Fig. 3.1
Multimodal neuroradiological diagnostics in the case of a 56-year-old patient with left-sided progressive visual disorder due to compression of the left optic nerve by a complex ICA aneurysm. Axial contrast-enhanced MRI due to the initial suspicion of a tumourous lesion a. DSA confirming the left-sided ICA aneurysm b. 3D reconstruction of the rotational angiographical data c

Fig. 3.2
MRI 3D MPR data set with contrast enhancement a. Segmentation of blood vessels and simulation of the surgical approach b. Intraoperative navigation guidance with exposure of the aneurysm neck c. The intraoperative image shows the massive compression of the left optic nerve by the medially oriented ACI aneurysm
Modern flow analysis programmes that work with time-of-flight MR data and 2D phase contrast MR date enable a blood flow quantification by using complex calculating algorithms. These quantifications play an increasing role in the planning of intracranial bypasses in revascularising surgery [16, 40, 47].
3.1.3 Computer-Assisted and Navigation-Guided Skull Base Surgery
After the multidisciplinary treatment planning, the actual operative simulation of the therapeutical concept can be done by using a 3D simulation based on CT and MRI imaging as well as on data from angiograms and nuclear medicine. The DICOM compatible data are fused on a workstation enabling a multimodal data set for the simulation including 3D visualisation, image editing, segmentation of crucial structures and designation of anatomical landmarks. This individually edited data set is the basis for intraoperative navigation. Thus, computer-assisted multimodal simulation and surgical planning using navigation data have been established as a basic principle in modern skull base surgery (◘ Fig. 3.3). The use of navigation systems has been accepted as an important strategy in modern skull base surgery [28, 33, 44].


Fig. 3.3
Navigation-guided combined temporobasal anterosigmoidal approach for the resection of petroclival meningioma. Planning of the approach with segmentation of the sigmoid sinus, transverse sinus and basilar artery in relation to the tumour a. Finished approach with dural opening b. With the use of virtual reality, the segmented vascular anatomy can be mirrored into the microscopic view
3.1.4 Radiation Therapy and Stereotactic Radiosurgery
Postoperative adjuvant radiation therapy and specific chemotherapies are becoming more and more important, not only for the treatment of malign skull base tumours. Also the concept of radiosurgical treatment especially in cases of complex skull base meningiomas is of increasing importance. The stereotactical radiation as a primary treatment option or the combination of surgery and radiosurgery with the Gamma Knife or the robot-assisted CyberKnife is an optimisation of the treatment concept (◘ Fig. 3.4). This therapeutical option which has become an established concept is appreciated in this book with a stand-alone chapter [9, 10, 27, 42, 65].


Fig. 3.4
Treatment plan for robot-assisted radiosurgery using the CyberKnife for the therapy of an extensive recurrent meningioma of the sphenoid wing with infiltration of the left sigmoid sinus and Meckel’s cave showing the distribution of isodoses and simulation of multiple radiation angles
3.1.5 Special Techniques and Modern Technologies
Another strategy in skull base surgery is the improvement of surgical techniques as well as the use of specially designed instruments and tools.
A milestone in skull base surgery is the use of navigation as a basic principle for preoperative planning and intraoperative orientation. Furthermore, the refinement of intraoperative electrophysiological monitoring and anaesthesiological procedures contributed to an improvement of postoperative outcome and clinical results. The use of technical innovations, modifications and improvements of anaesthesia, cranial nerve monitoring and blood flow measurement as well as the establishment of ICG angiography as a monitoring procedure in aneurysm surgery and blood flow simulation in bypass surgery are just a few examples that have become strategical basic principles in skull base surgery [18, 23].
Furthermore, the gentle removal of bone tissue adjacent to blood vessels and cranial nerves by using Piezo ultrasound will become a technical standard and surgical strategy in skull base surgery [15].
And also innovations in haemostasis and new dura closure compounds are of importance. The avoidance of CSF fistulas by harvesting autologous tissues like fascia lata will disappear in the medium term since modern and biocompatible dura replacements are available, and, thus, the invasiveness of surgeries will decrease [17].
An important contribution to accurate and patient-individual reconstruction of the scull has been given by modern implant technology [36]. Nowadays very precise computer-aided manufactured implants enable an improvement of cosmetic results as well as an exact reconstruction of the skull base [21, 30, 45]. In the future 3D printing technologies will lead to a faster manufacturing and the use of different materials for the reconstruction of the skull base ([38]; ◘ Figs. 3.5, 3.6, and 3.7).




Fig. 3.5
Simulation and planning of an individualised cranial CAD/CAM titanium implant. The simulation was based on a 1 mm thin-sliced CT data set

Fig. 3.6
Finalised CAD/CAM titanium implant for the reconstruction of the frontal skull and skull base

Fig. 3.7
Intraoperative image after implantation and reconstruction of osteoclastic skull base defect a. Minimal adaption by drilling the edge of the implant with a diamant burr for better fitting accuracy b which is much more difficult than in cases of more recently used materials like PMMA
3.1.6 Era of Miniaturisation
With time a basic change of surgical strategy in terms of surgical radicalness has occurred. Some decades ago the radical resection of the lesion was the main goal of surgery, and, thus, complex and extensive surgical approaches had been developed and brought to perfection. Especially extensive midfacial approaches and surgeries inside of the cavernous sinus resulting from that time are nowadays only used in a few selected cases and have been widely replaced by less invasive techniques. Especially radiosurgery plays an important role in the treatment of complex skull base lesions [14, 26].
During the last few years, there has been a basic strategy in skull base surgery of diminishing radical approaches [46]. Nevertheless, the basic principle of «aggressive to the bone, smooth to the brain» is still valid. The actual common orbitozygomatic approaches or the combined temporal retrosigmoid or anterosigmoid resulted from these principles, and they have several modifications that are further individualised to the patient’s anatomy and the configuration of the skull base lesion by using navigation systems [29, 31, 35].
The new era of minimalisation and miniaturisation of skull base surgery has already begun. Personal clinical experience as well as the literature show that the minimalisation of surgical approaches reduces the trauma to muscles, especially to the temporal muscle and the neck muscles, resulting in faster convalescence and a better subjective well-being after surgery. Furthermore, smaller dural openings and smaller craniotomies are recognised as less surgery-related stress by the patient [25, 41].
Thus, in the future there will be a further individualisation and downsizing of skull base approaches. Modern endoscopy with its significantly improved visualisation will make a substantial contribution to this development [39].
Already today surgical approaches to pituitary adenomas and anterior skull base tumours can be significantly reduced by using endoscopically assisted microsurgery or even pure endoscopic surgery. Beside anterior skull base tumours, this strategy is also increasingly used for lesions of the petrous bone and retrosigmoid approaches. Improved visualisation as well as the training and education of younger colleagues and the expected innovation boost by 3D endoscopy will enhance this trend even further. Neuroendoscopy and the accompanying miniaturisation of surgical approaches will obviously be one of the relevant future strategies in skull base surgery [1, 8].
3.1.7 Training and Further Education
The rapid development of endovascular techniques with an increasing number of coils and stents for the treatment of vascular malformations and stenoses will reduce but not completely replace the number of microsurgical vascular procedures [12, 13, 34, 43]. Thus, an important strategy in skull base surgery is the education and surgical training of younger skull base surgeons who have to be able to treat complex vascular and neoplastic lesions.
