© Springer International Publishing Switzerland 2016
Thomas Platz (ed.)Therapeutic rTMS in Neurology10.1007/978-3-319-25721-1_1212. Therapeutic rTMS in Neurology: Applications, Concepts, and Issues
(1)
BDH-Klinik Greifswald, Ernst-Moritz-Arndt Universität, Greifswald, Germany
12.2.1 Response Variability
12.2.2 Models of Therapeutic Action
12.2.5 rTMS and Training
12.3 Concluding Remarks
Abstract
rTMS therapy has been shown to generate clinical benefits in a variety of conditions after stroke such as arm and leg paresis, spasticity, dysphagia, aphasia, and neglect, for motor deficits in Parkinson’s disease, for impaired gait and spasticity in incomplete spinal cord injury (SCI) subjects, and for other frequently encountered clinical conditions such as tinnitus and neuropathic pain. The variability of the brain’s response and any clinical effects to rTMS therapy still make it difficult to predict any individual’s response. Nevertheless, the clinical benefits that can be achieved are at times remarkable and favor the clinical application of rTMS therapy. Issues such as the neurophysiological model of action, the selection of the target site, the type, the schedule, and the combinations of rTMS applications, as well as the question of combined rTMS and training therapy, are reflected for the different conditions treated.
12.1 Applications of rTMS in Clinical Neurology
The previous chapters in this book give an overview over conditions where rTMS interventions have been shown to produce clinical benefits. Indeed, in a variety of conditions after stroke such as deficits of arm motor control and leg motor control as well as spasticity, dysphagia, aphasia, and neglect, functional improvements have been documented after rTMS interventions. Further examples are motor deficits in Parkinson’s disease, impaired gait and spasticity in incomplete spinal cord injury (SCI) subjects, and other frequently encountered clinical conditions such as tinnitus and neuropathic pain.
This book provides a state-of-the-art overview to what extent rTMS applications can therapeutically be considered in these areas of clinical neurology, pinpointing both to the encouraging clinical evidence available so far and the limitations of our knowledge asking for caution with regard to introducing rTMS interventions into routine clinical practice. While clinical benefits have at times been impressing, many questions still remain unanswered.
The aim of this chapter is to reflect some of the methodological and clinical reasoning that can be deduced from the evidence portrayed in this book and to address some of the questions that need further attention before rTMS interventions can be introduced in clinical practice in a more widespread manner.
12.2 Issues to be Considered for Scientific and Clinical Reasoning
12.2.1 Response Variability
For clinical decision-making, the variability of the brain’s response and any behavioral effects to rTMS applications cause the problem that it is difficult to predict any individual’s response.
One reason for the observed variability might be that TMS impulses activate many different synapses, both of excitatory and inhibitory neurons in the cortex (Di Lazzaro and Rothwell 2014). Further, rTMS can affect learning processes in a facilitatory way or as suppression. Different functional networks might again respond differently to comparable rTMS interventions. Age, gender, time of day, physical activity, prior history of synaptic activity, and genetics have all been shown to account for the variability responses to TMS impulses of the cortex (Ridding and Ziemann 2010).
One way to deal with the fact of intersubject variability is to test the effects of different rTMS approaches in single subjects and only then to engage in a series of applications for the individually most effective approach. The selection could both be based on individual behavioral data and individual neurophysiological data such as motor evoked potentials (MEP) or TMS-induced EEG changes, i.e., transcranial evoked potentials (TEP) (Premoli et al. 2014).
The infinite variability of the stimulation options (pulse waveform, frequency, intensity, number of stimuli, pattern of stimuli, schedule of repeated applications, site of application, type of coils used and its orientation, and any combinations of rTMS applications simultaneously or consecutively) adds to the variability of results across trials. As an example, in neuropathic pain rTMS applications over the primary motor cortex contralateral to the affected body side worked best with high-frequency (10 Hz) but not low-frequency (e.g., 0.5 or 1 Hz) rTMS (Lefaucheur et al. 2001a) and better when intensities used had been below motor threshold.
Further, the selection of physiological brain imaging and/or behavioral outcome measures influence results and type of information that can be deduced from individual studies or meta-analyses.
There is thus a need to describe meticulously and standardize both stimulation and assessment protocols across trials, to document potential modifiers, and to conduct confirmative large multicenter trials with subgroup analyses (only) for approaches with a marked clinical benefit in smaller trials.
