9
Transcranial Magnetic
Stimulation for Disorders
Other Than Depression
Transcranial magnetic stimulation (TMS) is still experimental when it comes to treating disorders other than depression, but its success for depression has led many to wonder whether it might also be effective for other conditions. After all, “treatment resistance” is common not only in depression but also in schizophrenia, obsessive-compulsive disorder (OCD), posttraumatic stress disorder (PTSD), chronic pain, and many other illnesses. Current pharmacological approaches for these conditions have limitations, and electroconvulsive therapy may produce significant side effects for some patients.
Developing stimulation-based treatments for any disorder begins with delineating the underlying neural circuitry of the disorder. Only with this understanding can researchers identify the key brain regions to target that are 1) involved in the pathophysiology of the disorder and 2) accessible to the depth of stimulation being used. Foundational knowledge of the relevant circuit(s) is also important for hypothesizing how other distinct—and sometimes distant—brain regions may be affected by stimulation.
In this chapter, we discuss how TMS-based treatments show promise for application to complex psychiatric illnesses.
Schizophrenia
AUDITORY VERBAL HALLUCINATIONS
Auditory verbal hallucinations are a hallmark positive symptom of schizophrenia. Imaging studies link hyperactivity of left-sided auditory and linguistic areas of the brain, including the left temporoparietal cortex, left superior temporal cortex, and Broca’s area, to the pathophysiology of such hallucinations, providing a potential target for TMS (Silbersweig et al. 1995; see Figure 9–1).
The first report of TMS treatment of auditory hallucinations involved three patients with schizophrenia (Hoffman et al. 1999). Slow-frequency 1-Hz stimulation over the left temporoparietal junction (midway between T3 and P3 electroencephalogram electrode sites) was performed for a maximum of 16 minutes, leading to a decrease in the frequency and intensity of hallucinations. A larger, controlled study lasting 9 days again showed substantial improvement following 1-Hz stimulation over the left temporoparietal cortex. More than half of the patients who improved showed sustained benefit 15 weeks later (Hoffman et al. 2003).
Over 30 studies involving more than 700 patients have since been completed. So far, only 1-Hz stimulation of the left temporoparietal cortex has consistently demonstrated efficacy (Slotema et al. 2014). It is worth noting that some patients improve with short courses of treatment and that improvement may often be sustained by periodic retreatment when symptoms emerge (Fitzgerald et al. 2006).
Figure 9–1. Schizophrenia circuit.
See Plate 1 to view this figure in color.
Left-sided lateral view of the brain outlining regions of interest in schizophrenia. The auditory and linguistic areas of the brain responsible for speech, including the right and left superior temporal cortex, Broca’s area, and the left temporoparietal area, are implicated in auditory hallucinations. The negative symptoms of schizophrenia are associated with hypoactivity of the dorsolateral prefrontal cortex (DLPFC). Image was visualized with the BrainNet Viewer (Xia et al. 2013).
NEGATIVE SYMPTOMS
Antipsychotic agents of all generations are less effective in reducing negative symptoms, such as flat affect, alogia, anhedonia, and avolition, than positive symptoms. Similar to what is seen in depression, these negative symptoms are associated with hypoactivity of the dorsolateral prefrontal cortex (DLPFC), suggesting that high-frequency stimulation may be of benefit (Stanford et al. 2011). Although a number of studies have used this approach and many of them have found evidence of improvement, they are limited by small sample sizes and short durations of treatment. Another potential confounding variable is that improvement in depression may be misinterpreted as improvement in negative symptoms.
Cole et al. (2015) assessed 10 studies (N=257) in which high-frequency TMS targeted the left DLPFC with the aim of increasing cortical excitation and inducing dopamine release. Effect sizes ranged from 0.58 to 0.63 when all trials (including open-label trials) were included. Because effect sizes for antipsychotic medication treatment of negative symptoms range from 0.17 to 0.21, TMS may be an appropriate treatment for some patients (Slotema et al. 2010).
CLINICAL VIGNETTE
The patient is a 24-year-old single woman with schizophrenia. She was living at home with her parents and being treated at a clinic specializing in treatment of early-onset psychosis. Symptoms developed during her first year of college, when she began hearing voices and believed that her thoughts were controlled by robots. She was hospitalized and unable to continue her education. She abandoned her interests and withdrew from family and friends. Her thinking was disorganized and her concentration poor. She could not sit through a movie and had not driven for several years. In public settings, she displayed poor impulse control and was often inappropriate, intrusive, and prone to arguments with strangers.
At the time of evaluation, she was taking lurasidone 60 mg/day. Previous treatments included risperidone, olanzapine, and aripiprazole, which were of limited benefit and poorly tolerated because of weight gain and sedation. Her Positive and Negative Syndrome Scale (PANSS) score was 91 and her Quick Inventory of Depressive Symptomatology—Self-Report (QIDS-SR) score was 7. She denied auditory hallucinations, and there was no clear evidence of delusions, but she was highly distractible, restless, and unable to sit still for more than a few minutes.