In general, highly qualified operative training and continuing education will continue to be a basic principle in this subspecialty. Computer-aided training models on the basis of virtual reality, intense microsurgical training using plastic and animal models especially in vascular microsurgery as well as hands-on workshops with practical microsurgical exercises using cadaveric specimen are methods for training and further education ([7, 11, 22, 37]; ◘ Fig. 3.8).


Fig. 3.8
Practical microsurgical education and «non-bloody» training using models a. Dissection of blood vessels in a cadaveric specimen b. Clipping on a 3D aneurysm model made of plastic c. Checking of the correct clip position and the vascular reconstruction d. Extensive osteoclastic craniotomy and spatula retraction of the Sylvian fissure on a realistic plastic model (Kezlex, Tokyo, Japan) e. Clipping of an artificial 3D aneurysm in the depth of the Sylvian fissure f
3.1.8 Function-Preserving and Function-Improving Skull Base Surgery
The fast ongoing development of the methods and techniques mentioned above will lead to a reduction of surgical morbidity by miniaturisation of surgical approaches which results in the resent development from function-preserving to function-improving skull base surgery.
3.2 Radiosurgical Principles
Radiosurgery is a very effective option for the definitive treatment of primary tumours of the skull base. Radiosurgery can be used as a sole treatment modality for tumours of the skull base in patients who are poor candidates for surgery, for recurrent tumours after primary surgery and as an adjunct to surgery in a combined bimodal treatment concept which is increasingly used to avoid mutilating surgery and provides a high chance for long-term tumour control.
Main indications for radiosurgery are schwannomas of the vestibular nerve and – less frequent – other cranial nerves, meningiomas of the skull base, pituitary gland adenomas and paragangliomas.
Radiosurgery is classically applied as a single-session treatment using dedicated devices like the Gamma Knife, the CyberKnife or the modified linear accelerators (LINACs). There are almost no randomised study data available yet directly comparing the efficacy of the different radiosurgical devices and techniques with each other or with conventionally fractionated treatment.
In current practice small tumours up to 30 mm are often treated with single-fraction stereotactic radiosurgery, whereas the treatment of very large tumours >50 mm is a domain for conventionally fractionated regimen. The Gamma Knife was the first dedicated tool designed for single-fraction radiosurgery. However, since more than a decade, other specialised tools for radiosurgery like the CyberKnife system and specifically adapted LINACs are available. The development of frameless radiosurgery without rigid fixation provides the possibility to apply hypo-fractionated schedules with three or five fractions, which widens the indication for radiosurgery of skull base tumours. These tools opened the possibility also to treat larger tumours of the skull base (>30 mm) and tumours in close proximity to the optic pathway by radiosurgery with ablative doses using hypo-fractionated regimen with three to five fractions.
Editor’s Comment
Uwe Spetzger4
(4)
Neurochirurgische Klinik, Klinikum Karlsruhe, Karlsruhe, Germany
Stereotactic radiosurgery using the Gamma Knife or CyberKnife made the big step from adjunct radiation therapy to a primary treatment option for tumours of the skull base. Especially in the treatment of acoustic neuromas as well as of tumours invading the cavernous sinus, stereotactic radiosurgery plays an increasing role as primary treatment option. This phenomenon is the result of the good treatment results as well as of the acceptance by patients who more and more prefer radiosurgery since they consider an incision and craniotomy as much more invasive than radiosurgery.
Stereotactic radiosurgery in skull base tumours is underrepresented in neurosurgical training and will gain an importance with networking and integration of neurosurgeons in such highly specialised treatment centres. The interdisciplinary and corporate treatment planning as well as the specific consideration and decision algorithm for microsurgery or primary radiosurgery requires adequate expertise and should be made with consensus since this optimises the quality of treatment for the patient. This structure was proven of value during my training and work at the Department of Neurosurgery of RWTH Aachen University with the local Gamma Knife Centre, and I experience this nowadays with the CyberKnife Centre Southwest in Göppingen as an optimum constellation for innovative treatment of complex skull base lesions.
3.2.1 Principles and Methods of Radiosurgery
The Biological Basis of Radiosurgery
In conventional radiotherapy the treated volume is manifold larger than the gross volume of the tumour. Set-up errors, patient movements and tumour movements relative to the patient add spatial uncertainties which demand safety margins of at least 5–10 mm, sometimes more, around the tumour to ensure reliable coverage of the target.
Even in cranial radiotherapy using mask fixation and conformational planning techniques, safety margins of 3–5 mm are not uncommon. Moreover, due to the physics of photon dose distribution and the limited number of beamlets, the lack of conformity to the tumour will additionally increase the volume of healthy tissue within the isodoses that are prescribed to the target.
The need for safety margins and the gradient for dose fall-off beyond the target normally results in a considerable spatial overlap between tumour and dose-limiting normal tissue within the treated volume.
Therefore, to overcome this problem and to widen the therapeutic window, the concept of «conventional fractionation» has introduced into radiation oncology almost 90 years ago.
The term «conventional or normo-fractionation» is related to treatment schedules which distribute the overall dose upon a series of small fractions of 1.8–2 Gy, which are applied five times weekly with a 24-h gap.
The rationale for fractionation relies on biological differences between tumour and dose-limiting normal tissue. In case of cranial irradiation, the dose-limiting normal tissue s (DLNT) include vascular structures, grey and white matter, cranial nerves, inner ear and visual pathway including the eyes. These are slow-growing, so-called «late-reacting,» normal tissue cells with intact DNA repair cope and low DNA turnover rates, which cope much better with sublethal ray damage compared to fast-growing tumour cells with a high DNA turnover rate which harbour often a variety of different DNA repair deficits.
Radiobiology has provided various mathematical models to estimate the fractionation sensitivity of different tissue types. The most commonly used in clinical practice is the so-called α-/β-model . The α-/β-value of a certain tissue type reflects the degree of sensitivity to fractionation. In most cases of cancer treatment, the therapeutic target is characterised by fast-growing tumours with high α-/β-values of 6–10 Gy, whereas the dose prescription to this target is mainly limited by late reaction tissues like nerves with low α-/β-values of 2–3 Gy. In such a setting, the therapeutic index, defined as the ratio between local control (LC) and normal tissue complication probability (NTCP), is increased by normo-fractionation . In these situations, fractionation can be considered as a biological strategy to widen the therapeutic window.
However, the situation may be different for the mostly benign tumour entities of the skull base. For instance, for acoustic neuromas (AN) alpha/beta values between 1.77 and 2.4 Gy and for meningiomas alpha/beta values between 3.3 and 3.6 Gy have been estimated. In such a setting, the benefit of fractionation is questionable since there is hardly no difference between the fractionation sensitivity of the tumour and the limiting normal tissues [121].
Target Volume Concepts in Cranial Radiosurgery
The concept of radiosurgery tries to solve the problem by a «physical» strategy. Radiosurgery aims to separate the target volume spatially from the DLNT. To this end set-up errors must be reduced to submillimetre ranges, so that safety margins can be more or less omitted. This requires a highly reliable and reproducible positioning of the patient, often including rigid fixation and/or the possibility of real-time online correction. Moreover, to avoid an overlap of high-dose regions and DLNT, the treatment plans used for radiosurgery must provide a very steep dose fall-off and a high degree of conformity, even when irregular targets are treated.
