Metabolic disorders and disorders caused by exposure to a toxic agent portend a good prognosis, leading most of the time to a full recovery when recognized and treated promptly. Despite significant advances in critical care in the last 30 years, leading to improved survival of affected patients, little advance has been made in the treatment of acute neurological problems in the pediatric population. Metabolic disorders represent an exception to this statement, due to the development of new diagnostic techniques combined with a better understanding of the pathophysiology of such disorders, which have lead to the application of life-saving therapeutic tools and reducing the severity of brain injury. Examples include hemodialysis for the treatment of urea cycle disorders1 (eg, citrulinemia). In addition, significant advances in communication have made more readily available consultative expertise through academic centers worldwide. Access to the Internet has allowed most practitioners managing patients with a toxic or metabolic encephalopathy to maintain the highest standards of treatment, reduce injury, improve survival, and reverse changes in the penumbra zone of the affected areas of the brain.
This chapter is an approach to the different etiologies causing a metabolic derangement leading to a toxic or metabolic encephalopathy, and classified according to the age of the patient. Also, this chapter provides a wide overview of the pathogenesis, clinical approach, and treatment of patients affected with such disorders.
Toxic and metabolic encephalopathies refers to a group of disorders characterized by changes in the level of consciousness due to an exogenous or endogenous substance causing a metabolic derangement in the normal neuronal activity and leading to a transient or permanent damage of neuronal pathways (see Figure 20-1). The source of the injurious substrate can be from (1) a malfunctioning organ such as the liver or kidney, (2) an outside source such as a poisoning, or (3) deregulation of internal homeostasis as seen in primary or secondary electrolyte disturbances. Either pathway leads to compromise of autoregulation of the blood-brain barrier or internal brain homeostasis. Several etiologies are included in this syndrome and classified in two groups: congenital and acquired disorders.2 Causes of acquired disorders are listed in Table 20-1 and include exogenous poisoning (eg, methanol, ethanol, and ethylene glycol), diabetes mellitus, uremia, and late-onset congenital enzymatic deficiencies. Causes of congenital disorders leading to a toxic metabolic encephalopathy can be divided into early and late onset, based on the clinical presentation, and include organic acidurias, congenital glycosylation disorders, and neurotransmitter deficiencies3 (Table 20-2). For better understanding, definitions of several terms used when describing patients with altered mental status have been summarized in Table 20-3.
Figure 20-1.

Pathogenesis of ischemic neuronal death. Ischemia deprives the brain of metabolic substrates, especially glucose, making it impossible for cells to carry out energy-dependent functions such as the maintenance of trans-membrane ion gradients. Loss of these gradients depolarizes cell membranes, leading to the influx of calcium through voltage-gated calcium channels and triggering the release of neurotransmitters such as glutamate from presynaptic nerve terminals. Glutamate binds to receptors on the postsynaptic neuronal membrane to activate the influx of sodium and calcium. This sets in motion a cascade of biochemical events that causes cellular swelling, injures mitochondria, generates toxic free radicals, and activates proteases, nucleases, and other enzymes. Depending on the severity and duration of ischemia, neurons may die rapidly, from necrosis, or more gradually, from programmed cell death or apoptosis. Necrotic cell death is characterized by shrinkage of the nucleus (pyknosis), early loss of membrane integrity, structural changes in mitochondria, and eventually cellular lysis. Programmed cell death (PCD) depends on the synthesis of new proteins. Apoptosis, one form of PCD, is associated with margination of nuclear chromatin, relative preservation of cell membrane and mitochondrial integrity, and the formation of membrane-bound extracellular blebs (apoptotic bodies). Necrosis and PCD can coexist in different regions of an ischemic lesion. (Reproduced with permission from Aminoff MJ, Greenberg, DA, Simon RP: Clinical Neurology, 6ed. McGraw-Hill, Inc. New York, 2005. Figure 9-3.)
