Chapter 83 Thoracic and Lumbar Spondylosis
Anatomy, Pathophysiology, and Biomechanics
The intervertebral disc is bound ventrally by the anterior longitudinal ligament, dorsally by the posterior longitudinal ligament, and rostrally and caudally by cartilaginous end plates that abut the vertebral bodies. The anulus fibrosus forms the outer ring of the disc and provides most of the structural integrity. A softer, notochord-derived nucleus pulposus forms the center portion of the disc and, although not as strong as the anulus, provides cushioning and some resistance, mainly to axial loads. With aging, the disc progressively desiccates and becomes less elastic, a process that has been termed disc degeneration. In the case of spondylosis, disc degeneration contributes to the overall pathology in several different ways. As the disc desiccates, a process that is demonstrable on MRI by loss of T2 signal (so-called dark disc disease) (Fig. 83-1), it has the potential, by virtue of its innervation by the recurrent sinuvertebral nerve, ventral rami, and rami communicantes, to contribute to back pain.1 With the loss of hydration, the disc may become incapable of resisting physiologic biomechanical forces, resulting in failure by herniation through a defect in the anulus. This herniation may result in neurologic deficit and/or pain. Even in the absence of true herniation, a broad-based bulge may compress the neural elements, causing symptoms. Apart from symptomatology, the desiccated disc is no longer able to perform a portion of its biomechanical function in the normal movement of the spine. Other elements of the spine must therefore bear the resultant biomechanical stresses, potentially accelerating their degeneration.
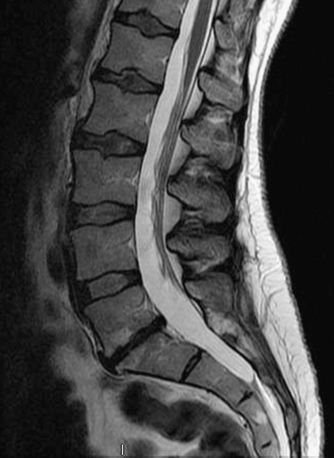
FIGURE 83-1 Sagittal T2-weighted MRI of the lumbar spine illustrating advanced disc degeneration at L5-S1.
Facet joints in the thoracic spine and the lumbar spine are alternatively referred to as zygapophyseal or apophyseal joints. Because they oppose the neural elements, degeneration and hypertrophy may cause compression of spinal roots, the spinal cord, and the thecal sac of the lumbar spine. Each joint is composed of the superior articular process of the caudal vertebra and the inferior articular process of the rostral vertebra. The opposing surfaces are covered with synovium, while the outer surface is covered by fibrous capsule. The joints of the thoracic spine from the C7-T1 joint to the T9-10 joint are typically oriented in the coronal plane and assume a configuration not unlike that of shingles on a roof. The T10-11 joint is often a transition area where the joint orientation becomes slightly tangential to the coronal plane. The portion of the joint that is visible dorsally in the lumbar spine is mostly composed of the inferior articular process of the rostral vertebra; this is readily demonstrated with removal of the joint capsule. The superior articular process of the inferior vertebra forms the ventral and lateral portion of the joint. Because of its location directly adjacent to the exit point of the nerve root laterally and the thecal sac medially, the superior facet process often comprises the point of maximal compression when joint hypertrophy leads to neural compromise. Because innervation of the facets themselves is via medial branches of the dorsal primary rami, degeneration of the facets may produce back pain by this mechanism.2–4
Signs and Symptoms
Back Pain
As previously stated, lumbar and thoracic spondylosis may cause back pain. This multifactorial complaint is very common and may occur in the absence of defined spinal pathology. In degenerative spondylosis, however, the generation of pain may be due to facet hypertrophy, disc degeneration, spinal instability, and/or referred pain from neural compression. These causes are generally difficult to separate from each other; even in the face of overt instability, the so-called pain generator may be protean and difficult to treat.
Nevertheless, the mechanisms by which possible sources of pain in spondylosis affect patients bear discussion. As was mentioned earlier, the intervertebral discs and facet joints are innervated and may cause pain with degeneration.1–4 Furthermore, as the spine ages, the degenerating facets and increasingly desiccated intervertebral discs lose some of their ability to maintain normal motion and support of the vertebral column. As a result, paraspinal muscles may be recruited to maintain posture, and this may contribute to painful paraspinal muscle spasm.