These are the prerequisites for the application of high single ablative doses. Keeping these challenges in mind, it is not surprising that radiosurgery was initially restricted to the treatment of intracranial targets with limited volumes (e.g. <30 mm diameter). The reasons are easily understandable: Cranial radiosurgery deals with static targets with a rigid spatial relation to the surrounding bony skull. Therefore, the localisation of the skull, which can be identified very precisely by imaging techniques (X-ray and/or computed tomography (CT), can serve as surrogate for the target. The identification of the target during the planning process relies on additional imaging modalities (MRI, angiography, etc.) which are fused to the planning CT scan.
In the pre-image-guidance era when correction of set-up errors by real-time image guidance was not available submillimetre accuracy could only be achieved by rigid fixation by using an invasive stereotactic frame. Rigid fixation is invasive, time consuming and grossly limited to cranial radiotherapy. These constraints were best resolved by using a single dose, thus defining the term radiosurgery as single-fraction stereotactic radiotherapy. In adequately selected patients, radiosurgery provides a variety of advantages compared to conventionally fractionated regimen: Single-fraction radiosurgery is convenient and cost-effective. In addition, single-fraction radiosurgery (or hypo-fractionated) radiosurgery is highly efficient in terms of local control. At least for tumours with low alpha/beta values, the use of SRS may sometimes enable higher biologically equivalent doses (BED) compared to normo-fractionated regimen. Moreover, high single doses may provide additional biological effects like induction of apoptosis, damage of tumour vasculature, lack of repair and repopulation [121].
The concept of single-fraction radiosurgery was established in the mid-1970s when the Gamma Knife came into clinical practice. More than 10 years later, first attempts have been made to establish this concept also on modified linear accelerators by using rigid frames with invasive fixation. When orthovolt image guidance became available at the end of the 1990s, non-invasive masks were introduced into cranial radiosurgery, but only the combination of real-time image guidance and the possibility of online correction of set-up errors by robotic couches or by robotic radiosurgery systems like the CyberKnife provided a comparable accuracy like invasive fixation. The benefit of these newer system compared to the first to third generation of the Gamma Knife or modified LINACs using invasive frames relies on the flexibility in terms of fractionation. Thus, extremely hypo-fractionated stereotactic radiotherapy/radiosurgery concepts came into practice. With these concepts tumours larger than 30 mm and/or in close proximity to the visual pathway (<3 mm) came into the range of radiosurgery.
Tools for Radiosurgery
Gamma Knife
The Gamma Knife was the first system dedicated to radiosurgery, developed and introduced in the mid-1970s by Lars Leksell. It is the only cobalt-based system still in use for radiosurgery. The initial system underwent various modifications and optimisations. The most up-to-date model is the Leksell Gamma Knife (LGK) Perfexion, which was introduced in 2006; however, the previous model, the LGK 4C, is still widely in use.
The therapeutic gamma ray photons are generated by numerous cobalt sources to produce gamma rays which are collimated and directed to converge precisely at a single point or «isocentre». Accuracy is achieved by eliminating almost any patient movement during treatment by means of a rigid stereotactic head frame. This frame has to be fixed under local anaesthesia. Immediately after fixation computed tomography and/or magnetic resonance imaging is taken for treatment dose planning.
The LGK 4C model includes 201 cobalt sources and collimators arranged in a hemispherical «helmet». Four individual «helmets» with 201 collimators are available to provide beam diameters of 4, 8, 14 or 18 mm each. The LGK 4C has a fixed 40 cm source-to-focal point distance.
The more recent Perfexion model relies on 192 cobalt sources. These cobalt sources are arranged in a cylindrical configuration of five rings. The source-to-focus distance for each ring varies from 37.4 to 43.3 cm. Instead of four different collimator helmets, in the Perfexion model, a single collimator unit is installed which enables the automatic changing of beam sizes between 4, 8 and 16 mm diameters. The advantage of this arrangement lies on the greater flexibility in composite dose distribution shaping, because the beams of different beam diameters can be combined in one treatment session.
In the most recent version, an additional reloca, non-invasive frame is available. In principle, however still time consuming, this device allows also hypo-fractionated therapy on the Gamma Knife. In addition, with this frame also, targeting of more caudal cervical spine locations is feasible. This new frame has a vacuum bite-block, which ensures as demonstrated by a study of the pre-commercial version hypo-fractionation with submillimetre displacement.
CyberKnife
The CyberKnife system (Accuray, Sunnyvale, CA, USA) is a non-invasive system designed for both intracranial and extracranial radiosurgery [48] (◘ Figs. 3.9, 3.10, 3.11, and 3.12). It combines a 6 mV, flattening filter-free LINAC mounted on an industrial robot (Kuka, Augsburg, Germany) with an orthogonal X-ray system (amorphous silicon detectors) which enables real-time image guidance with high frequency and tumour tracking during the whole treatment.





Fig. 3.9
Radiosurgical planning for the treatment of a vestibular schwannoma (©RadioChirurgicum/CyberKnife Südwest)

Fig. 3.10
Radiosurgical planning for the treatment of a meningioma (©RadioChirurgicum/CyberKnife Südwest)

Fig. 3.11
Radiosurgical planning for the treatment of a pituitary adenoma (©RadioChirurgicum/CyberKnife Südwest)

Fig. 3.12
Radiosurgical planning for the treatment of a glomus tumour (©RadioChirurgicum/CyberKnife Südwest)
The kV X-ray imaging system consists of two cameras in the ceiling directed obliquely towards amorphous silicon detectors integrated into the floor. This imaging system is used for 2D-3D image registration of the live images to a digitally reconstructed radiograph which determines the patient position in near real-time and is used to correct patient motions during treatment.
In cranial radiosurgery a so-called 6D skull algorithm is used which is based on bone correlation between the CT planning DRR and the real-time kV images and automatic real-time set-up correcting throughout the whole treatment by the robot.
The treatment table has 5° of freedom for automatic repositioning, and the robot’s arm has 6° of freedom, providing up to 2000 treatment positions with 5–60 mm collimators, a flexible IRUC collimator and – in the most recent version (M6) – a multi-leaf collimator.
A principle design advantage of the CyberKnife system lies in its method of active image guidance during treatment delivery. The system is capable of imaging before every single-treatment beam, although in clinical practice, imaging often can be reduced to intervals of every third to fifth beam or every 15–60 s without losing accuracy. These short imaging intervals in combination with the robot-based online correction of set-up errors achieve a spatial accuracy which is comparable to rigid fixation of the skull. The targeting error of the system lies within a submillimetre range [55].
According to our own experience and the literature, the observed accuracy for intracranial target ranges from 0.2 to 0.3 and even for extracranial target from 0.4.to 0.7 mm [96].
The accuracy of the system is defined by the end-to-end test. This test takes a phantom through the complete treatment planning and delivery process comparing the location of the delivered isodose with the planned dose.
It is often thought that the CyberKnife is a stereotactic system and can only treat small tumours; however, the system is able to treat quite large tumours (up to 2500 cm3) if they are appropriately planned. Since there is no need for rigid fixation in theory, every kind of fractionation regimen is feasible which is an advantage when larger tumours have to be treated. In theory, every fractionation schedule is applicable, even conventionally fractionated concepts. In the clinical practice, however, the system is used mostly for single-fraction radiosurgery and hypo-fractionated concepts with 3–5 fractions. This is recommendable not only due to economic reasons. Also the normal tissue dose constraints for radiosurgery and hypo-fractionated stereotactic radiotherapy are mainly related to these fractionation schedules [178].
