Therapeutic Potential of Melatonin in Sleep and Circadian Disorders
Irina V. Zhdanova
Leah Friedman
Melatonin (N-acetyl-5-methoxytryptamine) is a phylogenetically primitive molecule, found in such diverse species as unicellular organisms, plants, and vertebrates. In vertebrates, the main source of melatonin is the pineal gland (epiphysis cerebri). This small structure, located in approximately the center of the human brain, has fascinated anatomists and philosophers for over two millennia. It is believed to have been first described around 300 B.C. by Herophilous, an Alexandrine Greek physician, and it received its name, pineal organ, from Greek anatomist Galen (130 to 200 A.D.), to whom its shape resembled a pinecone. Galen had thought that this unpaired structure near the base of the third ventricle served as a valve to regulate the flow of thought. Expanding on Galen’s ideas, the French philosopher René Descartes (1596 to 1650) located psychic activity in the pineal gland, considering it “the seat of imagination and common sense” (1). In light of our modern knowledge about the pineal gland, it is remarkable that Descartes linked its function with the optic nerves and eyes (Fig. 9.1). Since all early conceptions of the physiologic role of the pineal organ had little scientific data to support them, it was primarily considered a vestigial appendix of the brain throughout the 18th and 19th centuries.
At the beginning of the 20th century, a study by McCord and Allen (2) showed that mammalian pineal tissue contained a substance that caused the skin of tadpoles to blanch. In the 1950s, Aaron Lerner, a professor of dermatology at Yale University, became interested in this effect, hoping that such a substance could help in treating vitiligo, a human skin pigmentation disorder. Pursuing this goal, Lerner et al. (3) identified an active compound of the pineal gland responsible for a blanching effect in amphibians and coined the name melatonin. Although melatonin proved to be without effect on human skin pigment distribution, Lerner was the first to observe and report a sedative effect of the pineal hormone in man.
Since then, studies conducted in dozens of laboratories all over the world have taught us much about melatonin, although many questions with regard to its role in human and animal physiology remain either unanswered or have not been addressed. Yet, the widely publicized claims about melatonin’s multiple positive effects and the fact that this hormone received the label “food supplement” have encouraged millions of people to consume melatonin on a daily basis without medical control and in quantities that increase circulating hormone levels 10- to 50-fold over those that normally occur in human blood.
The goal of this chapter is to present both basic and clinical information about melatonin to help promote understanding of its role in supporting the physiologic harmony of the organism and the importance of the appropriate use of melatonin therapy.
Melatonin—a Key Hormone of the Circadian Photoneuroendocrine Axis
All the major structures of the human circadian system, including the eyes and the suprachiasmatic nuclei (SCN) of the hypothalamus and the pineal gland, develop from the roof of the diencephalon. They are involved in the perception and/or translation of photic information,
and thereby facilitate an organism’s adaptive adjustment to rhythmic changes in environmental illumination due to the earth’s daily rotation on its axis. A near-24-hour (circadian) rhythm of melatonin secretion from the human pineal gland depends on a periodic signal from the SCN, the master biologic “clock.” The neurons of this small brain structure are normally active during the day and slowdown at night, and are capable of sustaining a circadian pattern of activity even in the absence of rhythmic environmental input. The activation of SCN neurons has an inhibitory effect on the pineal gland, defining a nocturnal pattern of melatonin secretion. If SCN neurons are activated at night, e.g., by environmental light perceived by the retina, melatonin production declines. Melatonin, in turn, can attenuate the activity of the SCN. This melatonin action is likely to support a normal decline in the activity of the SCN at night, further promoting melatonin secretion and contributing to an overall increase in the amplitude of circadian body rhythms.
and thereby facilitate an organism’s adaptive adjustment to rhythmic changes in environmental illumination due to the earth’s daily rotation on its axis. A near-24-hour (circadian) rhythm of melatonin secretion from the human pineal gland depends on a periodic signal from the SCN, the master biologic “clock.” The neurons of this small brain structure are normally active during the day and slowdown at night, and are capable of sustaining a circadian pattern of activity even in the absence of rhythmic environmental input. The activation of SCN neurons has an inhibitory effect on the pineal gland, defining a nocturnal pattern of melatonin secretion. If SCN neurons are activated at night, e.g., by environmental light perceived by the retina, melatonin production declines. Melatonin, in turn, can attenuate the activity of the SCN. This melatonin action is likely to support a normal decline in the activity of the SCN at night, further promoting melatonin secretion and contributing to an overall increase in the amplitude of circadian body rhythms.