Radiculopathy
The underlying pathophysiology of radiculopathy is most likely multifactorial. Direct mechanical compression of a nerve root certainly plays some role in the generation of radiculopathy, particularly as removal of the offending lesion frequently results in marked improvement in symptoms. In the lumbar spine, this compression most commonly occurs in the lateral recess and affects the nerve root exiting the next most caudal neural foramen (Fig. 83-2). Thus, a paracentral herniation at L4-5 most commonly affects the L5 nerve root exiting the L5-S1 neural foramen. However, while it is intuitive that a herniated thoracic or lumbar disc causes pain by direct mechanical compression or stretch on the nerve root, many patients have disc pathology that abuts or compresses the neural elements without associated radicular symptoms. Disc material is both immunogenic and inflammatory.5,6 Animal models have demonstrated that nucleus pulposus material causes an inflammatory reaction and a demonstrable increase in reactive cytokines. Tumor necrosis factor alpha has been proposed as a possible underlying inflammatory factor.7–10 Both processes have been implicated in symptom generation.
Radiculopathy is not always the result of disc pathology. As the thoracolumbar facet joints hypertrophy as the degenerative process progresses, compression of the ventrally lying nerve roots may occur. This compression generally occurs in the aforementioned region directly adjacent to the exit point of the nerve root laterally and the thecal sac medially, termed the lateral recess (Fig. 83-3). The ligamentum flavum may also hypertrophy, contributing to this compression.
Myelopathy
When spondylotic processes result in compression of the spinal cord, myelopathy may occur. Spondylotic processes in the thoracic spine, including disc pathology, facet hypertrophy, and ligamentum flavum hypertrophy, may cause direct compression of the spinal cord. In the lumbar spine, the situation is somewhat more complex, as the spinal cord in most adults ends in the region of L1-2. Thus, compression from spondylotic pathology at lumbar levels causes symptoms related to compression of the spinal cord, conus medullaris, or nerve roots. Symptoms from pathology at the thoracolumbar junction therefore vary according to the neural structures that are affected, although rarely true thoracic disc herniation can mimic lumbar radiculopathy.11,12
Claudication
When spondylotic processes result in compression of the thecal sac below the conus medullaris, neurogenic claudication may result. Most commonly, this occurs as the result of spondylotic spinal stenosis caused by hypertrophy of the ligamentum flavum, by facet arthropathy and subsequent overgrowth, by broad-based intervertebral disc bulges, or by a combination of any of the three. These symptoms are to be distinguished from vascular claudication.13 Pain often extends down the back of the legs into the calves in neurogenic claudication, while the pain of vascular claudication is often described as being in a “stocking” distribution. With vascular claudication, relief often comes quickly after rest; simply resting does not often help neurogenic claudication. Patients frequently must sit or assume a flexed or stooped posture (the so-called shopping cart sign) to relieve the pain of neurogenic claudication. Vascular problems that cause claudication usually result in diminished or absent peripheral pulses and cool extremities, whereas in neurogenic claudication, the lower-extremity examination may be entirely normal.
Correlative Diagnostics
MRI findings in lumbar spondylosis generally differ from those in thoracic spondylosis. Degeneration, as was mentioned earlier in the chapter, may be associated with disc herniation, ligamentum flavum hypertrophy, and facet joint hypertrophy. The summation of these pathologic tissue responses may cause stenosis of the spinal canal in the midline or lateral recesses of the canal (Figs. 83-4 and 83-5). Facet hypertrophy, ligamentum flavum hypertrophy, or broad-based disc bulges (Fig. 83-6) may also contribute to stenosis of the central canal, lateral recesses, or foramina. Extraforaminal disc herniations may cause compression of the exiting nerve root lateral to the neural foramen (Fig. 83-7). As spondylosis progresses and joint arthropathy worsens, increased fluid within the facet capsule itself or the presence of synovial cysts associated with the joints may contribute to compression of the thecal sac and/or nerve roots (Fig. 83-8). In the absence of frank instability, these findings may also portend the development of instability following surgical decompression without stabilization.
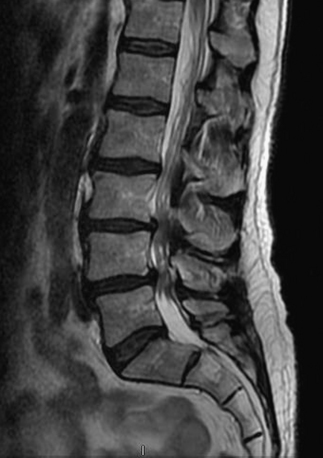
FIGURE 83-4 Sagittal T2-weighted MRI of the lumbar spine illustrating central stenosis at L2-3, L3-4, and L4-5.