Typical treatment times for cranial radiosurgery are about 20–50 min for each fraction. In contrast to conventional radiotherapy, the treatment planning is usually non-isocentric, and the dose prescription does not follow the ICRU guidelines (dose prescription to the 95% isodose). The treatment doses are prescribed usually to an isodose line between 65% and 80% of the maximum dose. This strategy is used because the dose fall-off in the range between the 60% and 75% isodose is often steeper than the range between the 90% and 100% isodose.
But in principle, like the fractionation schedule, also the dose prescription is flexible and should be adapted to the clinical situation. In some cases the CyberKnife is used to emulate brachytherapy dose distribution so the prescription isodose is around 50% like in the Gamma Knife, whereas in other clinical situations, when normal tissue is located within the target as in arteriovenous malformations, prescription isodoses of 80% and higher are used to achieve a higher degree of homogeneity.
Linear Accelerators (LINACs) Modified for SRS
At the time when the Gamma Knife was introduced in the mid-1970s, linear accelerators (LINACs) were used for conventional radiotherapy. In the early 1980s, the first reports had been published about LINACs adapted for SRS purposes. Pioneers in this field were the groups of Oswaldo Betti and Federico Colombo [105, 161]. In 1991 the first commercially developed LINAC dedicated to SRS, the Varian 600 SR system, became available. The initial LINAC-based radiosurgery systems were «retrofitted» conventional LINACs with modifications including circular collimator cones with various diameters, monitoring devices for couch motion and an attachment to connect the couch in a rigid manner with a stereotactic head frame . Planning systems were developed to support treatment planning, which is based on the combination of various noncoplanar arcs.
Due to the rigid fixation of the bony skull throughout the treatment, these systems did not depend on image guidance and the possibility of online corrections of patient movements during treatment. Spherical targets within the brain could be treated with such devices with high precision and satisfactory coverage and conformity, but the systems were limited when irregular targets should be covered adequately. They were inconvenient and time consuming and not suitable for multisession or extracranial radiosurgery.
With the development of image guidance based on kV or mV cone-beam CT or additionally installed X-ray systems in combination with high-definition micro-MLCs and forward or inverse treatment planning systems allowing the planning of intensity-modulated radiotherapy (IMRT), the practice of LINAC-based SRS changed.
Whereas the first-generation LINACs for SRS often used a set of fixed collimators, later generations are more flexible due to the addition of multi-leaf collimators which allow targeting of larger and more complexly shaped lesions. Intensity-modulated radiotherapy was developed in 1992. Since then the intensity of the radiation beam was also able to be dynamically changed with the multi-leaf collimators. Therefore, not only the shape but the dose distribution could be better tailored to irregular targets.
The development of image-guided radiation therapy (IGRT) added the possibility of correction of set-up errors immediately before or even during treatment. Imaging can be obtained by many methods, including radiography, fluoroscopy or cone-beam tomography. These images are then correlated with the treatment planning images to correct changes in target location due to patient motion or natural movement of the target.
With the introduction of image guidance, invasive frame-based fixation was mostly substituted by thermoplastic relocatable masks. The planning as well as the treatment process became much faster and much more convenient for both, staff and patient.
However, since thermoplastic masks allow some motion relative to the system and many devices for image guidance are not able to provide a high-frequency real-time online control throughout the whole treatment, the price for this gain could be a loss of a little bit of accuracy. Thus, many LINAC-based systems add 1 up to 2 mm safety margins to the clinical target volume to compensate inaccuracy.
To ensure precise positioning comparable to invasive systems, more sophisticated masks with bite-block systems have been developed to overcome this problem, but they are not widely used for LINAC-based SRS.
Currently various commercial systems, both dedicated devices for SRS use, and devices that can deliver both conventional radiotherapy and SRS, are available (◘ Tables 3.1 and 3.2). Whereas the Gamma Knife depends on rays generated by cobalt decay (1.17 and 1.33 mV), the X-rays produced by LINACs are over a range of energies which are usually higher (4–6 mV).
Table 3.1
A comparison of conceptual differences between radiosurgery and conventionally fractionated radiotherapy
Radiosurgery (single session) | Conventional fractionated RT |
---|---|
1-day outpatient procedure | 25–28 fractions, 5 times weekly |
Requirement of rigid frame or dedicated online imaging and correction | Non-invasive relocatable frame |
No additional margins to correct for set-up errors | 1–3 mm margins to correct for set-up errors |
Limited to smaller tumours (e.g. <30 mm) | Ability to treat larger tumours with signs of compression |
No improvement of therapeutic ratio with fractionation in slow-growing tumours with low alpha/beta values | Improvement of therapeutic ratio with fractionation in fast-growing tumours with high alpha/beta values |
Growth rate/repopulation does not matter | Growth rate/repopulation matters |
Lack of reoxygenation in apparently hypoxic (malignant) tumours | Reoxygenation |
Lethal DNA damage; additional death pathways (e.g. apoptosis) triggered by high single doses | Additive sublethal DNA damage |
Damage of vasculature | Apparently no effects on tumour vasculature |
Table 3.2
Characteristics of various SRS systems
System | Source or radiation | Patient immobilisation | Extracranial radiosurgery | Fractionation | Collimators | Image guidance |
---|---|---|---|---|---|---|
Gamma Knife 4C | 1.25 mV Co60 (N = 201) | Invasive frame | No | No | 4,8,14,18 mm | No |
Gamma Knife Perfexion | 1.25 mV Co60 (N = 192) | Invasive frame or relocatable non-invasive frame | Caudal cervical spine only | Possible | 4,8,16 mm | No |
CyberKnife | 6 mV LINAC | Frameless | Yes | Yes | 5–60 mm | Orthogonal kV X-ray |
Novalis | 6 mV LINAC | Frameless | Yes | Yes | 3 mm MLC | Orthogonal kV X-ray + infrared cameras |
Novalis TX | 6/15 mV LINAC | Frameless | Yes | Yes | 2.5 mm MLC | Orthogonal kV X-ray kV CBCT |
Synergy S | 6 mV LINAC | Frameless | Yes | Yes | 4 mm MLC | kV X-ray + kV CBCT |
Trilogy | 6 mV LINAC | Frameless | Yes | Yes | 5 mm MLC | kV X-ray + kV CBCT |
Artiste | 6/18 mV LINAC | Frameless | Yes | Yes | 5 mm MLC | kV + mV CBCT |
TomoTherapy | 6 mV LINAC | Frameless | Yes | Yes | 6.25 mm MLC | mV CT |
In general, the LINAC gantry rotates around the patient on a fixed arch. When noncoplanar beam ankles shall be added, the patient couch has to be rotated in the horizontal plane. The intersecting noncoplanar arches deliver a high dose to an isocentric target similar in concept to the intersecting beams produced by the Gamma Knife, but the number of beams is usually lower when static beams are used.
One of the first commercial LINAC-SRS systems was the Novalis (BrainLab, Heimstetten, Germany). BrainLab added an automated tertiary micro-multi-leaf collimator with leaf widths of 3, 4.5 and 5.5 mm for the Varian 600SR and renamed it the Novalis shaped beam radiosurgery unit. The Novalis system is based on a 6 mV LINAC. Image guidance is performed with two infrared cameras and kilovoltage X-rays.
Newer LINAC-based SRS systems include CT-IGRT like Varian Novalis TX (Varian, BrainLab, Palo Alto, CA, USA), Trilogy (Varian), Siemens Artiste (Siemens OCS, Concord, CA, USA) and TomoTherapy Hi-ART (TomoTherapy, Inc., Madison, WI, USA).