She underwent a course of 26 left-sided high-frequency treatments at 10 Hz. During the first week of treatment, she was restless, was easily distracted, and could not remain in the treatment chair for more than about 10 minutes at a time. By the end of her second week, she was able to sit through an entire treatment without interruption. During week 4, she drove herself to treatment with one of her parents in the car. At the end of treatment, her restlessness had completely subsided, her focusing ability had significantly improved, and she had resumed her interest in music and dance. She took responsibility for scheduling her own appointments and was planning to resume college on a part-time basis. Her PANSS and QIDS-SR scores were 56 and 3, respectively. Improvement was sustained when she was seen in follow-up 12 weeks later.
Obsessive-Compulsive Disorder
Up to 60% of patients with OCD experience persistent symptoms despite medications and psychotherapy (Pallanti and Quercioli 2006). Evidence suggests that the orbitofronto-striato-pallido-thalamic circuit underlies the core symptoms of OCD, including unwanted, persistent, anxiety-provoking obsessions and repetitive, time-consuming compulsions (Berlim et al. 2008). This well-defined circuitry involves the DLPFC, orbitofrontal cortex, medial prefrontal cortex, anterior cingulate gyrus, supplementary motor area, and basal ganglia (Del Casale et al. 2011; see Figure 9–2). All of these network “nodes” are potential targets for TMS, and many have been directly investigated with variable and sometimes conflicting results.
Figure 9–2. Obsessive-compulsive disorder (OCD) circuit.
See Plate 2 to view this figure in color.
Left-sided lateral view of the brain with medial structures also visible. Evidence suggests that the orbitofronto-striato-pallido-thalamic circuit underlies the core symptoms of OCD. This well-defined circuitry involves the dorsolateral prefrontal cortex (DLPFC), orbitofrontal cortex (OFC), medial prefrontal cortex (mPFC), anterior cingulate cortex (ACC), supplementary motor area (SMA), and basal ganglia, composed of the caudate and putamen (Del Casale et al. 2011). Image was visualized with the BrainNet Viewer (Xia et al. 2013).
One of the earliest studies of TMS in treating OCD (Greenberg et al. 1997) suggested that high-frequency stimulation over both the left and right DLPFCs could reduce compulsive symptoms, but a later study (Sachdev et al. 2007) did not corroborate this finding. The active and sham groups did not differ on change in Yale-Brown Obsessive Compulsive Scale (Y-BOCS) or Maudsley Obsessive-Compulsive Inventory scores over 10 sessions, with or without correction for depression ratings. Over 20 sessions, there was a significant reduction in total Y-BOCS scores but not after controlling for depression. In general, high-frequency TMS protocols and DLPFC stimulation do not appear to be effective. There is evidence to suggest possible benefit from slow-frequency 1-Hz stimulation over the orbitofrontal cortex or supplementary motor area (Berlim et al. 2013); this may be explained by the inhibitory effects of low-frequency TMS on hyperactive orbitofronto-striatal circuits (Menzies et al. 2008).
At this time, there is insufficient evidence to confidently support the use of TMS in the treatment of OCD (Slotema et al. 2010) with figure-eight and other currently available TMS coils. New coil designs may be capable of stimulating the relevant circuits to ameliorate symptoms of OCD.
One study used an H coil (HAC or H7 coil) designed to target the medial prefrontal cortices and anterior cingulate cortices bilaterally in 41 patients with treatment-resistant OCD with moderate to severe symptoms. Treatments were administered after the patient’s individual symptoms were provoked, and electroencephalogram measurements during a Stroop task were utilized to assess changes in error-related activity. Improvements were measured using the Y-BOCS. Initially, the study had four arms, a high-frequency arm of 20 Hz, a sham 20-Hz arm, a low-frequency arm of 1 Hz, and a sham 1-Hz arm. During the interim analysis, there was no difference between the sham and 1-Hz arms, so the study was continued with just 20-Hz high-frequency and sham arms. At the end of the study, the response rate in the 20-Hz arm was much greater than in the sham group, and the improvements lasted for a month after treatments ended. Interestingly, the improvement in the high-frequency group correlated with an increase in error-related negativity during the Stroop task following the treatment, suggesting that the improvement is related to the ability of the H7 coil to modify activity in the anterior cingulate (Carmi et al. 2015; Zangen et al. 2016).
Posttraumatic Stress Disorder
PTSD is characterized by a learned fear (due to a past traumatic event) that becomes generalized (Mahan and Ressler 2012). Fear conditioning and its circuitry are well conserved across vertebrate species, with the limbic system being the center of fear and emotion processing in humans and animals. Altered activity in the limbic regions of the amygdala, hippocampus, and prefrontal cortex is demonstrated in patients with PTSD (Karsen et al. 2014; Mahan and Ressler 2012; see Figure 9–3).