In the newborn, differential diagnosis considerations include two categories: disorders of glucose/ion metabolism and genetically programmed congenital errors of the metabolism. Disorders of glucose/ion metabolism are generally correctable if suspected and diagnosed promptly. Three important disorders in this category are (1) salt-wasting syndromes, (2) congenital hypothyroidism, and (3) vitamin D-dependent rickets. There are two different groups of genetically programmed congenital errors of metabolism that we classify based on clinical presentation: (1) acute-onset neurological derangement and (2) static encephalopathies of prenatal onset such as peroxysomal disorders. Several classifications have been attempted to better grade the severity of the symptoms. We have developed our own classification based on clinical findings and degree of impairment (Table 20-4).
System | Mild | Moderate | Severe |
---|---|---|---|
Upper cortical function | Lethargy/obtundation | Stupor | Coma |
Brainstem | Hypertension/tachycardia | Hypotension | Absent protective reflexes |
White matter symptoms | Hyper/hyporeflexia | Hyper/hypotonia | Spasticity, contractures |
Gray matter symptoms | — | Clinical seizures | Subclinical seizures |
Disorders of glucose and ion metabolism should be considered in the differential diagnosis of patients with altered level of consciousness. Under normal circumstances, glucose is the main source of energy for the brain. The brain is extremely susceptible to hypoglycemia. This is due to the fact that brain glucose is only 25% of the total blood glucose. Besides, there are no significant sources of stored glucose in the form of glycogen, as happens in cardiac or skeletal muscle. The central nervous system better tolerates hyperglycemia than hypoglycemia. However, hyperglycemia can cause significant neurological symptoms.
After having a load of glucose, the pancreas releases large amounts of insulin that regulate the glucose metabolism. Several other mechanisms are triggered after food ingestion, including storage of glucose in the liver as glycogen (glycogenosis). The human body has enough glycogen to maintain the blood glucose concentration between 80 and 100 mg/dL for 24 to 36 hours after having a last meal. Then, generation of glycogen (gluconeogenesis) takes over as the main mechanism for glucose control. Alanine and glutamate as well as lactic and pyruvic acid are well-known substrates for energy formation in this setting. The liver is the main organ involved in the process of maintaining glucose during starvation. After the hepatic sources for glucose have been depleted, the kidneys and other organs could contribute up to 50% of basal glucose. This explains why several organs are clinically involved during the severe hypoglycemic stage.
The mean cerebral blood flow is 50 mL/100 g of brain tissue. This flow provides large amounts of glucose to the brain if the peripheral glycemia is between 5 and 6 mmol/L. A cerebrospinal glucose transporter protein maintains appropriate CSF glucose level. Once in the brain, the glucose is metabolized into ATP by two main pathways: glycolisis and the Krebs cycle. Detailed review of both processes is beyond the scope of this chapter. However, faulty glycolitic mechanisms are responsible for significant systemic and neurological symptoms. A congenital disorder characterized by seizures and developmental delay has been identified—glucose transporter deficiency. In this disorder, cerebrospinal fluid (CSF) glucose is low due to a faulty glucose transporter protein. A ketogenic diet appears to be one of the main therapeutic tools available for better seizure control in this population. However, a ketogenic diet does not appear to modify other aspects of the disorder, such as developmental delay. Genetic testing is available for patients suspected to have glucose transporter deficiency.
The most common causes of hypoglycemia are (1) overuse of glucose, as seen in carnitine deficiency, sepsis, prediabetic state, and oral hypoglycemic abuse; (2) underproduction of glucose, as seen in enzymatic disorders6 (glucose-6-phosphatase deficiency, glycogen phosphorylase deficiency, glycogen synthetase deficiency, pyruvate carboxylase deficiency, and phosphoenolpyruvate carboxykinase deficiency); and (3) hormonal imbalances (eg, growth hormone). Maternal use of hypoglycemic medications or neonatal sepsis are the most common causes in the newborn period. Salycilate overdose and alcohol intoxication should be ruled out in the teenage population. There are three hypoglycemic syndromes: acute, subacute, and chronic.
Patients presenting in the acute state are usually restless and complain of dizziness or nervousness. Most of the time, patients presenting with an acute hypoglycemic state have a past history of diabetes mellitus. The most common cause of hypoglycemia in this group of patients is due to overdose of insulin or oral hypoglycemic medications. Symptoms usually resolve with a loading dose of IV glucose.
The subacute syndrome is characterized by obtundation, slowness of thought process, and amnesia. It is not uncommon to find hypothermia. The diagnosis is confirmed by checking the blood glucose during one of the episodes.
