Fig. 1
A gradient of grid patterns spacing from dorsal (small grids) to ventral (larger grids) cells of the MEC poles, combined to a topographically organized anatomical connectivity between MEC and ventral-dorsal hippocampus results in dorsal (respectively ventral) hippocampus receiving predominant connections from dorsal(respectively ventral) MEC. This could explain the smaller size of the place fields of the dorsal hippocampal cells, compared to ventral ones. Arrows’ thickness figures connection weights (Adapted from Solstad et al. [6] and Gaussier et al. [5])
The properties of hippocampal place fields vary according to the exact location where place cells are recorded (i.e. dentate gyrus: DG, CA3, CA1). However place cells share the spatial specificity of their strongly location-related signal, and exquisite sensitivity to the environment and context, either spatial or temporal. Different models have mechanistically demonstrated how the combination of grid cells with different spatial frequencies give rise to DG place fields of different sizes [5, 6]. Further, in an entorhinal-hippocampal loop model [5, 7, 8], spatial and temporal representations combine in CA3 and CA1 to provide a dynamic representation of the animal’s navigation, under the form of transitions from place to place, rather than pure locations, by associating allothetic and idiothetic information. Transitions form the building blocks for sequence and trajectory encoding.
Indeed, beyond the hippocampal stage, and in particular in deep MEC layers, strict spatial specificity is lost, in favor of trajectory encoding. In particular, in inverted W or in alternating T maze experiments, the firing field of EC pyramidal cells expands to an entire maze arm (Fig. 2). A supra-ordinate factor, like heading direction or path context could integrate successive firing fields in cells combining place and direction or task information.
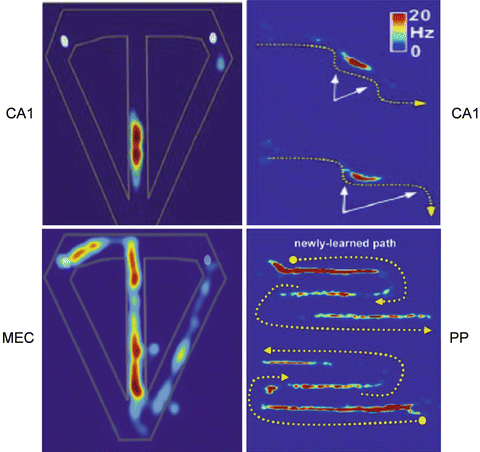
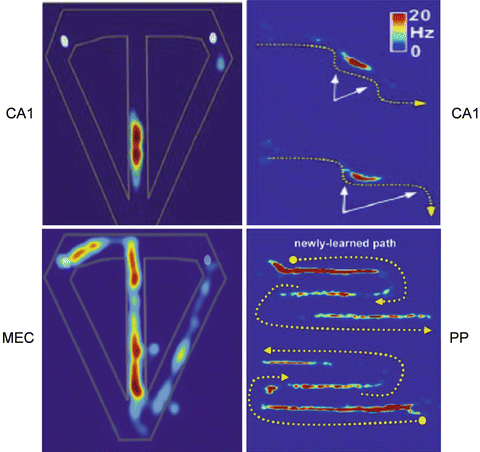
Fig. 2
Place fields in different anatomical structures: CA1, MEC, or Posterior Parietal cortex (PP) in different maze experiments. Left: discharge rate-maps for CA1/MEC neurons in rats performing a T-maze alternation task for right-to-left trials, to get reward at the white hole. Right: same rate-maps for outbound (CA1) and outbound (upper plots) and inbound (lower plot) (PP) traversal of a newly-learned path shown by broken yellow lines. For each of the two traversals the PP neuron discharges along each path’s entire first segment and the final half of the last segment. While the spatial specificity of the place fields of CA1 place cells remains very strong whatever the geometry of the maze, this specificity is partly lost in MEC and furthermore in PP neurons, in favor of a more functional, task-related significance (Adapted from Eichenbaum et al. [9] and Nitz [10])
From HS, spatio-temporal information may be routed to the posterior parietal (PP) cortex through the retrosplenium. In PP neurons, the trajectory coding undergoes further abstraction, becoming trajectory, size and direction independent (Fig. 2); mapping the order of multiple navigational epochs in a route; integrating location and self-movement information.
The head direction system which pervades all these structures seems to provide coherence between allo-centric and ego-centric representations.
Functional dissociation between representation and implementation systems can result from at least two different rationales: the process has basically nothing to do with action, like music listening, meditation in humans…; the process relates to action, but the brain is in a memory or planning mode, and implementation is not relevant, like in quiescent phases or sleep, in rodents; in this case, it seems that two opposite modulations take place: first, activation of the cortical areas where memories are recorded, and simultaneously inhibition of the subcortical implementation systems.
2 Implementation Structures
The phylogenetic evolution of the distinct cortical structures (archi-, paleo-, meso-, neo-cortex) takes place through the dual process of increased complexity (e.g., number of layers) and inclusion of older structures into more recent ones. The same type of inclusion is found within ventral and dorsal (neo)striatum, through (older) patch and (recent) matrix compartments, such that authors suppose that cortical evolution may have influenced striatal evolution. This could account for the tight functional links between the two structures. Indeed, there is an anatomic, hodologic, and functional coherence between the different compartments, limbic, associative-cognitive and sensori-motor, at all levels of the cortico-ganglio-basal loops: cortex, striatum, thalamus.
Following the characterization of cortico-striato-thalamic loops [1], the functions of the different striatal compartments have been progressively refined, particularly in relation to reinforcement learning, pavlovian conditioning, and instrumental conditioning in goal oriented behavior. Thus, the nucleus accumbens (ACu) has been more particularly associated to Pavlovian conditioning and stimulus-outcome (SO) association. The core is involved in preparatory CR and anticipatory approach; core lesions impair drug-seeking behavior triggered by drug-associated reinforcers. The shell mediates consummatory CRs and hedonic URs; its lesions preserve drug-seeking acquisition.
Similarly, dorso-medial striatum (DMS), involved in instrumental conditioning, during goal-oriented actions, plays a long-lasting role in the acquisition and expression of action-outcome (AO) learning, in clear contrast with the short-lived function of the medial prefrontal cortex (mPFC) in the same conditions. It contributes to the selection and learning of situations representing valuable parts of the task. The dorsolateral striatum (DLS) transforms goal-oriented repetitive behaviors into habits and skills, and contributes to learning behavioral sequences in general.
However, this view of dedicated functions of the striatal components of the loops can only be partial if the spiraling connections between the different loops are not taken into account [2]. Striatal neurons send direct inhibition to DA neurons from which they receive projections, and also disinhibitory connections to DA neurons projecting to the distinct next striatal area, allowing unidirectional activation-propagation from limbic to associative to motor loops (Fig. 3). A learned conditioned stimulus (CS) could simultaneously suppress a ventral tegmental area (VTA) DA learning signal, and potentiate a substantia nigra compacta (SNc) DA signal in the next cognitive loop. Thus, limbic striatal loops are in a position to control learning and processing within associative and sensori-motor cortico-striatal networks.