Given the success of targeting the DLPFC for depression, most studies also target this region for PTSD. A review by Karsen et al. (2014) included eight small studies (N=133) in which the TMS sites were either the left, right, or both DLPFCs or the bilateral medial prefrontal cortices. No statistically significant effect size was found, however, possibly because of the small sample sizes. Of the targets assessed, there was a trend toward efficacy when stimulating the right DLPFC at low frequency with a greater total number of stimuli/pulses (Karsen et al. 2014).
Figure 9–3. Posttraumatic stress disorder (PTSD) circuit.
See Plate 3 to view this figure in color.
Right-sided lateral view of structures implicated in PTSD. Functional neuroimaging findings in PTSD support the hypothesis that the amygdala is hyperresponsive, and ventral portions of medial prefrontal cortex (vmPFC) and right dorsolateral prefrontal cortex are hyporesponsive. Hippocampal volume and function appear to be abnormal as well. Image was visualized with the BrainNet Viewer (Xia et al. 2013).
At this time, TMS treatment of PTSD remains experimental. Because many patients with PTSD also suffer from major depressive disorder, many will qualify for TMS therapy based on their mood disorder diagnosis and may also experience improvement in PTSD symptoms. Military veterans with combat- or operation-related PTSD may be referred to Department of Defense or Department of Veterans Affairs TMS research trials.
Addiction
Behaviors associated with addiction are complex and include preoccupation, craving, anticipation, bingeing, satiation, cessation, and withdrawal. The brain regions underlying each of these behaviors likely exert interconnected effects, working in concert to modulate different stages of the disorder (Koob and Volkow 2010). This results in a breadth of potential cortical targets for TMS therapy.
In a randomized controlled trial, Dinur-Klein et al. (2014) used deep TMS bilaterally over the lateral prefrontal and insular cortices to augment smoking cessation. A standard figure-eight TMS coil is unable to induce direct stimulation of deeper cortical areas, but using a specific H coil allows induction of a deeper electromagnetic field (see Chapter 10, “Current FDA-Cleared TMS Systems and Future Innovations in TMS Therapy”). In this study, 10 daily sessions of high-frequency deep TMS with an H-coil system reduced cigarette consumption, with the effect being most pronounced when treatment was applied following presentation of a smoking cue.
To date, the most common target for the treatment of addiction disorders is the left DLPFC at frequencies of 10–20 Hz (Grall-Bronnec and Sauvaget 2014; see Figure 9–4). A review of 18 studies involving approximately 400 patients with a variety of addictions, including nicotine, alcohol, recreational drugs, and food, suggests a role for TMS in reduction of craving (Grall-Bronnec and Sauvaget 2014). Assessment of these results needs to take into account the behavioral differences involved in these addictions; the significant heterogeneity in design, type, and target of TMS; and differences in final outcome measures across the 18 studies.
Chronic Pain
Pain perception is a multidimensional experience involving a widespread network of brain regions. Anatomical, electrophysiological, and functional imaging approaches have established the cortical areas that contribute to the perception of pain and that may be potential TMS targets for the treatment of chronic pain. A general conceptual framework divides the nociceptive network into lateral and medial components, which contribute to the sensory-discriminative and affective-motivational dimensions of pain, respectively (Melzack and Casey 1968). The cortical targets of the lateral system, involving afferent projections from the lateral thalamic nuclei, are mainly the primary and secondary somatosensory cortices. By contrast, the insula and anterior cingulate cortex are the main targets of the medial system and involve medial thalamic nuclei. In reality, pain processing is more complex. Connections exist between the cortical areas that receive direct nociceptive input and other cortical or subcortical brain regions (e.g., prefrontal cortex, motor cortex, periaqueductal gray matter) and are implicated in the cognitive-evaluative aspects of pain, pain modulation, and the integration of pain with other sensory modalities (Treede and Apkarian 2009; Vogt and Sikes 2009; see Figure 9–5). It is now well established that structural and functional abnormalities are evident in these brain areas across numerous chronic pain conditions (Davis and Moayedi 2013).
Figure 9–4. Addiction circuit.
See Plate 4 to view this figure in color.
Left-sided lateral view of potential transcranial magnetic stimulation (TMS) targets for addiction therapy. Damage to the insula has been associated with an increased ability to stop smoking, whereas cue activation of the medial prefrontal cortex (mPFC) has been seen in alcoholic patients. The most common target to date is the left dorsolateral prefrontal cortex (DLPFC), where TMS may have a role in craving reduction. The DLPFC has been studied in patients for a variety of addictions, including nicotine, alcohol, recreational drugs, and food. Image was visualized with the BrainNet Viewer (Xia et al. 2013).