Chronic hypoglycemia is an uncommon situation, and when suspected, an insulinoma or excessive use of antihyperglycemic medications should be considered. Unlike the two prior groups, patients with chronic hypoglycemia usually do not respond to boluses of intravenous glucose.
The most common cause of hyperglycemia in the pediatric population is diabetic ketoacidosis (DKA). This condition is usually triggered by an infection in an otherwise well-controlled diabetic type I patient, or can present as new-onset hyperglycemia in an otherwise previously healthy patient, prompting a visit to the emergency room due to obtundation or other changes in the level of consciousness. In addition to polyurea and polydipsia, symptoms include anorexia, nausea, vomit, dehydration, and coma. This is a disorder of severe deficit of insulin. In the absence of insulin, the body’s peripheral glucose uptake and glycogen formation are inhibited, leading to excessive lypolisis, glycogenolysis, and hyperglycemia. Treatment with insulin enhances entry of glucose in to the cell, glycogen formation, and reduction of the rate of ketone body formation. Supportive treatment is based on the judicious correction of hypernatremia and dehydration. An excessively rapid correction of hypernatremia and hyperosmolarity may lead to cerebral edema. It is believed that a clinically silent form of cerebral edema may be present in most cases of DKA. When clinically indicated, further evaluation, including placement of an intracranial pressure monitor, should be considered to better guide therapy. The treatment of cerebral edema in this particular setting is based in decreasing the elevated intracranial pressure, including intubation, mechanical ventilation, elevation of the head, hyperoxygenation, and measures aiming to avoid Valsalva maneuvers such as treating constipation and avoiding excessive gag or suction stimuli to the throat or the trachea. The use of manitol to facilitate osmogenic diuresis is one of the pharmacologic tools available when treating patients with cerebral edema.
When hyponatremia is confirmed, serum osmolarity must also be checked, since hyponatremia and hypoosmolarity are both frequently associated. Causes include renal loss (renal disease, adrenal insufficiency), gastrointestinal losses (diarrhea, vomit), sequestration (peritonitis), hemodilution, sickle cell disease, hyperosmosis, hyperglycemia, and inappropriate secretion of anti-diuretic hormone (SIADH). In addition to hyponatremia, the diagnosis of SIADH should be suspected in the normovolemic or hypervolemic patient with documented normal renal function.
Water restriction is usually the treatment for mild cases. More severe cases require hypertonic solutions and diuretic treatment. Excessive overcorrection may lead to hypernatremia and central pontine myelonolisis (CPM). This last disorder is characterized by acute demyelination of the pontine structures due to fast correction of hyponatremia. Patients with CPM develop a myriad of symptoms including changes in sensorium, lower cranial nerve palsies, and diffuse weakness. Serial and frequent serum electrolytes monitoring and avoidance of excessive administration of hypertonic, hypernatremic solutions are needed to prevent this irreversible and permanent complication. Brain MRI typically show areas of demyelination in the mamillary bodies and around the fourth ventricle (see Figure 20-2).
Figure 20-2

Central pontine myelinolysis. Axial T2-weighted MR scan through the pons reveals a symmetric area of abnormal high signal intensity within the basis pontis (arrows).(Reproduced with permission from Fauci AS, Kasper DL, Braunwald E, Hauser SL, Longo DL, Jameson JL, Loscalzo J: Harrison’s Principles of Internal Medicine, 17th ed. McGraw-Hill, Inc. New York, 2008. Figure 269-6.)
Hyperosmolarity should be suspected in diabetic patients or in an otherwise healthy person presenting with severe dehydration and acute intracranial bleeding. Bleeding occurs due to tearing of the bridging vessels at the level of the dural sinuses. The deficit of water should be estimated carefully and replacement therapy should be started promptly, bearing in mind that a fast correction may lead to brain swelling. In general, it is recommended to attempt correction by the oral route, aiming to replace the deficits slowly.
