the thalamus.

The Vestibular Nuclei Have Functionally Distinct Descending Spinal Projections for Axial Muscle Control
For axial muscle control, the vestibular nuclei receive information primarily from the cerebellum and cerebral cortex. The vestibular nuclei have two functionally distinct descending projections for balance and coordinating head and eye movements: the lateral and medial vestibulospinal tracts. These two descending motor pathways form a major portion of the medial descending motor pathways. The lateral vestibulospinal tract, which begins at the lateral vestibular nucleus, descends ipsilaterally in the white matter to all spinal levels (Figure 12–3C). This pathway is crucial for controlling posture and balance, which involves neck, back, hip, and leg muscles. Recall from Chapter 10 that even though a particular tract may have a unilateral projection, the medial descending pathways collectively exert a bilateral influence on proximal and axial muscle control because they synapse on commissural neurons (see Figure 10-16A). The medial vestibulospinal tract, which starts primarily at the medial vestibular nucleus, descends bilaterally in the white matter but only to the cervical and upper thoracic spinal cord (Figure 12–3B). The medial vestibulospinal tract plays a role in controlling head position in relation to eye position.
The position and movement of the eyes are controlled voluntarily and by vestibular reflexes. There are five types of eye movements:
Vestibuloocular reflexes use information from the semicircular canals to compensate for head motion by automatically adjusting eye position to maintain the direction of gaze.
Saccades are rapid movements that shift the fovea to an object of interest.
Smooth pursuit movements are slow and are used for tracking a moving object.
Vergence movements (either convergent or divergent) ensure that the image of an object of interest falls on the same place on the retina of each eye.
Optokinetic reflexes use visual information to supplement the effects of the vestibuloocular reflex.
With the exception of vergence, all eye movements are conjugate: The eyes move in tandem at the same speed and in the same direction. Vergence movements are disconjugate; the eyes move in opposite directions.
Each eye is controlled by six muscles, which operate as three functional pairs, with antagonistic mechanical actions (Figure 12–4A). The lateral and medial rectus muscles move the eye horizontally, abducting (looking away from the nose) and adducting (looking toward the nose), respectively. The superior and inferior rectus muscles elevate and depress the eye, particularly when the eye is abducted. Finally, the superior and inferior oblique muscles depress and elevate the eye, especially when the eye is adducted. Other actions of the extraocular muscles are indicated in Figure 12–4B.
FIGURE 12–4
A. The two eyes with the various extraocular muscles and their innervation patterns. Not shown is the levator palpebrae, an eyelid elevator innervated by cranial nerve III. The extraocular muscles of both eyes operate as three functional pairs. The lateral and medial rectus muscles move the eye horizontally. The superior and inferior rectus muscles elevate and depress the eye, respectively (particularly when the eye is abducted). Finally, the inferior and superior oblique muscles elevate and depress the eye but to a greater extent when the eye is adducted. B. The mechanical actions of the extraocular muscles.

The oculomotor nucleus contributes most of the axons of the oculomotor (III) nerve, which exits the rostral midbrain. The oculomotor nucleus (Figure 12–5) innervates four of the six extraocular muscles: medial rectus, inferior rectus, superior rectus, and inferior oblique (Figure 12–4). This nucleus also innervates the levator palpebrae superioris muscle, an eyelid elevator. (A contingent of autonomic nervous system axons travels in the oculomotor nerve to innervate smooth muscle; see Chapter 15.)
FIGURE 12–5
Extraocular muscle control. A. View of the dorsal brain stem, showing the locations of the oculomotor, trochlear, and abducens nuclei and depicting the course of the trochlear nerve within the brain stem. B. Transverse sections through the oculomotor, trochlear, and abducens nuclei. Axon of internuclear neuron travels in the contralateral medial longitudinal fasciculus.