Melatonin can also produce a shift in the circadian phase of SCN activity, by either advancing or delaying it. The direction of the phase shift depends on the time of melatonin treatment, i.e., administration of melatonin in the late afternoon can advance the circadian clock, while early morning treatment can cause a phase delay (4).
A temporal and functional interplay between melatonin and the SCN, and their response to environmental light, promote a temporal alignment of multiple circadian body rhythms with each other (internal synchronization) and with the periodic changes in the environment (external synchronization).
Melatonin Production and Metabolism
The 24-hour pattern of melatonin production in humans (Fig. 9.2) is characterized by a nocturnal increase. It is typically initiated about 2 hours before an individual’s habitual bedtime, unless blocked by environmental light, and declines in the morning. This rhythmic pattern of melatonin secretion persists in people maintained in constant darkness. Exposure to darkness during the daytime does not induce melatonin production; however, the abrupt imposition of bright light at night can suppresses it.
The circulating amino acid L-tryptophan is the precursor of melatonin (Fig. 9.3). Within cells in the pineal gland it is converted to serotonin (5HT) by a two-step process, catalyzed by the enzymes tryptophan hydroxylase (TH) and 5-hydroxytryptophan decarboxylase. This process involves serotonin’s N-acetylation, catalyzed by N-acetyltransferase (NAT), and then its methylation by hydroxyindole-O-methyltransferase (HIOMT) to produce melatonin. The hormone is released directly into the blood stream and the cerebrospinal fluid as it is synthesized and, being lipid soluble, has ready access to every cell in the body. About 50% to 70% of circulating melatonin is reportedly bound to plasma albumin; the physiologic significance of this binding is not yet known. Inactivation of melatonin occurs in the liver where it is converted to 6-hydroxymelatonin by the P450-dependent microsomal mixed-function oxidase enzyme system. Most of the 6-hydroxymelatonin is excreted in the urine and feces as a sulfate conjugate (6-sulfatoxymelatonin), and a much smaller amount as a glucuronide. Some melatonin may be converted to N-acetyl-5-methoxykynurenamine in the central nervous system. About 2% to 3% of the melatonin produced is excreted unchanged in the urine.
Circulating Melatonin Levels in Health and Disease
The human fetus and newborn infant do not produce melatonin but rely on the hormone supplied via placental blood, and, postnatally, via mother’s milk. After infants are 9 to 12 weeks old, rhythmic melatonin production increases rapidly; the highest nocturnal melatonin levels are attained in children under 5 years of age. Thereafter, melatonin levels decrease, with perhaps the most dramatic changes occurring during adolescence (5). Though exceptionally healthy elderly individuals sometimes maintain high melatonin production (6), the majority of aged individuals have low circulating melatonin levels (7, 8, 9). Typical nocturnal peak serum concentrations of melatonin in young humans are around 100 pg per ml, while daytime levels are as low as 1 to 3 pg per ml. Although the circulating melatonin pattern for a given individual tends to remain surprisingly constant from day to day, marked interindividual variations in nocturnal melatonin levels are observed in all age groups (Fig. 9.4).
Some, though not all, studies have found that middle-aged and elderly insomniacs have lower melatonin production than good sleepers of the same age (10). Similarly, a controversial body of data exists on the melatonin levels in psychiatric patients. An early study proposed that low nocturnal melatonin levels could be a trait-dependent marker for major depressive disorder (11); conversely, another study reported that melatonin was elevated during manic states (12). Such trends have been considered a possible reflection of contrast changes in noradrenergic activity in depression and mania, since melatonin production in the pineal gland depends on its sympathetic innervation. Further studies, however, either failed to document significant differences between depressed patients and control subjects or even found higher melatonin levels associated with depression (13,14). Decreased nocturnal melatonin levels were also reported in both drug-free schizophrenics and those treated with antipsychotic drugs (15,16). The inconsistent reports regarding melatonin production in patients with psychiatric disorders might be explained by pronounced interindividual and age-related variability in melatonin levels, a heterogeneity of affective disorders, effects of environmental illumination during test procedures, or relatively small sample sizes. The potential impact of these factors requires rigorously controlled studies to determine whether, indeed, alterations in endogenous melatonin levels might be characteristic of psychiatric disorders.
