Airway secure/controlled
Chest, need for needle decompression
Hemorrhage, external, controlled/adjuncts (TXA, blood products)
C- Collar/spine precautions need
Splinting
Monitoring (O2 Sat, ETCO2)
Medications (Sedation, pain, seizure, RSI, and ICP elevation)
Table 14.2
Long-range aeromedical transport checklist
Airway secure |
ETT cuff location/cuff pressure |
Mechanical ventilation concerns (altitude compensation and sufficient oxygen stores) |
Spine protection (collar and vacuum spine board) |
CXR review for pneumothorax, pneumonia, and chest tube placement |
Oral-gastric tube placement (increased risk ileus/aspiration) |
Monitoring (ETCO2, EKG, Pulse Oximetry, and ICP) |
Laboratory review (Lactate, PaCo2, and O2) |
Medication considerations (Antibiotics, seizure prophylaxis, pain meds, sedation meds, hypertonic saline, DDAVP, and oral care) |
ICP elevation treatment algorithm |
Temperature regulation plan |
Blood product need assessment |
Medical records/images obtained |
Fit to fly determination? (Oxygenation, ICP, and infection) |
Positioning concerns (Head first, HOB elevated, and infection control) |
Stressors of Flight
While ground transport by itself can introduce risk, aeromedical evacuation (AE) of the acute neurotrauma patient introduces additional complexity. The air transport environment is physiologically hostile to patients in a number of ways. Environmental stressors include hypobarism, hypoxemia, gravitational and acceleration forces, noise, vibration, and decreased humidity. Depending on the distance to be traveled, the austerity of the transport environment can also have a profound effect on ability to diagnose and treat the deteriorating patient. Each of these environmental stressors can potentially exacerbate TBI and must be ameliorated.
Goodman et al. demonstrated that mice exposed to hypoxic hypobarism 3 h after mild TBI had increased levels of inflammatory cytokine IL-6 and neuron specific enolase. Inflammatory biomarkers were not elevated if the hypoxic hypobarism was delayed until 24 h post injury. The authors concluded that early, but not delayed, exposure of post-TBI patients to high altitude was associated with increased neuroinflammation [25]. Skovira et al. demonstrated in rats that non-hypoxic hypobaric exposure even up to 7 days after TBI was associated with worsened cognitive deficits, hippocampal neuronal loss, and microglial/astrocyte activation in comparison to injured controls (no hypobaria) [26]. In addition, they found that exposure to 100 % FiO2 during hypobaria was associated with further spatial memory deficits. In clinical practice, all patients with TBI-regardless of severity should be monitored for oxyhemoglobin saturation during transport to avoid either hypoxia or hyperoxia. Johannigman et al. have demonstrated that autonomous control of the FiO2 may be most effective, and have demonstrated less significant hypoxia when the computer controls the FiO2 [27]. Limited conclusions can be drawn from the combination of these studies, and additional research is needed to further characterize the optimum timing of AE in relation to neuroinflammation and limiting the ‘second hit’ caused by hypobarism with or without hypoxia. Early transport to reach a more capable intensive care unit (ICU), improved neurotrauma monitoring and neurotrauma intervention may ultimately place the patient at lower risks.
Johannigman et al. provide recommendations for limiting many of the stressors experienced during medical transport [4]. Helicopters and military cargo planes are commonly used for patient transport, and vibration and noise is unavoidable. Vibration can cause significant patient discomfort and so to limit this effect, patients’ beds should be padded and placed in the middle of the air frame away from the fuselage to decrease the impact of vibration. Noise should be limited for both the patient and staff by use of hearing protection. At high altitudes, dry air can lead to dehydration and so excess fluids may be needed and eye lubrication (in addition to removal of contact lenses) and a heat and moisture exchanger (HME) for the ventilator should be used. Temperature regulation can also be challenging as cargo aircraft and helicopters are often below 55 °F, and so warming blankets should be available [4]. AE takeoff and landing are the phases of flight that cause the greatest changes of ICP, due to acceleration and deceleration forces. Acceleration during takeoff produces the more abrupt and dramatic shifts. For this reason, patients should be loaded on the aircraft head first with the head of bed at 30° elevation [4].
The AE environment can also constrain patient monitoring. The noise of the AE environment makes audible alarms difficult if not impossible to hear. Patients are not always able to be loaded directly in the line of sight of a team member. Monitors should be mounted such that critical information is most visible, and a heightened awareness of monitor display is necessary. Improved visual interfaces with equipment and smart monitoring may, in the future, aid in earlier recognition of a deteriorating patient.