This group of disorders is usually associated with a deficient parathyroid gland function. In normal circumstances, about 50% of the serum calcium is bound to protein and therefore considered metabolically in quiescent form. The other 50% is ionized and therefore metabolically active. Hyperthyroidism may be associated with hypercalcemia and should be part of the differential diagnosis when evaluating patients with idiopathic hypercalcemia. Symptoms of hypercalcemia range from altered consciousness, weakness, and nausea and vomitting to coma. The most common causes of hypercalcemia are sarcoidosis, thiazide diuretics, vitamin D intoxication, and excessive calcium intake. Treatment includes hydration, diuresis, and supportive treatment.
Hypothyroidism is usually associated with hypocalcemia. Clinically, patients complain of paresthesias around the mouth and fingers, cramps, headaches, and seizures. The presence of positive Chvostek and Trousseau signs documented in the physical examination should raise the index of suspicion. Chovstek sign consists of tapping the thenar region and looking for a spasmodic contracture of the hand. Trousseau sign is usually elicited in the hypocalcemic patient when the cuff of a blood pressure monitoring device is applied to the arm and inflated above the diastolic pressure until a tonic contracture of the arm is present. Both findings are indicative of hypocalcemia. Treatment is based in administration of calcium to correct the deficit.
Hypothyroidism can also present clinically as idiopathic intracranial hypertension, formerly known as pseudotumor cerebri. Postural headaches, sixth cranial nerve palsy, and blunted optic disk borders are typical findings. Optic disk edema is a common finding. Figure 20-3 shows blunting of the optic disc borders and elevation of retinal blood vessels. Patients may complain of having blurry or double vision and early morning headaches. The pathophysiology of benign increased intracranial pressure syndrome is not well understood, but a mismatch between excessive CSF production by the choroid plexus and deficient CSF reabsorption mechanisms has been postulated. The diagnosis is suspected when the patient has a focal neurological exam such as optic disk edema or sixth cranial nerve palsy and has a normal brain MRI.The spinal tap is both diagnostic and therapeutic. An opening spinal pressure above 25 ml H20, within the right clinical setting, is confirmatory. Then, drainage of cerebro-spinal fluid is the treatment of choice. The aim is to drain enough CSF to bring the closing pressure to half the opening pressure. A comprehensive list of etiologies causing idiopathic increased intracranial pressure is given in Table 20-5. In nearly 60% of the cases, the cause is not established (idiopathic). When established, the treatment should be aimed to treat the cause. Early diagnosis and treatment should be established promptly to prevent further injury to the optic nerve and subsequent permanent blindness. Carbonic anhydrase inhibitors such as acetazolamide are usually the first line of therapy by decreasing the production of CSF. Other potential options are loop diuretics such as furosemide or corticosteroids such as prednisone. Patients with idiopathic intracranial pressure that fail medical treatment should be referred to the neurosurgeon for a decompressive procedure of the optic nerves such as optic nerve fenestration. Consultative expertise obtained from a neuro-opthalmologist should also be sought to better guide the therapy.
Figure 20-3

Mild papilledema. The disk margins are blurred superiorly and inferiorly by the thickened layer of nerve fibers entering the disk. (Reproduced, with permission, from Vaughan DG, Asbury T, Riordan-Eva P [editors]: General Ophthalmology, 15th ed. Originally published by Appleton & Lange. Copyright © 1999 by The McGraw-Hill Companies, Inc.)