The Elekta Synergy (Elekta, Stockholm, Sweden) system was the first one to incorporate cone-beam CT for image guidance for SRS. It uses a kilovoltage cone-beam CT (CBCT) imaging system perpendicular to the treatment beam and a flat-panel detector. The Synergy also has a 4 mm micro-multi-collimator. Its imaging is capable of radiography and fluoroscopy in addition to CBCT. The advantage of CBCT is that a 3D volumetric imaging can be acquired with a single rotation of the gantry.
The Novalis TX differs from other devices due to its high-definition multi-leaf collimator with the smallest leafs with 2.5 mm width. Moreover, it also can deliver radiation in three energy modes: 6 mV photon energy, 15 mV photon energy and 6 mV photon energy with a 1000 MU/min dose rate.
The Novalis TX and the Varian Trilogy have hybrid systems with both kV orthogonal X-ray imaging and CBCT. The TomoTherapy Hi-ART has high-energy mV single-slice CT image guidance. The Siemens Artiste has both kV and mV imaging capabilities. mV imaging has some advantages when high-density surgical hardware has been implanted, but usually this is relevant in some cases of body SRS but not for cranial SRS.
Technical Issues of Radiosurgery for Skull Base Tumours: Treatment Planning, Imaging and Definition of the Target Volume
In the pre-MRI era, target volume definition relied solely on contrast-enhanced high-resolution CT scans of the skull base. Since MRT has been available, the delineation of the target and the organs at risk (OAR) should be based on high-resolution 3D MRI scans that are fused to a planning CT.
All patients are initially fitted with a fixation system, either a rigid frame or a relocatable mask to ensure consistent correct positioning throughout the whole radiological imaging, planning and treatment process. Patients then undergo contrast-enhanced axial computerised tomography.
Prior to delineation of the target and the organs at risk additional imaging is fused with the planning CT. The target volume definition should be based on a high-resolution MRI 3D data set with 0.75–1.5 mm slice thickness including post-contrast T1, T2 and T2-CISS sequences. Brainstem, ipsilateral trigeminal and facial nerve, cochlea and vestibular organ, brain, optic pathway, eyes including the eye lens and pituitary gland should be delineated as organs of risk.
Target planning is usually accomplished by combining pretreatment MR and CT imaging. In tumours with involvement of osseous structures as it is the case in some meningioma, it may be helpful to adjust the treatment planning CT to visualise the bone. In vestibular schwannomas the internal auditory canal can be used as landmark for an exact definition of the intracanalicular part of the tumour. Therefore, also in this case, planning CT should be adjusted to visualise the bone. In addition, the cochlea is better defined when the CT is set for bone visualisation and to be used as a landmark for the quality of image fusion.
Neuromas and meningiomas are best seen on MRI with contrast. But for selected basal tumours, fat suppression imaging may be helpful. For radiosurgery of meningiomas, the limitation of the treated volume is crucial. Therefore, in contrast to fractionated radiotherapy usually only small parts of the dural tail in close proximity of the tumour are included into the GTV. Interestingly, recurrences in the penumbra of the «dural tail» are uncommon [58]. This may be explained by the fact that biopsies of dural tail tissue at resection often show only hyperemic dura and not tumour.
A gross tumour volume (GTV) is defined by integration of all imaging modalities. Due to the set-up error of the different devices for SRS, an additional margin of 0–2 mm has to be added to create the planning target volume (PTV).
Brainstem, ipsilateral trigeminal and facial nerve, cochlea and vestibular organ, brain, optic pathway, eyes including the eye lens and pituitary gland should be delineated as organs of risk.
A maximum tolerable dose is assigned to the principal critical structures based on the volume of the tumour and history of previous radiation treatment. The primary goal of planning for acoustic neuroma radiosurgery is to deliver a sufficient radiosurgical dose to the target volume that conforms closely to the surface of the tumour and spares thereby the adjacent neural structures.
During the individual planning process trade-offs have to be considered between the various organs at risk, e.g. the cochlea, the brainstem and the facial and trigeminal nerve. The evaluation of the plan is based on an analysis of the volumetric dose, including dose-volume histogram analyses of the target volume and critical normal structures.
3.2.2 Vestibular Schwannoma and Schwannomas of Other Cranial Nerves
Introduction
Vestibular schwannomas , also termed acoustic neuromas , are benign tumours of Schwann cell origin. They count for 5–10% of all intracranial tumours; however, they represent 80–90% of tumours of the cerebellopontine angle. Mostly they occur unilateral. Bilateral tumours are hallmarks of neurofibromatosis type 2 (NF-2). The estimated incidence of vestibular schwannomas is 2 per 100,000 person-years, and the prevalence is currently approximately 2 in 10,000 people [145].
Some authors report about an increasing incidence of diagnosed vestibular schwannomas over recent years, but it is unclear whether this simply reflects the increasingly used modern sensitive MRI imaging techniques for patients with respective symptoms and due to other reasons.
The overall prognosis of the disease is good in terms of survival. With the availability of modern techniques for early diagnosis, monitoring and treatment, vestibular schwannomas are usually not live limiting; however, they may have a significant impact on quality of life (QoL) due to hearing loss, tinnitus and dizziness.
The primary goal of treatment is local tumour control; however, secondary goals like the preservation of hearing, release of disease-related symptoms, QoL after treatment and treatment-related adverse effect are additional parameters which must be taken into account for treatment decision making.
Currently there is no international consensus and no high-level evidence in the literature about the appropriate treatment strategy. Management options include observation, microsurgical resection and stereotactic radiosurgery. Moreover, further reports in the literature describe the use of hypo-fractionated radiosurgery or conventionally fractionated conformal radiotherapy. The data about fractionated radiotherapy are sparse, and the published trials altogether encompass a very limited number of patients compared to the number of patients treated with microsurgical resection or single-fraction radiosurgery worldwide [108, 128]. Any randomised data comparing the different strategies to each other are still lacking [145].
Since many of these tumours grow slowly over years or may not grow at all for a period of time, not only the choice of the treatment strategy but also the optimal time point for treatment is a matter of debate. In elderly patients with asymptomatic or minimal symptoms and in selected patients with bilateral NF-2 tumours, observation may be an appropriate strategy, but in most cases, early therapeutic intervention appears to be the best strategy to preserve functional hearing [51].
Surgery appears to be the treatment of choice for very large tumours (>30 mm) with brainstem compression and/or obstructive hydrocephalus. For small to intermediate tumours, stereotactic radiosurgery provides similar tumour control rates of more than 90% compared to microsurgical resection [144]. There may be a trend for better preservation of hearing in patients treated with radiosurgery, but this statement should be made with caution since the hearing preservation rates differ between different surgical approaches, and currently we have no data available which addressed this issue in a randomised trial.
Results of Radiosurgery of Vestibular Schwannoma: Local Control, Functional Outcome and Adverse Effects
The use of stereotactic single-session radiosurgery for vestibular schwannomas was first described by Lars Leksell [125]. Since then this technique with some modifications has been increasingly used as an alternative to microsurgical resection in patients with small and moderate size tumours [94, 114, 144]. Currently there is a common consensus that tumours up to 3 cm in diameter when including the internal auditory canal in the measurement can be successfully controlled in the majority of patients with single-fraction RS. The largest body of evidence for single-session RS comes from Gamma Knife radiosurgery. Currently more than 80,000 patients have been treated worldwide since the introduction of the technique in the 1970s. When the technique was established at the Karolinska Institute, few initial patients were treated with very high doses of 25–35 Gy. Since short-term local control was good but posttreatment hearing loss was reported in the majority of these patients, the prescribed doses were stepwise reduced. Early Gamma Knife and LINAC trials including patients treated in the 1980s used doses of about 14–18 Gy which are still higher than those used in current concepts (see ◘ Table 3.3). This must be taken into account when clinical results are analysed and compared to each other. For instance, Kondziolka and associates treated 162 consecutive patients in the years between 1987 and 1992 with a mean transverse tumour diameter of 2.2 cm and an average dose to the tumour margins of 16 Gy.
