Figure 15.1 Propagation of activation from input to response (thin lines), and task-set related processes modulating bottom-up processing. The box WM shows task-set representation in working memory. The goal representation modulates activation propagation at the task level (semantic layer). The mapping representation modulates activation propagation at the level of the S–R mappings. Furthermore, conflict sensitive controls at the different levels show modulation of propagation at anterior levels.
the automatic processing route, this modulation will not always be powerful enough. Therefore, on some occasions, the response performed may be the one propagated via the automatic route. Sometimes, this will be the correct response, but at other times the response will be incorrect because of stimulus–response compatibility (as observed in the Simon effect, the SNARC effect, etc.) or because the stimulus–response association is a particularly strong one (e.g., a prepotent response). For a completely rational system, this implies that other interactions will be necessary to maximize correct performance.
Several authors have explored the idea that neural structures within the anterior cingulate cortex monitor the presence of response conflict, i.e., a situation in which one response is not favored over another one (see Botvinick et al. 2004). When such a conflict is detected, the attentional selectivity on the input is changed, which in its turn results in an adjustment of the activation of the response alternatives. Although a very interesting idea, conflict detection only at the level of response choice (output level in Figure 15.1) does not seem to be enough when there are several task parameters that may affect the response activations (as also suggested by Botvinick et al. 2004). In the example we have been considering thus far, response conflict is only partially informative, because it is the product of several information processing routes that are at work. However, the idea may be extended by assuming that conflicts may occur at different processing stages and that all these (p.250) conflicts may be monitored. This leads to the propagation of a signal that may be used to modulate processing. In particular, we propose that a conflict may also occur at the semantic level. Basically, at this level, two kinds of conflicts may be distinguished. First, it may be argued that at this level a kind of implicit response is required in the categorization of the inputs. In the example of Figure 15.1, a digit has to be categorized as large or small. If the activations of the two categories conflict (are rather similar), then a conflict arises that may be detected and used for further adaptations. Second, it is also possible that the amount of activation for the relevant task (magnitude judgment in our example) conflicts with activations in nodes associated with other, probably irrelevant, tasks. If the activations accrued for an irrelevant task compete with the activation in the relevant task stream, there is again a conflict that may be detected and counteracted by biasing processing towards the relevant task.
This extension of the conflict-monitoring hypothesis is displayed in Figure 15.1. A conflict monitoring unit is present in this figure not only at the level of response choice, but also at the level of stimulus categorization. In both cases, the output of the conflict monitoring unit feeds a signal to the task-set representation in working memory. More specifically, the conflict detected at the output level is propagated to the stimulus–response mapping configuration, so that via this connection adaptations can be initiated directed to the mapping layer. By biasing the activations towards the relevant stimulus–response mapping, it may be possible to reduce the conflict. Similarly, the conflict detected at the semantic level propagates a signal to the goal representation in the task-set and from there the activations leading to stimulus categorization may be modulated. The figure shows one of the possible streams, namely to bias the activations towards the relevant task.
Occasionally, conflict detection will not suffice to bias the system towards the appropriate response. This may result in the selection of an incorrect response. While this happens, the cycle of conflict monitoring and subsequent adjustments may still be operative, so that it becomes possible to detect that there is a mismatch between the response being executed and the one that should have been selected. This mechanism is then at the basis of the detection of an error. As the cycle is continuing to produce adjustments, this will lead to an increase of the response threshold with a subsequent slower responding on the following trial (e.g., Gratton et al. 1992).
It should be clear from this explanation that response selection is based on an interaction of bottom-up routes (from stimulus to response) and top-down modulations based on the signal provided by conflict detection units and propagated further on by mediation of the currently active task-set. In this proposal, conflict is monitored at two different stages of information processing and the modulations based on these conflicts are also mediated by different components within the task-set representation. At present, the number of stages at which conflict monitoring may be needed is not clear at all. However, we have argued that such monitoring occurs at least at two levels.