MELATONIN TREATMENT
Even if extreme changes in circulating melatonin levels are not part of the pathogenesis of age-related insomnia or psychiatric diseases, a number of clinical symptoms characteristic of these disorders, e.g., sleep alterations, circadian rhythms disturbances, and anxiety, might benefit from timely melatonin treatment.
Effects of Melatonin on Sleep
MELATONIN AND SLEEP IN HEALTHY INDIVIDUALS
There are indirect signs of a close relationship between melatonin and sleep in humans. The hours of the increased melatonin secretion are concurrent with the habitual hours of sleep, and the onset of melatonin secretion correlates with the onset of evening sleepiness (17, 18, 19). Studies of circadian phase shifts in humans also show that change in the timing of the onset of melatonin secretion correlates with change in the timing of evening sleepiness, a phenomenon widely observed in shift workers and in transmeridian jet-travelers. An increase in endogenous circulating melatonin levels resulting from infusion of its precursor, tryptophan (20), or a suppression in melatonin metabolism (21) is reported to increase sleepiness and to promote sleep. In contrast, suppression of melatonin production by treatment with beta-blockers disturbs the sleep process (22).
Melatonin treatment is typically found to produce subjective and/or objective sleep-promoting effects in healthy research subjects when administered during the day or to improve overnight sleep in insomniacs (23). Depending on the methods of sleep assessment used and the baseline sleep patterns characteristic of the various populations tested, the effects of melatonin treatment have been described as increased reaction time, diminished subjective alertness, increased fatigue or sleepiness, increased sleep propensity, reduction in latency to sleep onset, decrease in the number of nocturnal awakenings, or an increase in sleep efficiency. These effects are observed in response to doses that induce physiologic (i.e., around 100 pg per ml) and pharmacologic (over 200 pg per ml) circulating levels of the hormone. Remarkably, the effects of both physiologic and pharmacologic doses of melatonin are not accompanied by any dramatic changes in electrophysiologic sleep architecture, a common complication encountered with many existing hypnotics and anxiolytics. Collectively, the available data on the effects of melatonin on sleep suggest that a nocturnal surge in melatonin production may be an important factor in normal human sleep regulation, and that melatonin deficiency might contribute to an altered sleep pattern.
MELATONIN TREATMENT IN GERIATRIC POPULATION
Persistent insomnia in older patients is strongly associated with depressed mood, while improvement in sleep can positively affect daytime vigilance and performance (24). However, conventional hypnotic drugs, often used in older patients to promote sleep, can cause daytime sedation and impair motor coordination and memory function. For example, amnestic and performance-disruptive effects are reported to parallel the hypnotic effects of the benzodiazepines (25,26). Although the reasons for the substantial increase in mood and sleep disorders found in older humans are not entirely clear, and may involve a number of factors, low amplitude of the circadian signal (27), including decline in melatonin production, may well contribute to altered nighttime sleep quality and low daytime mood and performance. Melatonin treatment, used in both physiologic and pharmacologic doses, can improve sleep in older insomniacs (28, 29, 30, 31). It should be noted, however, that the sleep-promoting effects of melatonin demonstrate substantially higher variability in older individuals compared to young healthy adults, presumably due to age-related changes in the number or sensitivity of
melatonin receptors, or alterations in melatonin metabolism. This finding bears on the important issue of whether, for therapeutic purposes, melatonin doses should be individualized.
melatonin receptors, or alterations in melatonin metabolism. This finding bears on the important issue of whether, for therapeutic purposes, melatonin doses should be individualized.
MELATONIN TREATMENT IN CHILDREN WITH NEUROLOGIC DISORDERS AND MENTAL RETARDATION
Studies in children with severe idiopathic insomnia (32,33) or insomnia associated with multiple neurologic disorders showed that the administration of melatonin could substantially improve their sleep patterns and increase sleep duration (34). Similarly, our study of children with Angelman syndrome (AS), a rare genetic disorder characterized by severe mental retardation, hyperactivity, and disturbed sleep, observed that timely administration of low melatonin doses promoted both nighttime sleep and advanced the circadian rhythm of melatonin production (35). Children suffering from the Asperger disorder, typically associated with sleep alterations, also show improved sleep when treated with melatonin (36).