Neurocritical Care During Transport
Extensive evidence exists for the importance of avoiding hypoxia in TBI. A prospective observational study by Chi et al. demonstrated prehospital hypoxia in 28 % of TBI patients, with mortality significantly increased in those patients (37 % mortality vs. 20 %, OR 2.66) in the face of similar injury severity scores between the two groups [28]. Of the survivors, the Disability Rating Scale and length of stay (LOS) were significantly higher when secondary insults had occurred (hypoxia and/or hypotension).
Two effective means of reducing hypoxia in a ventilated patient are increasing the FiO2 or the positive-end-expiratory-pressure (PEEP). Traditional thinking was that higher levels of PEEP should be avoided: increasing the PEEP would lead to increased thoracic venous pressure which would in turn decrease venous return resulting in an increase in ICP and a decrease in cerebral perfusion pressure (CPP). Huynh et al. looked retrospectively at this hypothesis in severe head injury patients with ICP monitors and found the opposite to be true: an increase in PEEP was associated with a decrease in ICP and an increase in CPP [29]. Notwithstanding this evidence, Barnes et al. found underutilization of PEEP in long range AE by CCATT in almost 50 % of patients [30]. As PEEP is an effective means of treating pulmonary injury after trauma and given the above data, appropriate use of PEEP is recommended during the transport of critically ill patients.
Historically, mild hyperventilation was used as a means of lowering ICP due to cerebral vasoconstriction. This practice has largely been abandoned except in acute situations of herniation due to a resultant decrease in cerebral blood flow resulting in increased morbidity and mortality [31, 32]. Jeremitsky et al. found 80 % of their severe TBI patients experienced hypocapnia at least once (defined at PCO2 < 30) and this was associated with an increased ICU LOS as well as overall LOS [33]. Davis et al. demonstrated that end-tidal carbon dioxide (ETCO2) monitoring led to a significant reduction (5.6 % vs. 13.4 %) of severe inadvertent hyperventilation (PCO2 < 25) during transport of head injury patients [34]. These studies and others have led to the recommendation for ETCO2 monitoring by capnography during transport [35–38].
Along with hypoxia, the most well-studied ‘second hit’ is hypotension. Jeremitsky et al. reported that 68 % of their severe blunt TBI patients (GCS ≤ 8) experienced a hypotensive episode (MAP < 70) and this was independently associated with increased morbidity (length of stay, discharge to rehab rather than home) and mortality [33]. Chestnut et al. also looked at hypotension in severe head injury patients (GCS ≤ 8) and found a high prevalence (34.6 %) and an associated doubling in mortality (55 % vs. 27 %). Furthermore, the combination of hypotension and hypoxia at admission resulted in a 65 % mortality [39]. From this evidence, regular blood pressure monitoring (every 5 min) and invasive blood pressure monitoring when available are highly recommended prior to and during transport.
There is mounting data regarding the importance of temperature control in TBI patients. While controlled hypothermia in severe TBI was shown earlier in smaller trials to be beneficial, [40, 41] the more recent data favors maintenance of normothermia [33, 42]. Jones et al. found hyperthermia to be one of the three most important predictors of mortality in severe TBI patients (along with hypotension and hypoxemia) [43]. Hyperthermia is thought to be detrimental in several ways. It is associated with ICP elevation [44] and found to be an independent risk factor for posttraumatic vasospasm [45]. As transport environmental temperatures may be difficult to monitor and control, regular monitoring and maintenance of patient normothermia are important to prevent further ischemia, inflammation, edema, and secondary injury. This can usually be adequately accomplished during transport with external warming and cooling techniques; invasive and noninvasive targeted temperature management systems are being used more frequently in neuro ICUs.