Infectious |
Lyme disease |
Rocky Mountain spotted fever |
Cat scratch disease |
Metabolic |
Hyperthyroidism |
Hypoparathyroidism |
Pregnancy |
Adrenal insufficiency |
Diabetic ketoacidosis |
Systemic Disorders |
Iron deficiency anemia |
Systemic lupus erythematosus |
Leukemia |
Malnutrition |
Guillain-Barré syndrome |
Drugs |
Vitamin A deficiency/excess |
Oral contraceptive pills |
INH |
Vitamin D deficiency |
Thyroid replacement hormones |
Corticosteroids |
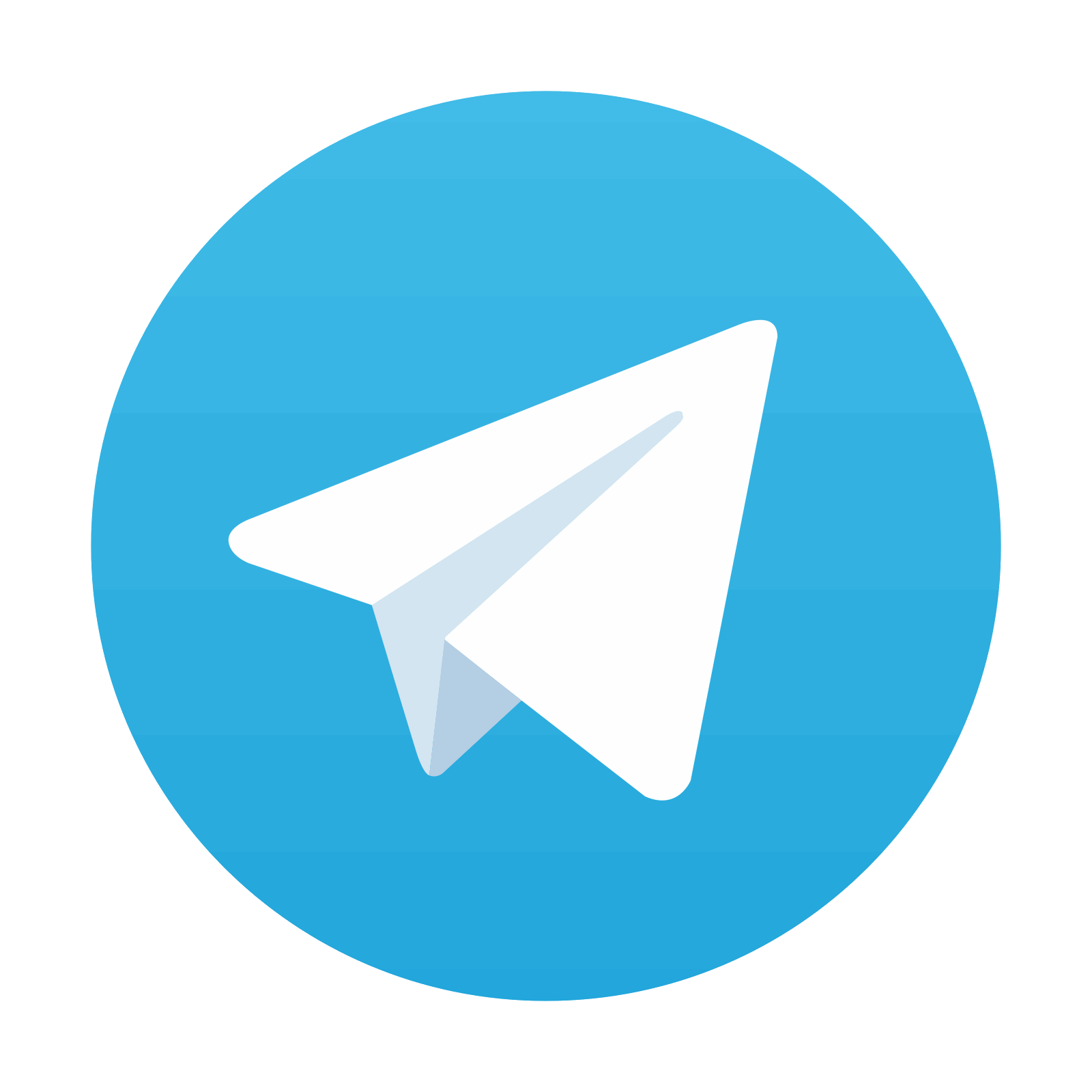
Stay updated, free articles. Join our Telegram channel

Full access? Get Clinical Tree
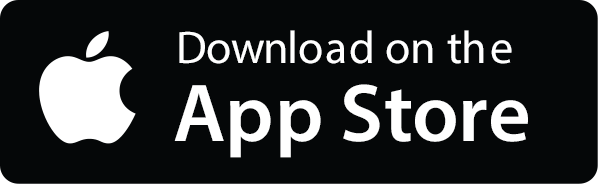
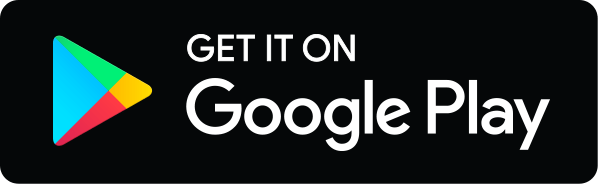