Melatonin treatment in children with attention deficit hyperactivity disorder (ADHD) and chronic sleep-onset insomnia results in earlier sleep onset and increase in total sleep time, although no significant changes in daytime behavior have been documented (37, 38, 39). This is especially important since ADHD patients typically receive psychostimulants for their primary diagnosis and those may further disturb their sleep. Melatonin can improve sleep in children with epilepsy, another disease often complicated by disturbed nighttime sleep (40).
MELATONIN TREATMENT IN PSYCHIATRIC DISORDERS
Sleep and mood are intimately linked physiologic processes and sleep deprivation in healthy adults results in increased anxiety and/or depression (41). The majority of mood disorders are associated with significant sleep alterations, which often manifest as the first behavioral symptoms of the disease (42). The increase in subjective sleep disturbance may also be the first signal of relapse in remitted depressed patients. Difficulty falling asleep, frequent nighttime awakenings, and nightmares are present in such diverse mental illnesses as depression, schizophrenia, and anxiety disorders. Moreover, individuals with chronic insomnia have significantly higher risks for incident depression (43). Successful treatment of psychiatric symptoms is typically associated with sleep improvement (44), while effective management of insomnia in depressed patients can markedly improve their mood (45). Thus, insomnia might be part of a pathophysiologic complex predisposing to psychiatric disease and/or might be an important factor in precipitating or sustaining alterations in mood and cognitive functions.
Few studies have attempted to use melatonin to treat patients suffering from psychiatric disorders. Such treatment was found to improve sleep in patients with major depression, though it did not significantly affect their mood (46,47). Both sleep and mood were improved by melatonin treatment in patients suffering from winter depression or from mania (48,49). Sleep in schizophrenic patients, experiencing chronic insomnia, was also enhanced by melatonin administration (15). Conversely, no changes after melatonin treatment in sleep or mood were documented in patients with rapid-cycling bipolar disorder (50). All these studies involved relatively small numbers of patients, especially considering the heterogeneity of these disorders. Clearly, more studies are needed to evaluate the efficacy of melatonin treatment for insomnia in psychiatric patients and to compare it to conventional therapy with hypnotic medications.
Effects of Melatonin on Circadian Body Rhythms
Both sleep and mood are under the control of the biologic clock, located in the SCN (51). Alterations in biologic rhythms, lack of their synchronization with each other or with the environment, might result in sleep and mood disorders or exacerbate existing pathologic conditions. The role of melatonin in synchronizing circadian body rhythms or increasing the
amplitude of circadian oscillation suggests that the pineal hormone might have beneficial effects in psychiatric and sleep disorders associated with disruption in body rhythms.
amplitude of circadian oscillation suggests that the pineal hormone might have beneficial effects in psychiatric and sleep disorders associated with disruption in body rhythms.
CIRCADIAN ALTERATIONS IN PSYCHIATRIC DISORDERS
Some, though not all, studies assessing chronobiologic measures in psychiatric patients found increased variability, lower amplitude, and/or internal desynchronization of circadian body rhythms (52, 53, 54, 55). However, it is not clear whether these changes observed in psychiatric patients reflect alterations in their sleep-wake cycle and/or social schedule, result from faulty feedback mechanisms in their hypothalamo-pituitary-adrenal and somatotropic axes, or from changes in their intrinsic circadian clock system.
Some studies in seasonal affective disorder (SAD) patients suggest that this condition is associated with, and perhaps results from, a circadian phase delay (56,57). Melatonin treatment in the afternoon can improve both mood and sleep in patients with SAD (58). Whether the effect on mood in this population results from an observed modest phase shift in patients’ circadian body rhythms or from improved nighttime sleep needs further clarification.