The stimulus–response translation processes described here seem to fit well with the traditional distinction between three processing stages, namely perception or stimulus identification (the lowest two levels in Figure 15.1), response selection (semantic to output levels), and response execution. One possible view is that the response selection stage is capacity limited so that only one response selection can be performed at any time. This central bottleneck hypothesis has been proposed to explain the psychological refractory period (PRP), a response delay which occurs when the stimulus for a second task follows very shortly (0–300 ms) after the onset of the stimulus for the first task (e.g., Pashler 1984; Ruthruff et al. 2001). This bottleneck hypothesis has been challenged, however, by the observation of cross-talk between the two tasks in the backward compatibility design (e.g., Hommel 1998). Without abandoning the idea that response selection is capacity-limited, the latter findings can be explained by distinguishing four, instead of three processing stages. After stimulus identification and before response selection, there is a stage of (p.251) response activation which is not capacity-limited and in which the possible response alternatives are activated prior to the selection being made (cf. Lien and Proctor 2002). With reference to Figure 15.1, response activation concerns the bottom-up processing of the incoming stimulus up to the output level. Response selection itself comes into play when the detected conflicts lead to a modulating signal that is propagated via the active task-set in working memory. In the first of these two stages (response activation), the activations of the incoming information are quasi-automatically propagated through the network. In the second stage, the propagation of information is modulated by information propagated from the working memory representation.
A number of neuroimaging studies support such a distinction between response activation and response selection stages of processing. Bunge et al. (2002) report an event-related fMRI study in which healthy adults performed a flanker task. On the basis of appropriate comparisons, the authors could conclude that the parietal cortex is involved in the activation of possible responses and that areas in the prefrontal cortex, in particular, the lateral prefrontal and rostral anterior cingulate cortices, are recruited when a selection between competing responses is needed (see also the review by Bunge 2004).
The mechanisms discussed thus far cover an important part of executive control, namely that part which is involved in the selection of an appropriate response. We have described a system from which goal-directed behavior emerges because there is a task-set representation modulating the activations that are propagated from stimulus to response. The system is also capable of error monitoring, because on error trials, there is a heightened conflict at some processing stages. The monitor being sensitive to such conflict propagates this back to the task-set representation in working memory, which in its turn modulates propagation of the activation from stimulus to response. The (part of the) system as described here explains that response selection, rather than response activation, recruits the relevant task-set representation in the capacity-limited working memory system.
Dual-task coordination and response selection
The question may now be raised how such a system explains the trade-offs observed in dual-task situations typical of working memory research. Actually, two different situations must be distinguished. A first type of dual-task situation concerns the execution of two processing tasks concurrently. The second type concerns combinations of storage and processing as in the complex memory span tasks.
When two tasks must be performed concurrently, the task-sets for both tasks must be configured and maintained in working memory. At each time one of the two task-sets is more activated than the other one. The most active task-set is considered to be dominant in that it currently governs controlled processing and behaviour, because only this task-set can send signals to modulate processing. When the activation for the competing task-set increases so that this one becomes dominant, then only this task-set can affect information processing in agreement with the task goals and task constraints. This constitutes a bottleneck on control. This bottleneck is functional, rather than structural, because the fact that there is a bottleneck depends on the fact that there are two independent tasks. If after extensive practice the two tasks are coordinated into a single task, the processing limitation is no longer effective.
If two tasks are performed concurrently, and both tasks require the selection of an appropriate response, then both tasks will also require controlling signals forwarded from the task-set representation. However, only the signals coming from the dominant task-set will be propagated and have an effect on resolving the conflict at the semantic or the response level. If meanwhile the nodes activated for the second task require also resolution of a conflict, the processing of the conflict (p.252)adaptation will be delayed until the second task-set becomes dominant. As a consequence, responding to the second task will be delayed and in some cases, the delay may cause the conflict to be resolved by bottom-up propagation of activation, occasionally resulting in an error. Hence, the competition between the two tasks will lead to delayed responding and an increase in errors. These delays will not be restricted to one task. Sometimes, the ‘second’ task will be the dominant one, and the first one will have to wait. In sum, on the basis of this view it is expected that when both tasks require the selection of an appropriate response among two or more alternatives, both tasks will be slowed down and will be more error-prone in comparison to the same tasks performed in single-task conditions.
The second kind of dual-task design involves a trade-off between maintenance of information and processing. In order to clarify this trade-off, we shall start from the example of a working memory span task, also known as complex span task. In the operation span task (Turner and Engle 1989), for example, participants have to memorize a sequence of words while performing a series of calculations. A trial starts with a rather complex arithmetical operation that must be solved; after that, a word is presented for later recall. Then another operation is given and another word is presented. After such a sequence of 2–6 operations/words, the participant is required to recall the words in the correct order. Trials start with a sequence length of two and continue as long as the participant is able to respond correctly to the majority of the trials of a given length. A better variant of the task reverses the two components in each trial by first presenting a word to-be-remembered followed by an operation to be performed (see Barrouillet and Camos 2001). This procedure ensures that from the very start of a trial, a memory load is present.