12.2.2 Models of Therapeutic Action
12.2.2.1 Motor Rehabilitation After Stroke
Comparing cerebral activation pattern when performing movements with either the paretic or non-paretic hand in patients with unilateral stroke frequently documented a higher bilateral and thus contralesional activity when the paretic hand was moved compared to a more contralateral and lateralized activation pattern with movements of the non-paretic hand (e.g., Grefkes et al. 2008). Two mechanisms have been suggested as explanation for this “overactivity” of the contralesional motor network representing both (a) an adaptive and (b) maladaptive mechanism of functional reorganization. Further, a time-dependent role of the contralesional motor activity has been proposed, with a supportive influence early after stroke that declines with time (Grefkes and Ward 2014). According to a “vicariation model,” (a) homologue sensorimotor areas of the contralesional side can support motor functions that have been lost by damage to the ipsilesional network as an adaptive mechanism of functional reorganization; conversely, in the model of “unbalanced interhemispheric inhibition (IHI),” (b) a net inhibition of the lesioned motor network exerted by the non-lesioned hemisphere acts as a maladaptive influence poststroke and impairs functional recovery. To the extent that such an unbalanced IHI from the contralesional M1 to the ipsilesional M1 exists, both an inhibitory rTMS to the contralesional M1 and an excitatory rTMS to the ipsilesional M1 are treatment options to counterbalance this maladaptive influence (Volz et al. 2015).
While the interhemispheric competition model has explanatory value for rTMS effects that have been observed in motor stroke, it must be kept in mind that the two models that both receive some experimental credit (i.e., the vicariation model and the interhemispheric competition model) would predict opposite effects by rTMS interventions. It remains to be determined for which patient and point in time poststroke the interhemispheric competition model is a valid assumption for rTMS interventions targeting the ipsilesional or contralesional M1.
12.2.2.2 Aphasia After Stroke
Language is represented in distributed brain networks frequently with left hemisphere dominance. Recovery from damage to parts of the network depends on the adaption in the undamaged brain. Functional imaging techniques document activation pattern that is associated with language processing. In recovering from aphasia after stroke, the observed pattern depends on the site and extent of the stroke, and they change over time as does the course of recovery (Heiss et al. 1999): with small lesions outside the primary centers, the original activity pattern is restored and clinically optimal recovery can be observed; with moderate damage to the primary centers, interhemispheric compensation with changes in activation pattern is associated with good recovery; with severe damage to primary centers, reduction of transcallosal inhibition is thought to cause activation of contralateral homotopic areas associated with less efficient recovery of function. Conversely, contralateral homotopic areas might be limiting the functional activity and thereby recovery by their transcallosal inhibition of primary centers.
An intervention that reduces excitability of the contralesional Broca’s homologue area by LF rTMS might facilitate the reactivation of primary centers including Broca’s area and thereby enhance the potential of speech and language therapy (Naeser et al. 2011). This has specifically been shown by Thiel and coauthors (2013): although only one stimulation site was tested in patients with different types of aphasia, the intervention group experienced a more pronounced language improvement than the sham group. The rTMS-induced inhibition of overactivation in homotopic speech areas of the contralesional hemisphere and the shift of activation back to the dominant hemisphere were associated with significant improvement of the language function in the group treated with rTMS combined with speech and language therapy.
Here we have an example where rTMS at one stimulation site (Broca’s homologue) could induce a shift of network activation back from the nondominant to the dominant hemisphere and where this shift was associated with functional/behavioral recovery of a complex function such as language, even though the type of language deficits (aphasia syndromes) and the patients’ lesion sites were different. Larger trials with subgroup analyses would be necessary to learn whether a “one site for all” rTMS target would be a valid model for rTMS interventions in aphasia after stroke. Nevertheless, the experiment shows the potential to intervene and modify recovery of network activities targeting one strategic stimulation site. The coupling of rTMS with speech and language therapy points to a priming role of rTMS in aphasia therapy.
12.2.2.3 Neglect After Stroke
According to Kinsbourne’s “opponent processor model,” each hemisphere causes a natural attention bias to the contralateral hemifield (Kinsbourne 1977). Under normal conditions, the two hemispheres are kept in balance due to interhemispheric inhibition. In spatial neglect patients, damage to either hemisphere leaves the contralesional intact hemisphere unopposed. As a result of this reduced inhibition, the contralesional hemisphere becomes overactivated and causes an ipsilesional attention bias.