Table 3.3
Early SRS trial for VS using higher single dosesa
Author | Median FU (months) | Patients (N) | Marginal dose (Gy) | Prescription isodose (%) | Local control (%) | CN V preservation (%) | CN VI preservation (%) | Useful hearing preservation (%) |
---|---|---|---|---|---|---|---|---|
Leksell 1971 [125] | 44.4 | 160 | 18–25 | NA | 81 | 82 | 86 | 20 |
Flickinger 1993 [79] | 24 | 134 | 17 (median) | 50 | 89.2 | 67.1 | 71 | 35 |
Foote 1995 [80] | 16 | 36 | 16–20 | 50 | 100 | 41.4 | 33.5 | 41 |
Mendenhall 1994 [134] | >12 | 56 | 10–22.5 | 80 | 95 | >78 | >78 | NA |
Kondzioloka 1998 [118] | >60 | 162 | 16.6 (median) | 50 | 98 | 73 | 73 | 51 |
Suh 2000 [177] | 49 | 29 | 8–24; 16 (median) | 80 | 94 | 85 | 68 | 26 |
The follow-up included patients between 5 and 10 years after radiosurgery. Tumour control defined as failure of progression requiring surgery was excellent at 98%. However, the functional outcome was still not satisfying. They reported about comparably high rates of post radiosurgery cranial neuropathies involving the facial of the trigeminal nerve of more than 20% and a drop of 67% in their Gardner-Robertson hearing level [118].
In the 1990s radiosurgery came down to single doses of 12–13 Gy, usually prescribed to the 50% isodose levels in case of Gamma Knife treatment or to the 80 up to 90% isodose in cases of LINAC-based radiosurgery. Imaging and planning quality was also improved, and the planning for schwannomas became more and more conformal. The results published by the University of Pittsburgh may serve as an example for what can be achieved by «up-to-date» radiosurgery of vestibular schwannomas: About 313 patients were treated in Pittsburgh with radiosurgery single doses of 12–13 Gy in the years between 1991 and 2001. The actuarial clinical tumour control rate of these patients was 98.6% after 7 years with unchanged rates of facial strength, facial sensation, stable hearing levels and useful hearing preservation rates after 7 years of 100%, 95,6%, 70.3% and 78,6%, respectively. These excellent results were reproduced by other groups like [99, 100, 142]. In the year 2011, Murphy et al. [143] reviewed present studies of SRS for VS; both concepts form the «high-dose» and the «low-dose» era [144]. They included 13 trials (N = 10 trials with 1324 patients based on the Gamma Knife; N = 3 trails with 340 patients based on dedicated LINAC systems) with modern dose concepts. They found progression-free survival rates of 91% up to 100% when doses of 12–13 Gy are prescribed to the PTV (50–80% ID) (see ◘ Table 3.4).
Table 3.4
Long-term results of SRS trials for VS using lower single dosesa
Author | Median FU (years) | Patients (N) | Marginal dose (Gy) (median) | Local control (%) | CN V preservation (%) | CN VI preservation (%) | Useful hearing preservation (%) |
---|---|---|---|---|---|---|---|
Prasad 2000 [160] | 4.27 | 153 | 13 | 92 | 98.3 | 98.4 | 58 |
Unger 2002 [180] | 6.3 | 100 | 13 | 96 | 100 | 98 | 55 |
Chopra 2007 [67] | 5.7 | 216 | 13 | 98.3 | 94.9 | 100 | 44 |
Hasagawa 2005 [93] | >5 | 317 | 13.2 | 93 | 96/98 (>/<13Gy) | 94/99 (>/<13Gy) | 13/68 (>/<13Gy) |
Myrseth 2005 [146] | >3–4 | 103 | 12.2 | 93 | NA | 94.8 | 32 |
Kim 2007 [115] | 6 | 59 | 12 | 97 | 100 | 98.3 | 33.3 |
Iwai 2008 [99] | 7.4 | 25 | 12 | 100 | 100 | 100 | 64 |
Niranjan 2008 [149] | 3.5 | 96 | 13 | 99 | 100 | 100 | 64.5 |
Fukuoka 2009 [83] | >5 | 152 | 12 | 94 | 97.4 | 100 | 71 |
Murphy 2010 [143] | 3.6 | 103 | 13 | 91.1 | 99 | 95 | NA |
Coombs 2006 [69] | 9 | 26 | 13 | 91.1 | 92 | 95 | 55 |
Friedman 2006 [81] | 3.3 | 296 | 12.5 | 99 | 99.3 | 99.3 | NA |
Kalogeridi 2009 [111] | 4.6 | 19 | 11–12 | 100 | 100 | 100 | NA |
The preservation of functional hearing was in the range of 44–71% when doses ≤13 Gy are used. Not only functional hearing was much better than in the older trials but also the neuropathy rates came down to below 5%. Facial nerve preservation was in a range of 95% up to 100%, and also much lower rates of trigeminal neuropathy of between 0% and 8% were observed in these trials [92, 114, 144]. These favourable functional results were confirmed by two large meta-analyses: A review of 23 articles representing 2204 patients revealed an overall facial nerve preservation rate of 96.2% after Gamma Knife radiosurgery [189], and a review of 45 articles representing 4234 patients showed an overall hearing preservation rate of 51% [190].
After the introduction of the CyberKnife ® by John Adler in 1997, a further device dedicated for radiosurgery was available which provides comparable accuracy and quality of treatment plan for brain tumours compared to the Gamma Knife [187].
Since then the CyberKnife is increasingly used for single-session radiosurgery of vestibular schwannoma [74], the dose concepts were comparable to Gamma Knife treatment; however, CyberKnife normally uses prescription isodoses between 65% and 75%. The group of Wowra which collected experience with both techniques analysed in detail a cohort of 386 patients treated between 1995 and 2008, 257 of them with the Gamma Knife and 159 with the CyberKnife. The patient characteristics as well as the treatment parameters showed no significant difference between both groups with the exception of the prescription isodoses that were 53.5 (52.7–54.3) Gy for the GK patients and 68.2 (67.5–68.9) CyberKnife. They achieved comparable good results in terms of local control and functional outcome which were in the line of the Pittsburgh data described above.
Tsai et al. [179] also reported excellent tumour control rates of 99.1% with a mean imaging follow-up of 61.1 months using the CyberKnife technique. In their cohort 81.5% of the patients’ initial hearing according to the Gardener-Robertson scale I–II maintained GR I or II hearing after CK, with a mean audiometric follow-up of 64.5 months.
In contrast to the first-generation Gamma Knifes, CyberKnife radiosurgery is frameless and does not need rigid fixation. Therefore, hypo-fractionated regimens are also feasible. This has been evaluated by various groups either to treat larger tumours greater than 25 or 30 mm of maximum diameter or to improve the rates of serviceable hearing.