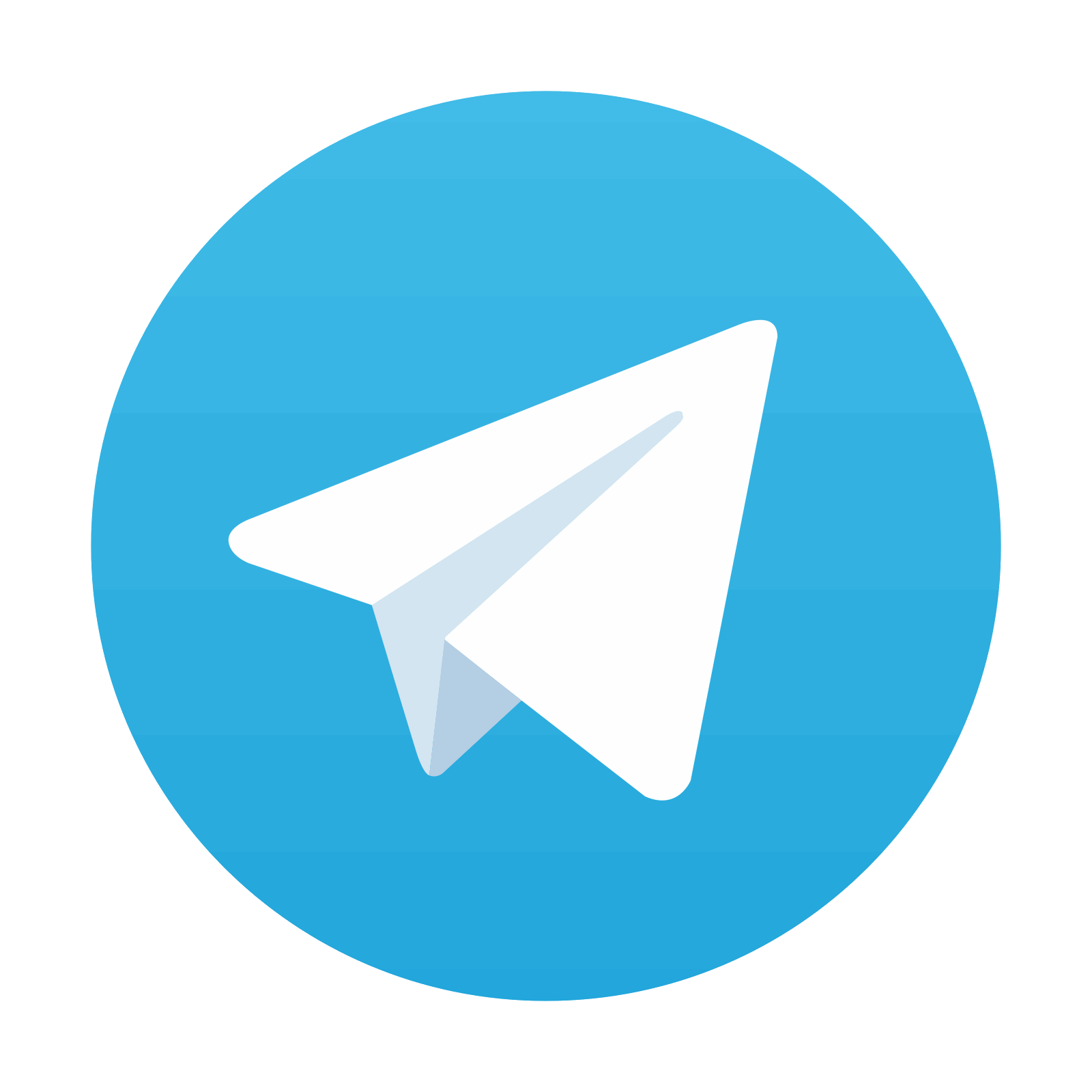
Stay updated, free articles. Join our Telegram channel

Full access? Get Clinical Tree
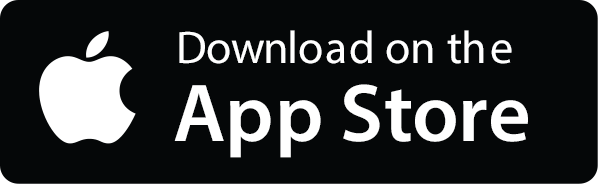
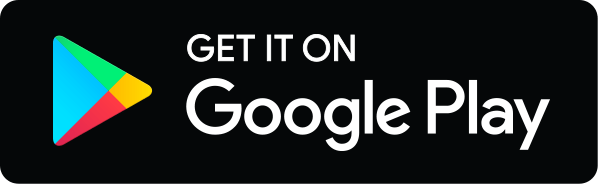