TMS has been studied as a potential treatment for a variety of chronic pain syndromes, including fibromyalgia, neuropathic pain, facial pain, migraine, and irritable bowel syndrome, with several studies reporting significant pain reduction compared with sham treatment (Galhardoni et al. 2015). In general, high-frequency (10- or 20-Hz) stimulation to the primary motor cortex (M1) has yielded positive results (Johnson et al. 2006; Khedr et al. 2005; Lefaucheur et al. 2001; Pleger et al. 2004; Rollnik et al. 2002). The mechanism of action of M1 stimulation is not entirely understood, but there is reason to believe its analgesic effects rely on endogenous opioid systems (de Andrade et al. 2011). Some analgesic effects are also reported with high-frequency DLPFC stimulation in patients with phantom limb pain and fibromyalgia (Ahmed et al. 2011; Brighina et al. 2004; Short et al. 2011), but these effects likely involve a different mechanism (de Andrade et al. 2011). For both motor cortex and DLPFC stimulation, there is evidence to suggest that the analgesic effect depends on trans-synaptic stimulation of distant brain areas involved in pain integration and modulation (Hasan et al. 2014). For example, in an animal model, M1 stimulation resulted in decreased thalamic activity bilaterally and increased periaqueductal gray activity (França et al. 2013; Pagano et al. 2012). Moreover, the precise region of stimulation within these broad brain areas may have different effects. For example, homotopic analgesia (i.e., analgesia corresponding to the body part associated with a specific region of cortex) is observed with electrical transdural M1 stimulation in rats (Maarrawi et al. 2007).
Plate 5. (Figure 9–5) Pain circuit.
See Plate 5 to view this figure in color.
Left-sided view of both lateral and medial structures involved in chronic pain. Pain processing is complex; however, a general conceptual framework has been adopted in which the structures involved are divided into lateral and medial components, which contribute to the sensory-discriminative and affective-motivational dimensions of pain, respectively. The lateral system includes the primary and secondary somatosensory cortices, whereas the insula and anterior cingulate cortex (ACC) are the main targets of the medial system. The thalamus plays a processing role in both systems. Image was visualized with the BrainNet Viewer (Xia et al. 2013).
Dementia
Dementia is a worldwide public health problem. The number of cases is expected to exceed 65 million by 2030 and is likely to double by 2050 (Rutherford et al. 2013). Alzheimer’s disease (AD) accounts for more than half of all cases of dementia. Current drug treatments are of limited and temporary benefit.
Reports of TMS improving cognitive function began to emerge shortly after its introduction as a research tool. Over 60 studies with normally functioning, healthy subjects have reported significant improvement in speed and accuracy in a variety of tasks involving perceptual, motor, and executive processing (Luber and Lisanby 2014). In addition, a number of studies have specifically investigated TMS as a treatment for AD (Guerra et al. 2011) and other forms of dementia, including dementia with Lewy bodies (Liang et al. 2010) and vascular dementia (Pennisi et al. 2011).
High-frequency bilateral DLPFC stimulation has been shown to improve the accuracy of action naming and object naming in moderate to severe AD (Cotelli et al. 2008). Episodic memory and information processing speed have also been shown to improve following high-frequency TMS to the left DLPFC in patients with AD (Haffen et al. 2012).
High-frequency repetitive TMS has also been combined with cognitive training (rTMS-COG) using a proprietary medical device, the NeuroAD System (Neuronix, Yokneam, Israel). A prospective, randomized, double-blind, placebo-controlled study (Rabey et al. 2013) included 27 patients with AD (18 and 8 in the treatment and sham groups, respectively, and 1 dropout). The participants were categorized into groups with mild AD (Mini-Mental State Examination [MMSE] score=21–26) or moderate AD (MMSE score=18–20). The rTMS protocols were configured for six cortical areas (both dorsolateral prefrontal and parietal somatosensory association cortices and Broca’s and Wernicke’s areas). Patients received 10-Hz TMS at 90%–110% intensity for 5 days/week for 6 weeks. Neuropsychological assessments were performed using the Alzheimer’s Disease Assessment Scale Cognitive subscale (ADAS-Cog), Clinical Global Impression of Change (CGIC) Scale, and MMSE before, immediately after, and 6 weeks after the end of rTMS-COG treatment. Significant improvement in cognition was observed among the patients with AD. Mean ADAS-Cog scores among those with mild AD improved by 5.46 points, which is remarkable considering that cholinesterase inhibitors result in an average improvement of 1.8 points over 12 weeks (Lee et al. 2015) and 2.7 points over 6 months (Birks 2006).
The NeuroAD System with its associated treatment protocol (stimulation of multiple cortical areas combined with cognitive training) is commercially available in Europe but is still considered investigational in the United States as of this writing. Although TMS is not a cure, it does appear to modify disease progression and may represent an important advance in AD treatment.