Three to five fraction schedules with doses of 3 × 6–7 Gy or 5 × 4–5 Gy have been evaluated. The Stanford group introduced a three-fraction schedule and reported comparable high rates of local control (98%) as in single-session RS with a very good functional outcome. No trigeminal dysfunction was observed, and only two patients showed a transient facial palsy. Of the 63 patients under evaluation, 74% presented with serviceable hearing (GR class I–II) after a mean follow-up of 48 months [62]. Other groups have also reported about the efficacy and feasibility of hypo-fractionated regimen. With regard to hearing, the results are inconsistent. The rate of hearing preservation was in the range of other published data, but currently there is no clear indication that hypo-fractionation will improve the functional outcome compared to modern single-session concepts [112, 140, 183].
A small series of patients treated with the Gamma Knife either with one session (mean dose 12.6 Gy) or with three sessions (overall dose of 18 Gy) showed no significant differences between both schedules [114]. However especially the Vincenza group demonstrated that hypo-fractionation may be a way to treat patients safely with large VS which are poor candidates for surgery [59].
The aetiology of hearing loss after radiotherapy is a matter of debate. The posttreatment hearing function is a multiparametric issue which is influenced by both treatment- and patient-related factors. Patients with a Gardener-Robertson (GR) hearing class of I or II definitively have a better prognosis than patients with pretreatment GR scales of III or IV. Maybe even more relevant, it has been demonstrated that patients with a pretreatment speech discrimination score (SDS) of >70% were 11.1 times more likely to maintain serviceable hearing following SRS compared to patients with an SDS <70%.
With regard to treatment-related factors, the mean cochlea dose is considered to be the most meaningful parameter. Recently, a meta-analysis of hearing preservation after radiosurgery for vestibular schwannomas has been published in two articles including nearly 6000 patients. A 57% preservation rate of serviceable hearing was reported. Amazingly, patient age and tumour size had no significant effect on hearing preservation, but radiation dose did matter.
In this regard, the question arises in which way mean cochlea dose and the dose prescribed to the target are correlating. It is important to keep in mind that the mean cochlea dose is of course correlated to the dose prescribed to the target, but this relation is strongly influenced by other cofounding factors like the size of the target, its extension with the internal auditory canal and the quantity of the plan, its gradient index in direction to the cochlea and the conformity of the plan. It is not clear yet whether size and location of the tumour or the prescribed overall dose are independent factors which are relevant for hearing preservation or if they are just co-factors which have an impact on the mean cochlear dose. It cannot be ruled out that also the maximum on the cochlear nerve of its DVH will have an addition impact on hearing, an issue, which has been poorly evaluated yet.
Nevertheless, there are various reports which demonstrate that a mean cochlear dose higher than 3.0 up to 4.2 Gy (depending on the threshold for discrimination chosen by the respective study) and a maximum cochlear dose higher than 6.9 Gy were significantly associated with post-SRS hearing worsening. An analysis by Baschnagel et al.[54] reported about a very favourable rate of 2-year serviceable hearing preservation rate of 90% in the subgroup of patients who received a mean cochlea dose of 3 Gy. Therefore, it is our policy is to keep the maximum dose to the cochlea under 7.0 Gy and the mean dose under 3.0 of 4.0 Gy whenever possible.
A matter of concern has been the possible induction of malignancy in vestibular schwannomas after radiosurgery [167]. However, the causal role of radiation is still not quite clear. In fact, few case reports have been published about malignant transformation of VS after radiosurgery. Seferis et al. [167] recently described a new case of verified induction of malignant peripheral nerve sheath tumour (MPNST) in a vestibular schwannoma. This patient is currently the 26th reported case of verified malignant transformation after radiation.
However, most of the cases were patients with NF-2, which has an inherent enhanced risk for malignant transformation to MPNSTs. More important, additional 20 cases of malignant transformation of VS have been reported yet in patients who had not been irradiated before. Seferis et al. [167] concluded that despite recent reports of malignant transformation in vestibular schwannomas after radiation, only in NF-2 cases the incidence of malignancies appears to be notable in relation to the number of patients treated. Even in this group malignant transformation is rare event.
The overall risk appears to be small in the region of 0.01–0.02%. At least in patients which are no candidates for watch and wait strategies, this risk has to be counterbalanced with a reported mortality rate after craniotomies of about 1% [167].
Posttreatment Follow-Up
Clinical Evaluation
During the first 2 years after radiosurgery, ENT and neurological examination and audiograms are performed every 6 months. After the first 2 years, audiograms are obtained annually, after 4 years and depending on the clinic, every 2 years. Speech discrimination, speech reception threshold and pure tone averages should be evaluated from each audiogram. Whenever possible, patients should obtain audiograms at the same facility to minimise errors in technique. Physical examination along these intervals includes neurological examination and testing of the fifth, seventh and eighth cranial nerves.
Radiological Evaluation
The radiological follow-up should follow the same schedule. For the first 2 years after radiosurgery, gadolinium-enhanced MRI scans be should performed every 6 months, in the third and fourth years after initial treatment every 12 months and thereafter in patients without new symptoms every 2 years. Tumour size is measured in three orthogonal dimensions on each follow-up. MRI scans are compared with the previous measurements and the MRI scan used for planning.
Currently there is no consensus about the appropriate length of follow- up. Most of the data regarding local control report about 5- or 10-year local control rates. Some groups advocate a 10-year period of follow-up; some performed after an 8-year follow-up period another MRI 16 years after initial treatment.
Very few reports focus on long follow-up intervals. One series analysed changes in MRI and clinical status in a period of more than 10 years after the initial treatment in 80 patients with unilateral vestibular schwannomas. Tumour progression after 10 years was an infrequent; however, surgical removal was necessary in 3.8% (3/80) at 143, 151 and 167 months. Of notice, no new cranial nerve symptom without tumour growth and no malignant transformation of the tumour were observed more than 10 years after SRS [147].
Conclusions and Implications for Practice
Stereotactic radiotherapy is increasingly used in the management of patients with small to intermediate size vestibular schwannomas . With modern dose concepts and treatment techniques, it is an effective technique providing high rates of local control, comparable to the control rates which can be achieved by microsurgical resection. In addition, the functional outcome is satisfactory with preservation rates of functional hearing of between 50% and 70%, whereas facial nerve palsy and trigeminal dysfunction occur in only 1% up to 5% of the patients.
Since hearing preservation is better with early intervention, watchful waiting strategies should be restricted to elderly patients without or with minimal symptoms.
Until now, there are no randomised studies available which compare various treatment strategies to each other. Therefore, the choice of the treatment modality should be made on an individual basis taking into consideration the patient’s preferences, medical history and individual disease-related parameters.
Surgical resection should be preferred in large tumours >30 m and/or in tumours with acute signs of compression as well as in patients who present with severe dizziness as the leading symptom. Hypo-fractionated radiosurgery of conventionally fractionated radiotherapy may be options for patients with large tumours and contraindication for surgery, but the optimal fractionation regimen still needs to be evaluated.
3.2.3 Skull Base Meningiomas
Introduction
Microsurgery is still the mainstay for the treatment of meningiomas . However, in the recent years, there has been a shift away from aggressive surgical approaches aimed to remove the tumour completely if ever possible to treatment strategies with lower invasiveness providing better functional outcomes. Nowadays there is a major emphasis on the patient’s quality of life and the avoidance of postoperative neurological deficits. Particularly in critical anatomical regions like the skull base, the cavernous sinus and the petroclival region, complete surgical removal of meningiomas is not feasible or can be associated with severe postoperative neurological deficits. Since incompletely resected skull base meningioma carries a significant risk for symptomatic recurrences, primary radiosurgery or combined approaches including tumour debulking and postoperative radiosurgery are increasingly used. Long-term follow-up studies have demonstrated that radiosurgery has an important role in completing microsurgery in these cases by providing postoperative long-term tumour control.