This is clearly a dual-task design; it requires the participant to achieve two task goals. On the one hand, it is necessary to remember all the words in the presentation order. On the other hand, it is necessary to perform well on the calculation task. Hence, the participant must configure two task-sets that are kept active during the entire test procedure. The first task requires maintenance in memory of the sequence of words in the correct order. To achieve this goal, the memory traces must be reactivated before they fade away. Without the presence of a calculation task, memory maintenance can be achieved by subvocal rehearsal so as to keep the phonological word forms active (in the phonological loop). However, it is quite likely that both the passive phonological storage and the articulatory rehearsal loop are also used to solve the rather complex equations (maintenance of subgoals of the calculation, interim results, whether or not a carry is needed, etc.). In other words, to maintain the words in (short-term) memory, attention has to be paid to the words and meanwhile the numbers for the calculation task are presented and now the participants have to execute the appropriate operations to produce the correct answer.
How can the participants succeed in both tasks of such a dual-task set-up? Probably the control mechanisms will be involved in both tasks. These mechanisms are part of different processing routes (word memorization and calculation) and work independently from each other. They propagate signals to the respective task-sets stored in working memory. Our assumption is that only one task-set governs behavior at any one time (cf. supra). Consequently, the signal from the control mechanism from only one task at a time can be handled to modulate processing and this depends on the task-set presently active (or dominant). This way, a functional bottleneck arises at the level of the controlling or adjusting working memory representation. While the signal of one of the tasks is processed, the other task has to wait and as a consequence, processing is delayed. At times the delay may be so large that errors occur. Hence, the dual-task situation leads to performance being slower and/or less accurate. Similarly, when the memorization task is kept on hold, it may become impossible to access the stored element and at the end of the series, recall is incomplete.
To sum up, if our view is correct, response selection plays an important role in dual-task situations as well with a memory and a processing task as with two concurrent stimulus–response tasks. (p.253)The reasons for this are (a) that the task-set components send controlling signals to modulate ongoing processing and (b) that only the most active or dominant task-set can exert this kind of control. The result of this is that in a situation with two stimululs–response (S–R) tasks, both tasks are impaired (slower and possibly less accurate), and that in a situation with competing storage demands and an S–R task, similarly, maintenance of stored information and performance on the S–R task will suffer.
Control at the level of response selection
Since the view elaborated here strongly depends on the presence of response selection in the S–R tasks, the question arises as to what happens if only one of both tasks involves response selection. It is known that a simple reaction-time task does not involve a response selection (Schubert 1999). Although such a task may still need some form of control for its proper execution, no choice requirements must be met. No semantic categorization of the stimulus is required, as it suffices to detect the presence of a stimulus to emit the response. Therefore no conflict is expected to occur at either the semantic level or the output level, and there will be no requirement to modulate processing so as to resolve these conflicts. These expectations are supported by an event-related potentials (ERP) study of Szmalec et al. (submitted). These authors found a large N2 component, which is considered to be a marker of cognitive conflict, for a choice reaction time (RT) task but no N2 for a simple RT task.
Also at the behavioral level, the role of response selection can be estimated by comparing the effects of a choice RT task to the effect of a simple RT task. This yields a simple and straightforward methodology to test the prediction that the presence of response selection in a dual-task setting where the other task involves either memory maintenance or another choice task will result in impaired task performance. This implication concerning response selection was tested in a series of behavioral studies. In the remainder of the current section we present a brief overview of these studies and the obtained results, in saccade control, in verbal fluency, in mental arithmetic and in working memory tasks involving the slave systems.