When the posterior parietal cortex (PPC) has been used as rTMS stimulation site, both inhibitory rTMS protocols to the left non-lesioned hemisphere (Cazzoli et al. 2012; Kim et al. 2013) and an excitatory rTMS protocol to the right lesioned hemisphere (Kim et al. 2013) produced functional improvements of neglect symptoms with benefits in everyday life situations in patients with right hemisphere stroke suffering from neglect. Here again, there is an example where stroke-related functional deficits could be ameliorated by rTMS. More specifically, assuming that an unbalanced IHI from the contralesional PPC to the ipsilesional PPC exists, both an inhibitory rTMS to the contralesional PPC and an excitatory rTMS to the ipsilesional PPC were treatment options to counterbalance this maladaptive influence in stroke patients with neglect.
12.2.2.4 Dysphagia After Stroke
Dysphagia after stroke is a condition where a bilaterally organized sensorimotor system is affected. Dysphagia can result from a unilateral or bilateral hemispheric stroke or a brainstem stroke. In hemispheric stroke, it seems most severe when the “dominant” swallowing hemisphere is affected (Hamdy et al. 1997), and recovery from dysphagia after hemispheric stroke is associated with an increase of the pharyngeal cortical map in the unaffected hemisphere (Hamdy et al. 1998).
A consequence of this observation for rTMS applications could be to use an rTMS intervention that increases excitability of the pharyngeal motor cortex in the contralesional hemisphere. This would be the opposite to the most frequently used approach in arm motor, aphasia, and neglect rehabilitation after stroke, where excitability-reducing low-frequency rTMS has successfully been applied to the contralesional hemisphere or excitability-increasing high-frequency rTMS to the affected hemisphere’s M1. And yet, HF (5 Hz) rTMS over the contralesional pharyngeal motor cortex for 10 min per day for 2 weeks improved dysphagia in subacute dysphagic stroke patients; the effects were corroborated at a 2-week follow-up (Park et al. 2013). Thus, we have an example where the opposite approach (enhancing excitability in the contralesional motor cortex) to the conventional approach in motor, language, and neglect rehabilitation produced a clear and prolonged clinical benefit.
A parallel observation had been made in gait rehabilitation after stroke. In a sham-controlled RCT with crossover design, positive effects of high-frequency rTMS delivered with a H-coil to both leg motor cortices on lower limb motor function had been documented in chronic ambulatory middle cerebral artery (MCA) stroke patients (Chieffo et al. 2014).
Accordingly, the clinical model for rTMS applications needs to take the basic organization of the treated system into account. It seems unlikely that even for a condition such as stroke, different target symptoms would all be manageable by the same logic. To the contrary, any rTMS approach and the presumed model of action need to be defined and experimentally tested for each condition treated.
12.2.2.5 Parkinson’s Disease (PD)
Motor symptoms are a cardinal feature of PD that to some extent can be positively influenced by rTMS interventions: high-frequency (HF) rTMS over the M1 including less focal stimulation (e.g., leg and bilateral hand M1 rTMS) or over the dorsolateral prefrontal cortex (DLPFC) and low-frequency (LF) rTMS over the supplementary motor area (SMA) have been shown to result in some clinical benefits (see Chap. 9 for details). There were, however, considerable inconsistencies across trials. LF (1 Hz) rTMS of the SMA with a weekly schedule for 8 weeks was among the more favorable rTMS interventions for the treatment of motor symptoms in PD (Shirota et al. 2013).
Thus, the issue of selecting a target site for the treatment of motor symptoms in PD cannot be regarded as solved. It is, however, noteworthy that not only primary motor areas can be rTMS targets in the motor domain but other nodes of the motor network such as the SMA or even areas outside the motor network, e.g., the DLPFC. The mode of action here is not clear. A potential role of an overactive SMA-subthalamic nucleus network in PD had been entertained (Mure et al. 2012). Motor effects following DLPFC stimulation in PD subjects might (in part) be secondary effects due to its antidepressive action.
Given the complex nature of brain networks involved in various functions such as sensorimotor functions, it follows that a variety of target sites can (or must) be entertained for each condition treated. Models of therapeutic rTMS applications don’t have to be restricted to the sites that have been used as targets so far. Rather, the pathophysiology of each condition and the resulting changes in network activities should be taken into account.
12.2.2.6 Neuropathic Pain
Neuropathic pain of either peripheral or central origin has been shown to be reduced after cortical rTMS applications. Most frequently, the primary motor cortex contralateral to the affected limb or side of the face has been treated.