Other Disorders
Craving not only is a characteristic feature of addiction disorders but also plays a prominent role in bulimia nervosa and other conditions involving bingeing behaviors. Similar patterns of activation have been identified in addiction and obesity (Van den Eynde et al. 2010). High-frequency stimulation over the DLPFC may reduce these cravings or inhibit their development altogether (Uher et al. 2005), although whether this translates to reduced consumption has not yet been studied.
Previous studies of autism spectrum disorder have suggested a morphological disturbance resulting in a deficit of inhibition that leads patients with autism to process information excessively and be unable to successfully differentiate between target and irrelevant stimuli (Sokhadze et al. 2009). This lack of inhibition suggests a possible role for low-frequency TMS. For example, when assessed in combination with measured event-related potentials, low-frequency TMS minimized early cortical responses to irrelevant stimuli and increased responses to relevant stimuli (Sokhadze et al. 2010).
Other neurological disorders currently under study are those that have an associated motor aspect. These include Tourette syndrome, Parkinson’s disease, epilepsy, multiple sclerosis, and traumatic brain injury. Research is also under way involving treatment of migraine and dystonia and rehabilitation following stroke.
Conclusion
Much research is still needed to discover various potential uses of TMS. Before successful TMS treatments can be developed, relevant neural circuitry of a disorder of interest must be understood. Once this circuitry is identified, we can then target the regions involved. The details of how TMS exerts its therapeutic effects are still unclear. Further investigation is needed to understand how TMS affects neural circuitry through long-term potentiation and long-term depression. This can be achieved with careful symptom and behavioral assessment and further augmented with the addition of neuroimaging (e.g., functional magnetic resonance imaging, positron emission tomography) and other techniques. Finally, a relatively unexplored use of TMS may be as a means to improve diagnostic methods and/or identify subtypes of disease. For example, the ability of TMS to create temporary and reversible disruptions in normal neuronal activity (“virtual lesions”) on demand could serve as a diagnostic testing mechanism that psychiatry currently lacks.
KEY CLINICAL POINTS
• At this time, TMS has been cleared by the U.S. Food and Drug Administration for adult patients with major depression who have not responded to one or more antidepressant medications. All other uses are considered experimental or off-label and are not generally covered by health insurance.
• There is evidence from randomized controlled studies and meta-analyses to support the off-label use of TMS in the treatment of refractory negative symptoms of schizophrenia.
• Evidence from several open-label studies and one randomized controlled study suggests that a modified form of TMS combined with cognitive training may slow the cognitive decline of patients with mild to moderate Alzheimer’s disease.
• There is insufficient evidence to support the off-label use of TMS in obsessive-compulsive disorder, posttraumatic stress disorder, and other conditions, but ongoing research and new technological developments may yield new insights and opportunities.
References
Ahmed MA, Mohamed SA, Sayed D: Long-term antalgic effects of repetitive transcranial magnetic stimulation of motor cortex and serum beta-endorphin in patients with phantom pain. Neurol Res 33(9):953–958, 2011 22080997