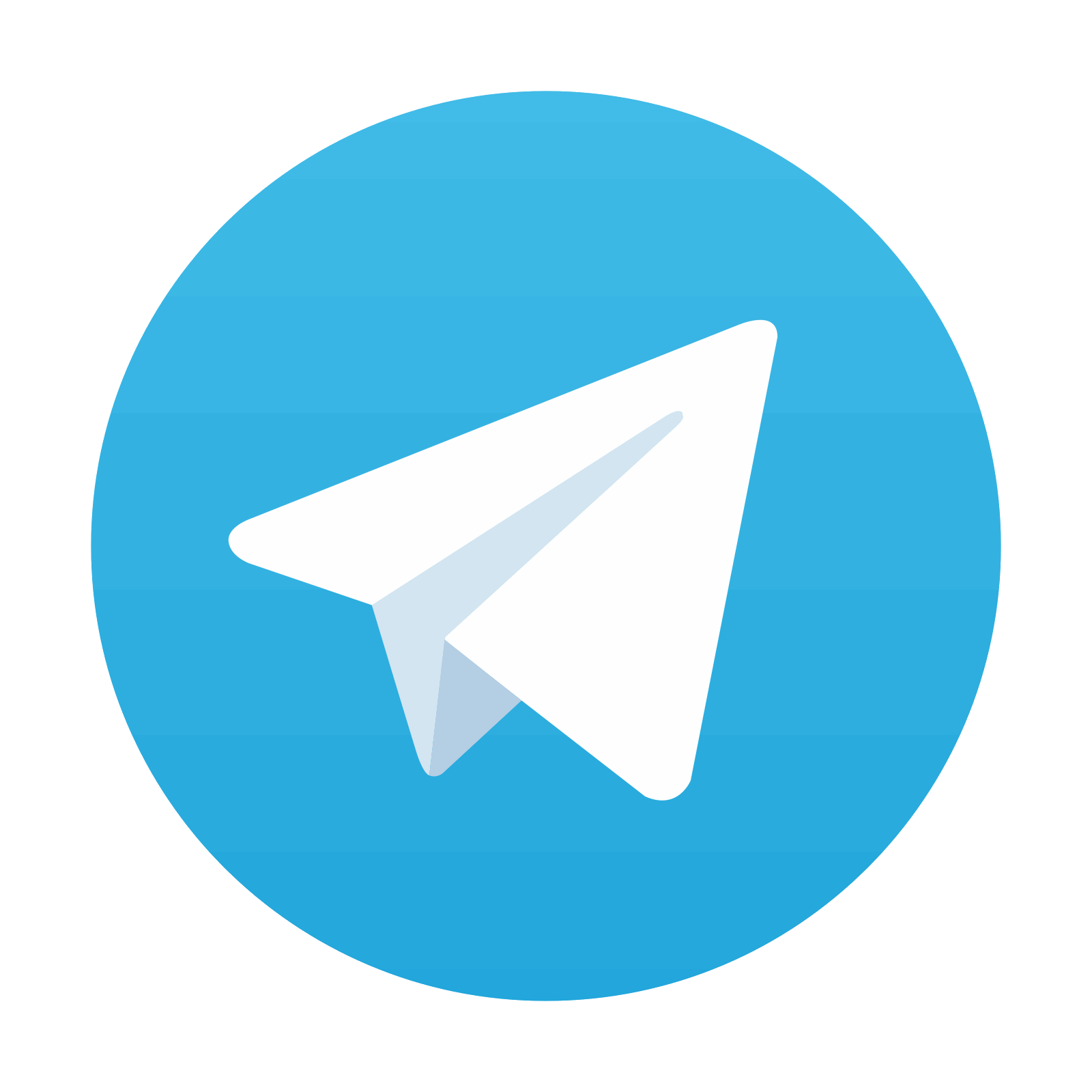
Stay updated, free articles. Join our Telegram channel

Full access? Get Clinical Tree
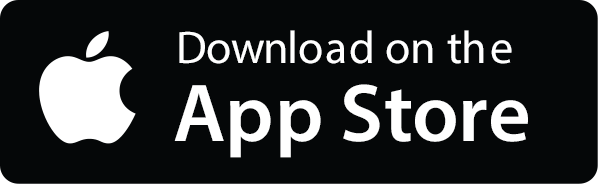
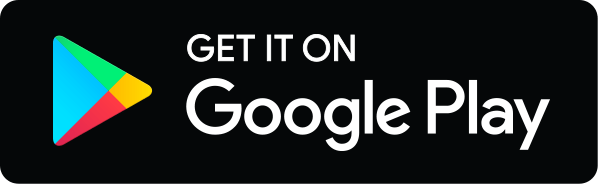