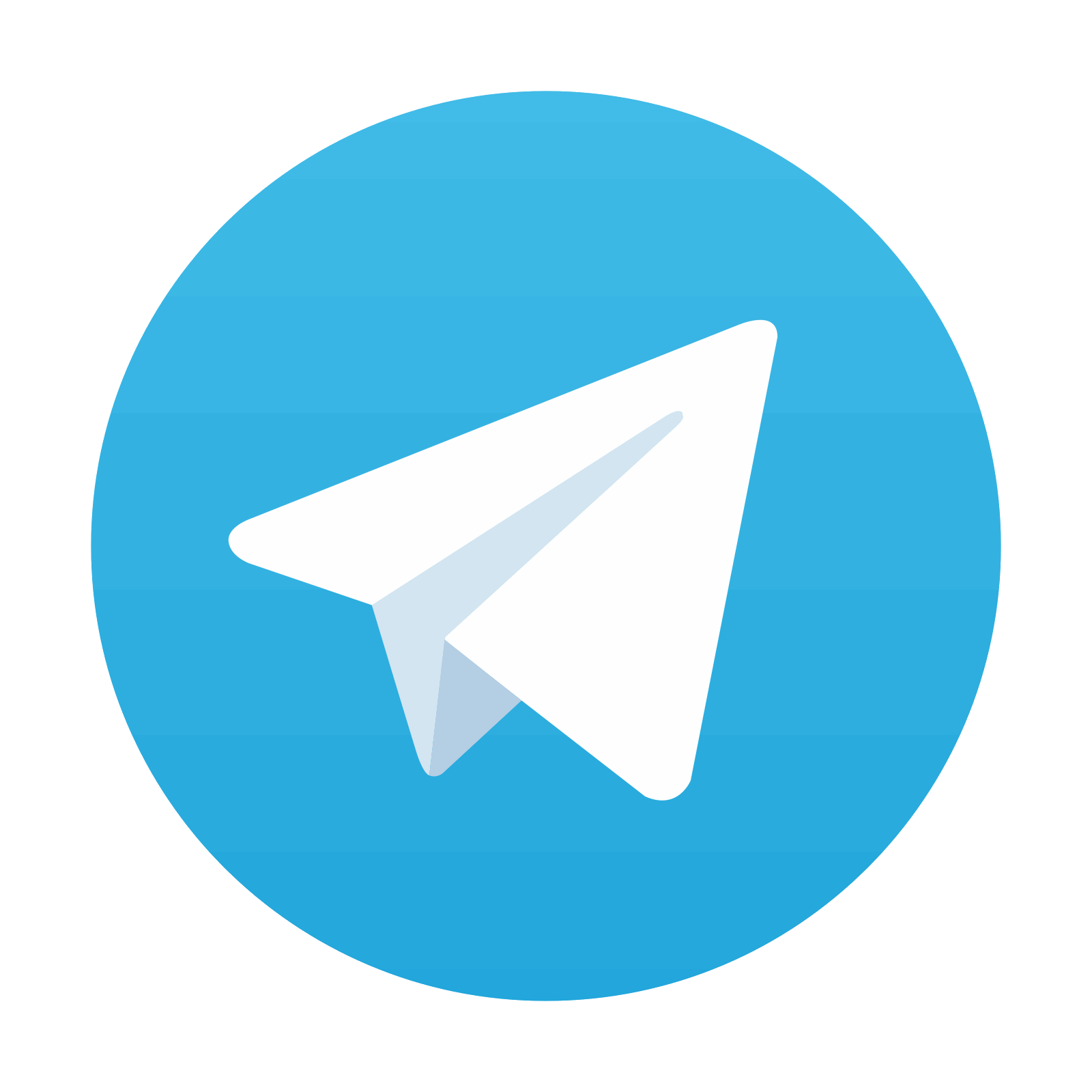
Stay updated, free articles. Join our Telegram channel

Full access? Get Clinical Tree
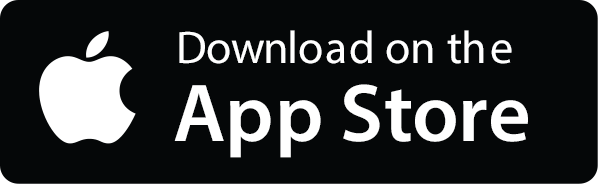
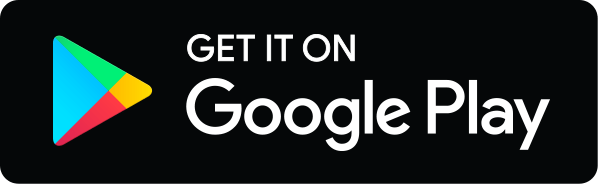