The other two extraocular motor nuclei are the trochlear and abducens nuclei (Figure 12–5). Motor neurons in the trochlear nucleus give rise to the fibers in the trochlear (IV) nerve, which innervate the superior oblique muscle (Figure 12–4). This cranial nerve is the only one that exits from the dorsal brain stem surface. The trochlear nerve is further distinguished because all of its axons decussate within the central nervous system. The abducens nucleus (Figure 12–5) contains the motor neurons that project their axons to the periphery through the abducens (VI) nerve. Abducens motor neurons innervate the lateral rectus muscle (Figure 12–4). Unlike spinal and other cranial nerve motor neurons, extraocular motor neurons are not controlled by the primary motor cortex.
Stable fixation can be maintained on an object during head movement because the vestibular system generates eye movement control signals that compensate for head movements. For example, horizontal rightward movement of the head generates leftward conjugate movement of the eyes (Figure 12–6A). This movement is produced by excitation of left lateral rectus motor neurons and right medial rectus motor neurons. The lateral and medial rectus motor neurons are directly activated by vestibular neurons (Figure 12–6B), demonstrating the importance of automatic control of eye movement by head movement. In addition, the medial rectus motor neurons are indirectly activated by the internuclear neurons in the left abducens nucleus (Figure 12–6B, thin line). Although not shown in Figure 12–6B, the circuit for vestibuloocular control also ensures that the mechanical action of the muscle that moves the eye, termed the agonist muscle, is not impeded by contraction of the antagonistic muscles (the muscles whose mechanical action is opposite that of the agonist muscle). This process occurs through inhibitory connections with the motor neurons of antagonistic muscles. For example, when the left lateral rectus motor neurons are excited, the left medial rectus motor neurons are inhibited.
Voluntary Eye Movements Are Controlled by Neurons in the Frontal Lobe and the Parietal-Temporal-Occipital Association Cortex
Saccades are triggered by neurons in the frontal eye field, a portion of cytoarchitectonic area 8 (see Figure 2–19). This cortical territory receives subcortical inputs from the basal ganglia and cerebellum, transmitted via thalamic neurons. The frontal eye field projects to the superior colliculus (Figure 12–7A). The axons of these frontal lobe projection neurons descend within the anterior limb and genu of the internal capsule to the brain stem. Superior colliculus neurons, in turn, project to distinct regions of the pontine and midbrain reticular formation that directly control saccades through their monosynaptic connections to extraocular motor neurons. The frontal eye fields also project directly to these two reticular formation zones. For controlling horizontal saccades, the frontal eye fields project to neurons in the paramedian pontine reticular formation (Figure 12–7A, B). These neurons process control signals and, in turn, project to the abducens nucleus. The abducens nucleus is more than a motor nucleus because, in addition to containing lateral rectus motor neurons, it also contains internuclear neurons (Figure 12–7B). Signals from the paramedian pontine reticular formation trigger horizontal saccades by directly exciting lateral rectus motor neurons in the abducens nucleus and, via the internuclear neurons, indirectly exciting medial rectus motor neurons in the oculomotor nucleus. For vertical saccades, the frontal eye fields project to the rostral interstitial nucleus of the medial longitudinal fasciculus in the midbrain reticular formation (Figure 12–7A). Neurons in this nucleus coordinate the muscles that produce vertical eye movements (Figure 12–5B). A portion of the posterior parietal cortex within area 7 participates in saccade generation through its role in visual attention: You must first attend to a stimulus before looking at it. This region projects through the posterior limb of the internal capsule to the superior colliculus.