Berlim MT, Fleck MP, Turecki G: Current trends in the assessment and somatic treatment of resistant/refractory major depression: an overview. Ann Med 40(2):149–159, 2008 18293145
Berlim MT, Neufeld NH, Van den Eynde F: Repetitive transcranial magnetic stimulation (rTMS) for obsessive-compulsive disorder (OCD): an exploratory meta-analysis of randomized and sham-controlled trials. J Psychiatr Res 47(8):999–1006, 2013 23615189
Birks J: Cholinesterase inhibitors for Alzheimer’s disease. Cochrane Database Syst Rev (1):CD005593, 2006 16437532
Brighina F, Piazza A, Vitello G, et al: rTMS of the prefrontal cortex in the treatment of chronic migraine: a pilot study. J Neurol Sci 227(1):67–71, 2004 15546593
Carmi L, Alyagon U, DAR R, et at: Deep transcranial magnetic stimulation (TMS) in obsessive compulsive disorder (OCD) patients. Eur Psychiatry 30 (suppl 1):794 2015
Cole JC, Green Bernacki C, Helmer A, et al: Efficacy of transcranial magnetic stimulation (TMS) in the treatment of schizophrenia: a review of the literature to date. Innov Clin Neurosci 12(7–8):12–19, 2015 26351619
Cotelli M, Manenti R, Cappa SF, et al: Transcranial magnetic stimulation improves naming in Alzheimer disease patients at different stages of cognitive decline. Eur J Neurol 15(12):1286–1292, 2008 19049544
Davis KD, Moayedi M: Central mechanisms of pain revealed through functional and structural MRI. J Neuroimmune Pharmacol 8(3):518–534, 2013 22825710
de Andrade DC, Mhalla A, Adam F, et al: Neuropharmacological basis of rTMS-induced analgesia: the role of endogenous opioids. Pain 152(2):320–326, 2011 21146300
Del Casale A, Kotzalidis GD, Rapinesi C, et al: Functional neuroimaging in obsessive-compulsive disorder. Neuropsychobiology 64(2):61–85, 2011 21701225
Dinur-Klein L, Dannon P, Hadar A, et al: Smoking cessation induced by deep repetitive transcranial magnetic stimulation of the prefrontal and insular cortices: a prospective, randomized controlled trial. Biol Psychiatry 76(9):742–749, 2014 25038985
Fitzgerald PB, Benitez J, Daskalakis JZ, et al: The treatment of recurring auditory hallucinations in schizophrenia with rTMS. World J Biol Psychiatry 7(2):119–122, 2006 16684685
França NR, Toniolo EF, Franciosi AC, et al: Antinociception induced by motor cortex stimulation: somatotopy of behavioral response and profile of neuronal activation. Behav Brain Res 250:211–221, 2013 23692698
Galhardoni R, Correia GS, Araujo H, et al: Repetitive transcranial magnetic stimulation in chronic pain: a review of the literature. Arch Phys Med Rehabil 96 (4 suppl):S156–S172, 2015 25437106
Grall-Bronnec M, Sauvaget A: The use of repetitive transcranial magnetic stimulation for modulating craving and addictive behaviours: a critical literature review of efficacy, technical and methodological considerations. Neurosci Biobehav Rev 47:592–613, 2014 25454360
Greenberg B, George MS, Martin JD, et al: Effect of prefrontal repetitive transcranial magnetic stimulation in obsessive-compulsive disorder: a preliminary study. Am J Psychiatry 154(6):867–869, 1997 9167520
Guerra A, Assenza F, Bressi F, et al: Transcranial magnetic stimulation studies in Alzheimer’s disease. Int J Alzheimers Dis 2011:263817, 2011 21760985
Haffen E, Chopard G, Pretalli JB, et al: A case report of daily left prefrontal repetitive transcranial magnetic stimulation (rTMS) as an adjunctive treatment for Alzheimer disease. Brain Stimulat 5(3):264–266, 2012 22037125