Pretreatment Patient Evaluation
The indication for treatment should be based on up-to date magnetic resonance imaging and a throughout clinical evaluation. CT is helpful in cases of infiltration of osseous structures. The extension of the tumour and its spatial relation to critical skull base structures, namely, the optic pathway, have to be determined in three dimensions. Prior to treatment, clinical evaluation of the cranial nerves and a comprehensive physical and neurological examination have to be done. In meningiomas adjacent to the optic pathway and the pituitary gland, additional pretreatment ophthalmological and endocrinological evaluation of the pituitary axes is mandatory. Trigeminal nerve function is graded on a semi-quantitative scale as normal sensation, decreased sensation or no sensation. Facial nerve function can be graded on the House-Brackmann scale.
Results of Radiosurgery: Local Control, Functional Outcome and Adverse Effects
To date, however, no formal randomised study comparing surgical resection with radiotherapy or radiosurgery with other forms of radiotherapy techniques has been published. Nevertheless, single-session radiosurgery has become a generally accepted technique for managing a small inaccessible benign skull base meningioma (WHO grade I and II) [50, 63, 64, 70, 71, 82, 119, 120].
For radiosurgery local control rates of 92–100% have been reported when single doses between 12 and 16 Gy for grade I meningiomas and between 14 and 20 Gy for grade II prescribed to the 50 or 70% isodose are applied. The overall toxicity and especially the toxicity to the cranial nerves are commonly kept below 5% when modern techniques and dose concepts are used which respect the dose constraints for cranial nerves and brain tissue. Even for meningiomas at the cerebellopontine angle, facial nerve morbidity is an infrequent event. Especially for parasellar meningiomas, the tolerance of the optic pathway is one of the most critical issues. Maximum point doses should be kept below 10 Gy (single fraction) to optic pathway structures in patients without pretreatment. Other cranial nerves obviously tolerate slightly to moderately higher single doses [141].
These satisfactory results reported from various single institutions are in accordance with the largest corpus of date regarding radiosurgery of meningiomas published in 2012. A retrospective multicentre observational analysis included 4565 consecutive patients harbouring 5300 benign meningiomas. All patients were treated with single-fraction radiosurgery with a median prescription dose of 14 Gy. The median tumour volume was 4.8 cm3, and the median imaging follow-up 63 months. After exclusion of the patients with an imaging follow-up of less than 24 months, 3768 remaining meningiomas (71%) were available for evaluation [165].
The overall tumour control rate was 92.5% defined by no change or shrinkage of the tumour. In 58% of all cases, the volume of treated tumour tissue decreased and remained unchanged in 34.5%. The reported 5- and 10-year progression-free survival rates were 95.2% and 88.6%, respectively.
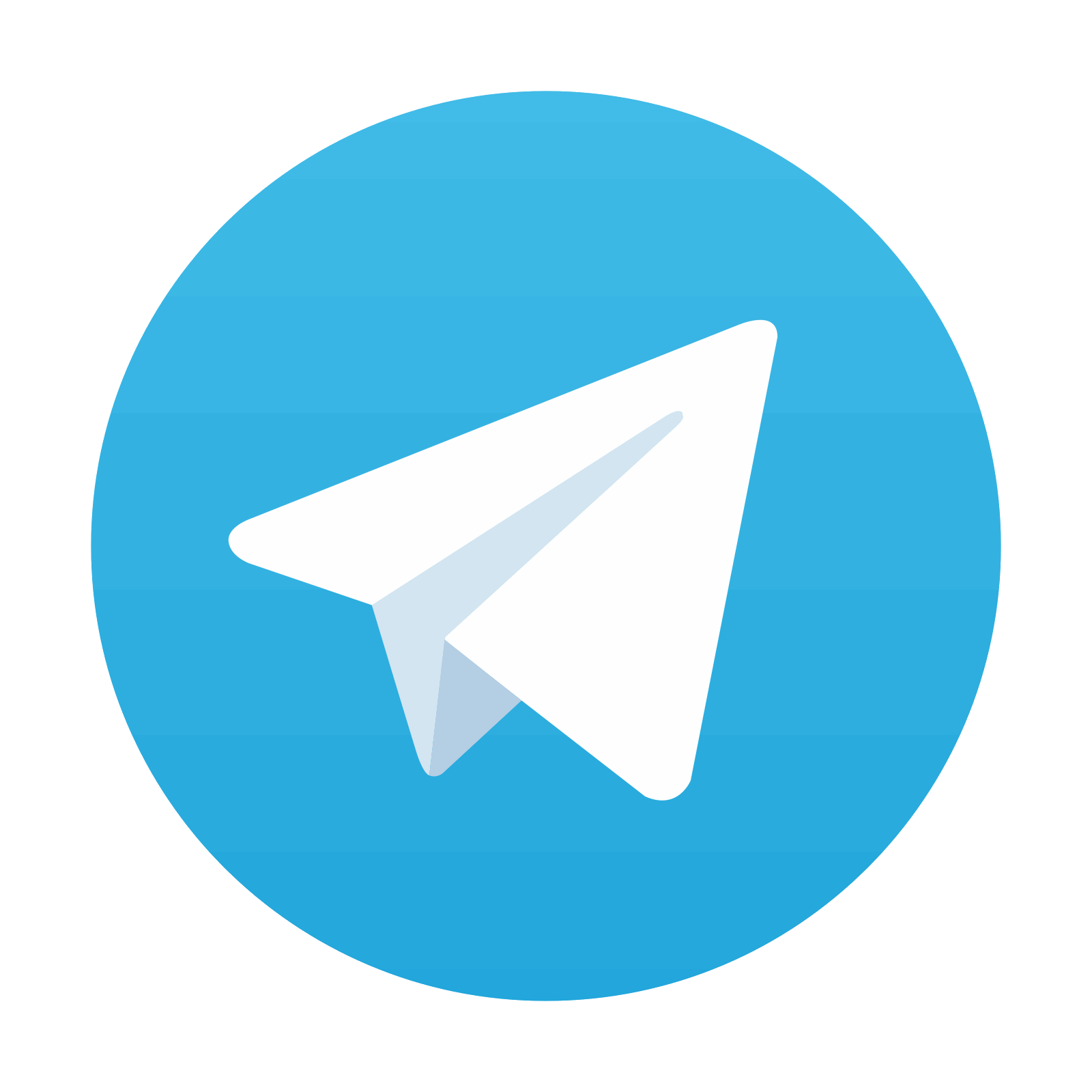
Stay updated, free articles. Join our Telegram channel

Full access? Get Clinical Tree
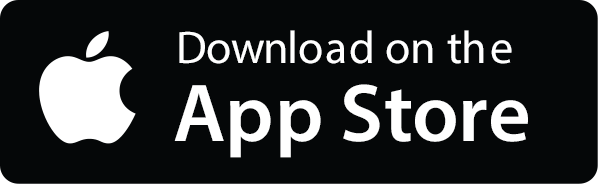
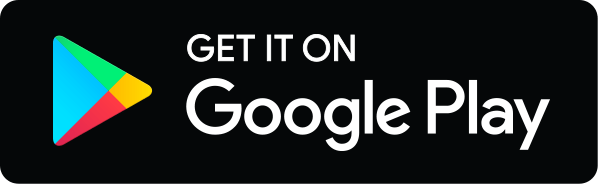