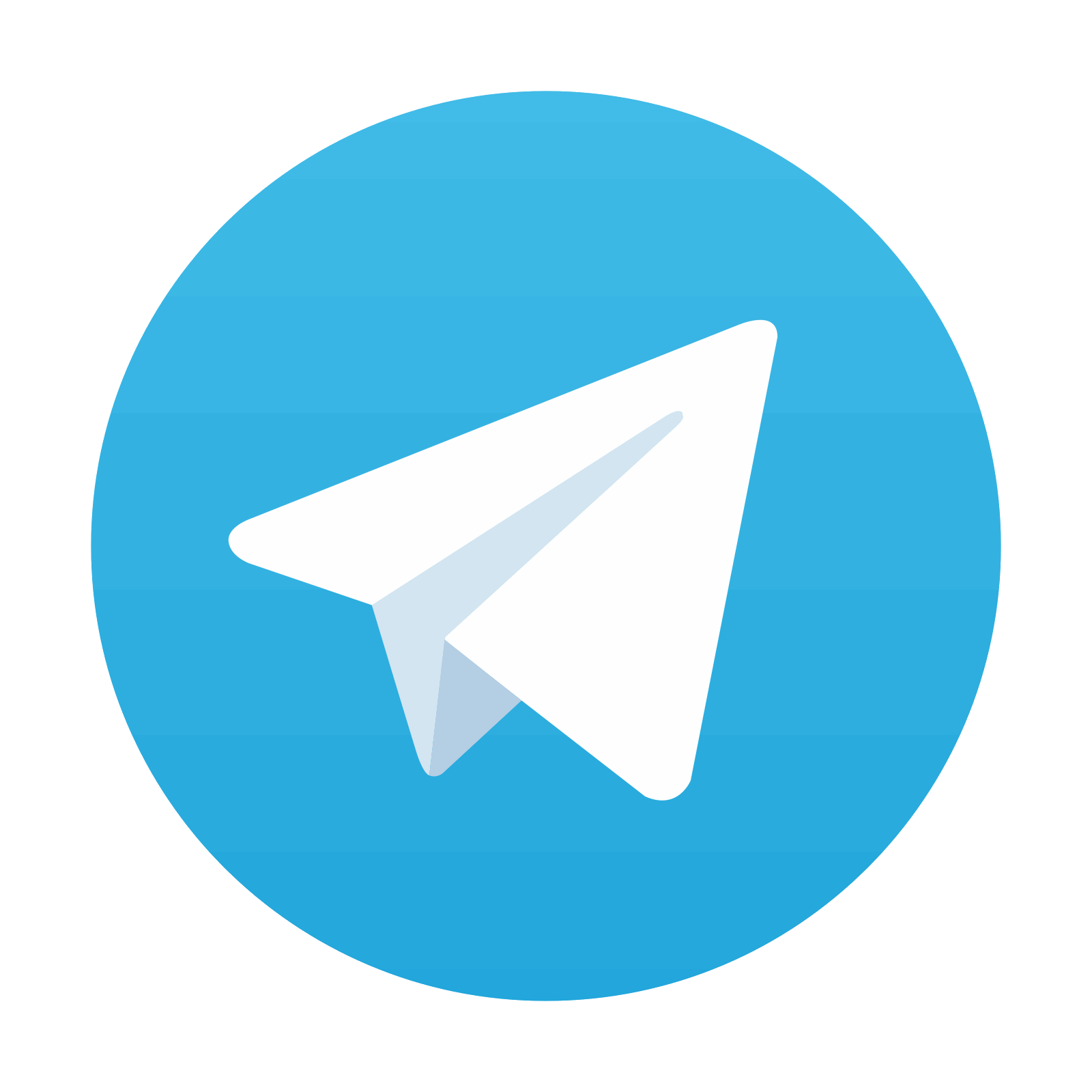
Stay updated, free articles. Join our Telegram channel

Full access? Get Clinical Tree
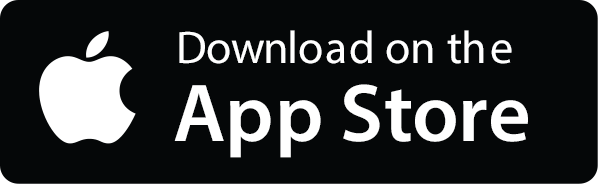
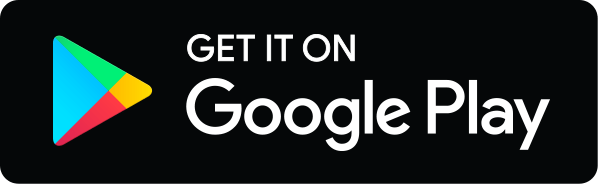