Hasan M, Whiteley J, Bresnahan R, et al: Somatosensory change and pain relief induced by repetitive transcranial magnetic stimulation in patients with central poststroke pain. Neuromodulation 17(8):731–736, discussion 736, 2014 24934719
Hoffman RE, Boutros NN, Berman RM, et al: Transcranial magnetic stimulation of left temporoparietal cortex in three patients reporting hallucinated “voices.” Biol Psychiatry 46(1):130–132, 1999 10394483
Hoffman RE, Hawkins KA, Gueorguieva R, et al: Transcranial magnetic stimulation of left temporoparietal cortex and medication-resistant auditory hallucinations. Arch Gen Psychiatry 60(1):49–56, 2003 12511172
Johnson S, Summers J, Pridmore S: Changes to somatosensory detection and pain thresholds following high frequency repetitive TMS of the motor cortex in individuals suffering from chronic pain. Pain 123(1–2):187–192, 2006 16616419
Karsen EF, Watts BV, Holtzheimer PE: Review of the effectiveness of transcranial magnetic stimulation for post-traumatic stress disorder. Brain Stimulat 7(2):151–157, 2014 24486424
Khedr EM, Kotb H, Kamel NF, et al: Longlasting antalgic effects of daily sessions of repetitive transcranial magnetic stimulation in central and peripheral neuropathic pain. J Neurol Neurosurg Psychiatry 76(6):833–838, 2005 15897507
Koob GF, Volkow ND: Neurocircuitry of addiction. Neuropsychopharmacology 35(1):217–238, 2010 19710631
Lee JH, Hong YJ, Bae HJ, et al: The effects of galantamine treatment on attention and its relationship with cognition and activities of daily living in patients with mild to moderate Alzheimer’s disease. J Clin Neurol 11(1):66–72, 2015 25628739
Lefaucheur JP, Drouot X, Nguyen JP: Interventional neurophysiology for pain control: duration of pain relief following repetitive transcranial magnetic stimulation of the motor cortex. Neurophysiol Clin 31(4):247–252, 2001 11601430
Liang X, Liu K, Guo L: Repetitive transcranial magnetic stimulation (rTMS): a possible novel therapeutic approach to dementia with Lewy bodies. Med Hypotheses 74(5):877–879, 2010 20006914
Luber B, Lisanby SH: Enhancement of human cognitive performance using transcranial magnetic stimulation (TMS). Neuroimage 85 (Pt 3):961–997, 2014 23770409
Maarrawi J, Peyron R, Mertens P, et al: Motor cortex stimulation for pain control induces changes in the endogenous opioid system. Neurology 69(9):827–834, 2007 17724284
Mahan AL, Ressler KJ: Fear conditioning, synaptic plasticity and the amygdala: implications for posttraumatic stress disorder. Trends Neurosci 35(1):24–35, 2012 21798604
Melzack R, Casey KL: Sensory, Motivational, and Central Control Determinants of Pain. Springfield, IL, Charles C Thomas, 1968
Menzies L, Chamberlain SR, Laird AR, et al: Integrating evidence from neuroimaging and neuropsychological studies of obsessive-compulsive disorder: the orbitofronto-striatal model revisited. Neurosci Biobehav Rev 32(3):525–549, 2008 18061263
Pagano RL, Fonoff ET, Dale CS, et al: Motor cortex stimulation inhibits thalamic sensory neurons and enhances activity of PAG neurons: possible pathways for antinociception. Pain 153(12):2359–2369, 2012 23017297
Pallanti S, Quercioli L: Treatment-refractory obsessive-compulsive disorder: methodological issues, operational definitions and therapeutic lines. Prog Neuropsychopharmacol Biol Psychiatry 30(3):400–412, 2006 16503369
Pennisi G, Ferri R, Cantone M, et al: A review of transcranial magnetic stimulation in vascular dementia. Dement Geriatr Cogn Disord 31(1):71–80, 2011 21242688
Pleger B, Janssen F, Schwenkreis P, et al: Repetitive transcranial magnetic stimulation of the motor cortex attenuates pain perception in complex regional pain syndrome type I. Neurosci Lett 356(2):87–90, 2004 14746870