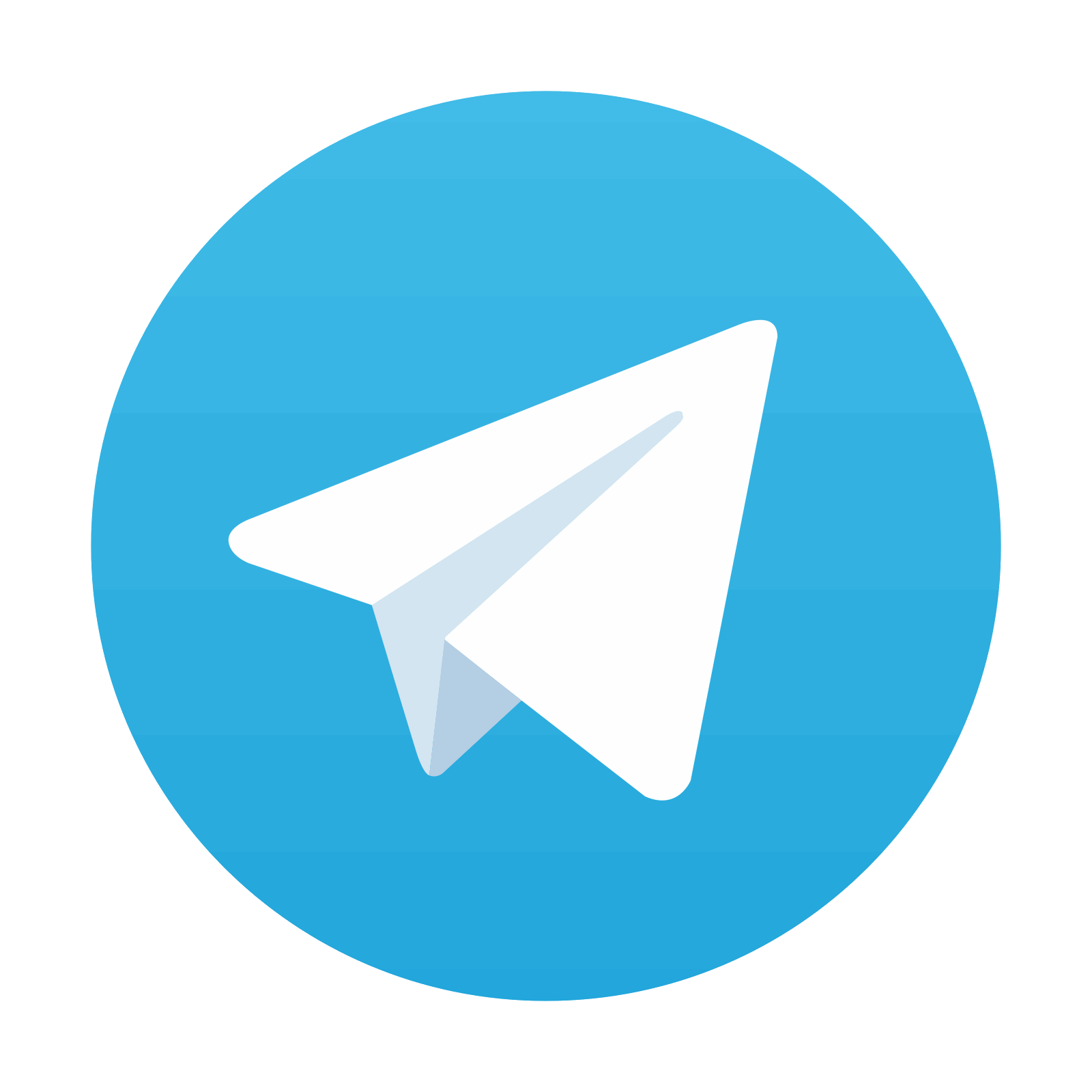
Stay updated, free articles. Join our Telegram channel

Full access? Get Clinical Tree
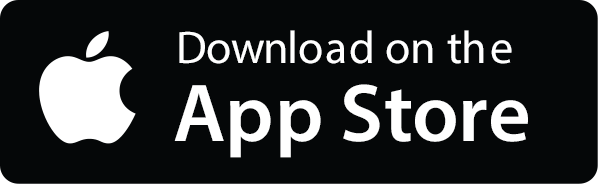
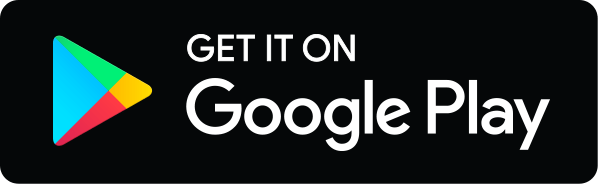