Rabey JM, Dobronevsky E, Aichenbaum S, et al: Repetitive transcranial magnetic stimulation combined with cognitive training is a safe and effective modality for the treatment of Alzheimer’s disease: a randomized, double-blind study. J Neural Transm (Vienna) 120(5):813–819, 2013 23076723
Rollnik JD, Wüstefeld S, Däuper J, et al: Repetitive transcranial magnetic stimulation for the treatment of chronic pain—a pilot study. Eur Neurol 48(1):6–10, 2002 12138303
Rutherford G, Gole R, Moussavi Z: rTMS as a treatment of Alzheimer’s disease with and without comorbidity of depression: a review. Neurosci J 2013:679389, doi: 10.1155/2013/679389 2013 26317096
Sachdev PS, Loo CK, Mitchell PB, et al: Repetitive transcranial magnetic stimulation for the treatment of obsessive compulsive disorder: a double-blind controlled investigation. Psychol Med 37(11):1645–1649 2007 17655805
Short EB, Borckardt JJ, Anderson BS, et al: Ten sessions of adjunctive left prefrontal rTMS significantly reduces fibromyalgia pain: a randomized, controlled pilot study. Pain 152(11):2477–2484, 2011 21764215
Silbersweig DA, Stern E, Frith C, et al: A functional neuroanatomy of hallucinations in schizophrenia. Nature 378(6553):176–179, 1995 7477318
Slotema CW, Blom JD, Hoek HW, et al: Should we expand the toolbox of psychiatric treatment methods to include repetitive transcranial magnetic stimulation (rTMS)? A meta-analysis of the efficacy of rTMS in psychiatric disorders. J Clin Psychiatry 71(7):873–884, 2010 20361902
Slotema CW, Blom JD, van Lutterveld R, et al: Review of the efficacy of transcranial magnetic stimulation for auditory verbal hallucinations. Biol Psychiatry 76(2):101–110, 2014 24315551
Sokhadze EM, El-Baz A, Baruth J, et al: Effects of low frequency repetitive transcranial magnetic stimulation (rTMS) on gamma frequency oscillations and event-related potentials during processing of illusory figures in autism. J Autism Dev Disord 39(4):619–634, 2009 19030976
Sokhadze E, Baruth J, Tasman A, et al: Low-frequency repetitive transcranial magnetic stimulation (rTMS) affects event-related potential measures of novelty processing in autism. Appl Psychophysiol Biofeedback 35(2):147–161, 2010 19941058
Stanford AD, Corcoran C, Bulow P, et al: High-frequency prefrontal repetitive transcranial magnetic stimulation for the negative symptoms of schizophrenia: a case series. J ECT 27(1):11–17, 2011 20966771
Treede R, Apkarian A: Nociceptive Processing in the Cerebral Cortex. New York, Elsevier, 2009
Uher R, Yoganathan D, Mogg A, et al: Effect of left prefrontal repetitive transcranial magnetic stimulation on food craving. Biol Psychiatry 58(10):840–842, 2005 16084855
Van den Eynde F, Claudino AM, Mogg A, et al: Repetitive transcranial magnetic stimulation reduces cue-induced food craving in bulimic disorders. Biol Psychiatry 67(8):793–795, 2010 20060105
Vogt B, Sikes R: Cingulate Nociceptive Circuitry and Roles in Pain Processing: The Cingulate Premotor Pain Model. New York, Oxford University Press, 2009
Xia MR, Wang JH, HE Y: BrainNet Viewer: a network visualization tool for human brain connectomics. PLoS One 8(7):e68910, 2013 23861951
Zangen A, Carmi L, Alyagon U, et al: Deep transcranial magnetic stimulation of the anterior cingulate cortex in obsessive compulsive disorder patients. Brain Stimul 9(5):e4 2016
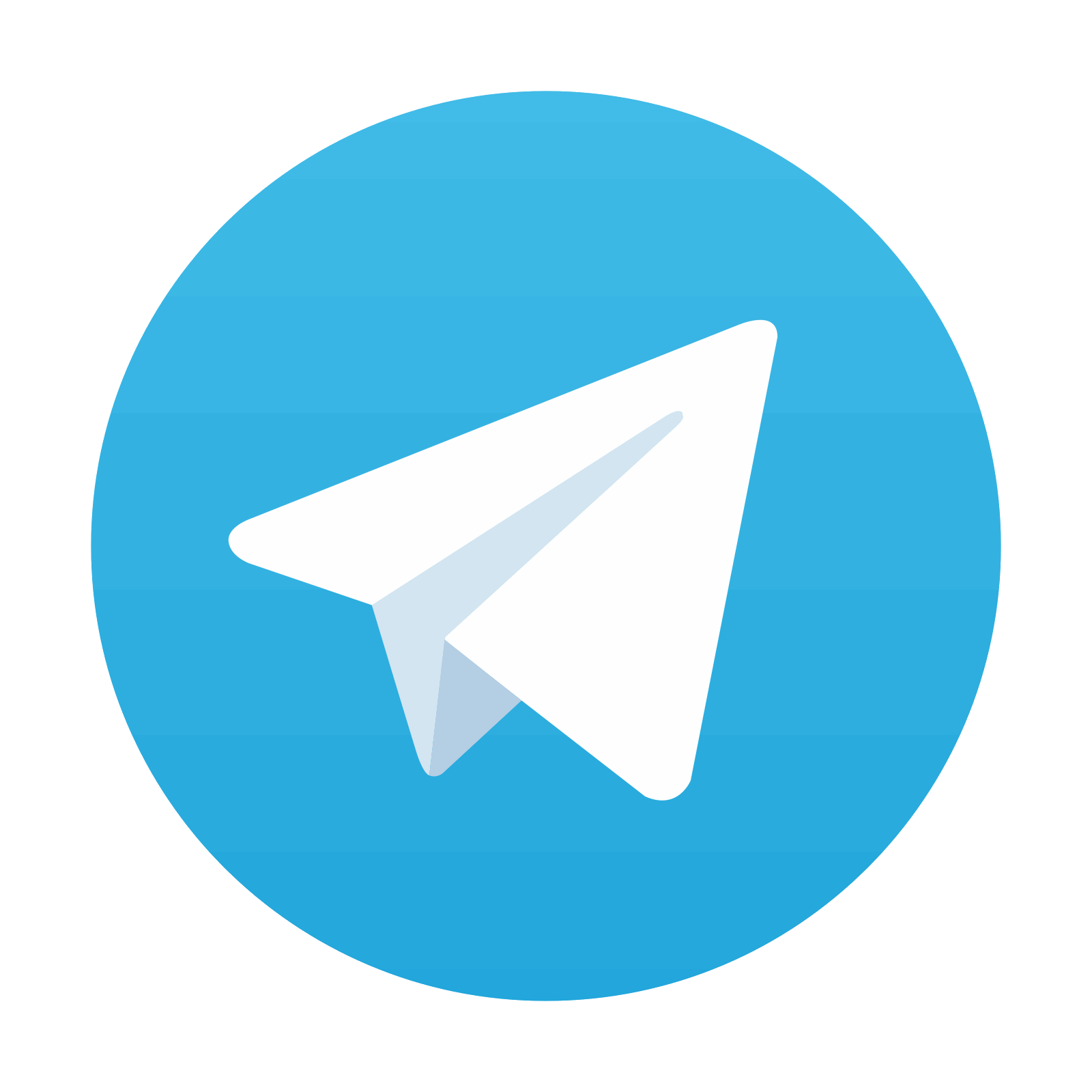
Stay updated, free articles. Join our Telegram channel

Full access? Get Clinical Tree
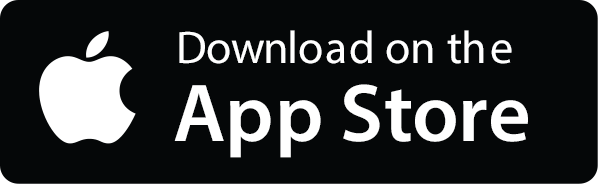
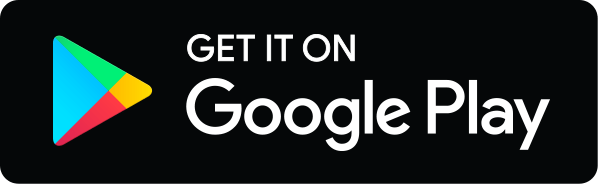