Current guidelines for the management of severe brain injury recommend ICP monitoring in all patients with severe TBI (GCS ≤ 8) and evidence of intracranial pathology on CT scan [9, 46]. Further, any patient with severe head injury without CT evidence of intracranial pathology but with any two of the following: age older than 40 years, any hypotensive episodes with Systolic Blood Pressure (SBP) < 90, or abnormal motor posturing (unilateral or bilateral) should have ICP monitoring in place [46]. Hospital monitoring of the neurosurgical patient involves turning off sedation for neurologic checks frequently. This is more challenging in austere transport environments that are hyperstimulating with both noise and vibration which may independently exacerbate ICP. As a result, it is necessary to keep these patients more deeply sedated and minimize neuro checks; thus additional methods for monitoring are often needed. During long transports, ventricular drains may be preferred over intraparenchymal fiberoptic catheters as ventriculostomies allow therapeutic CSF drainage in addition to ICP monitoring. However, ventricular drain collection systems must be handled correctly to avoid inadvertent CSF drainage. Transport teams must be trained with and feel comfortable using and troubleshooting these devices. As an example, occlusion of the air filter (with moisture) has resulted in inadvertent pressurization of the system and increased ICP readings. A prevention strategy includes maintaining the systems upright (do not lay down). Those patients with an ICP intraparenchymal monitor may require calibration and an inflight monitor specific to the manufacturer of the catheter.
Prior to transport, hard copies of all documentation and imaging should be obtained and transported with the patient. This is especially important if the electronic medical record system becomes nonfunctional or the patient is diverted to an unintended location while en route.
Neurocritical Care Standard Bundles
Seizures are a well known complication of TBI. Prior to empiric prophylaxis with anti-epileptics, seizures affected an estimated 5000–30,000 head injury patients a year in the United States. Temkin et al. in 1990 conducted a landmark randomized, double-blinded study of severe TBI patients which assigned to seizure prophylaxis with phenytoin compared to no prophylaxis. Phenytoin significantly decreased the risk of seizure during the first week after injury (3.6 % vs. 14.2 %) [47]. This and other studies have led to the current recommendation of a loading phenytoin dose followed by 7 days of phenytoin prophylaxis. Levetiracetam has essentially replaced phenytoin due to its ease of administration.
Stress ulcer prophylaxis (proton pump inhibitors or histamine-2-receptor antagonists) is used in high-risk patients (i.e., in ventilated or coagulopathic) who are not undergoing enteral feeding [48]. In patients without progression of intracranial pathology on CT or other contraindications to anticoagulation (coagulopathy and active bleeding), enoxaparin (30 mg subcutaneously twice daily) should be started for this high-risk population [49]. In patients without abdominal pathology, nutrition should be started as soon as possible. This has been safely accomplished during 10 h transports by USAF CCATT using an oral gastric tube decompression and placement of a feeding tube distal to the ligament of Treitz to prevent aspiration during transport. Patients receiving enteric nutrition must be monitored closely due to the increased incidence of ileus in TBI as well as the known intestinal gaseous expansion at altitude (Boyle’s Law).
Hyperglycemia has also been shown to exacerbate secondary brain injury and is an independent predictor of outcome [50–53]. In addition, tight glucose control (80–110 mg/dL) is associated with worse outcomes versus loose control (120–150 mg/dL) [54]. From this body of evidence, control of blood sugar (120–150 mg/dL) is recommended and frequent checks during transport should be performed.
Complications and Management Considerations During Transport
Transport of the trauma patient over long distances requires an absolute awareness of the numerous potential neuro and trauma specific clinical complications which may develop. The goal CPP (MAP-ICP) is currently recognized to be > 60 mm Hg [9]. In treating low CPP, the first step should be normalization of blood pressure. In severe TBI, SBP should always be above 90 mm Hg [46] or above 100 mm Hg [9] and with some evidence supporting a goal greater than 110 mm Hg [55] using isotonic to hypertonic fluid resuscitation (to maintain normovolemia) and vasoactive agents when necessary. A treatment algorithm for the management of elevated ICP should be followed, see Table 14.3.
Table 14.3
Increased ICP treatment algorithm (Maintain ICP < 20–25)
1. Head of bed elevation > 30 degrees |
2. Head midline positioning |
3. C- Collar tightness limited |
4. PaCo2 titrated to 35–40 |
5. Treat hypoxia; maintain saturation > 95 % |
6. Treat hypotension, maintain CPP > 60 |
7. Treat potential pain |
8. Sedation; limit external stimuli |
9. Evacuation of CSF if ventriculostomy present |
10. Consider hyperosmolar therapy |
11. Head CT imaging once available |
12. Consider subclinical seizure activity |
In hemorrhagic shock or in severe bleeding associated with a coagulopathy, a more aggressive and balanced approach to blood product resuscitation should be implemented [56]. Targeting a 1:1:1 ratio of PRBC:plasma:platelets decreases hemorrhagic shock mortality [57]. Flight teams increasingly carry blood and blood products to obtain this survival advantage [58].
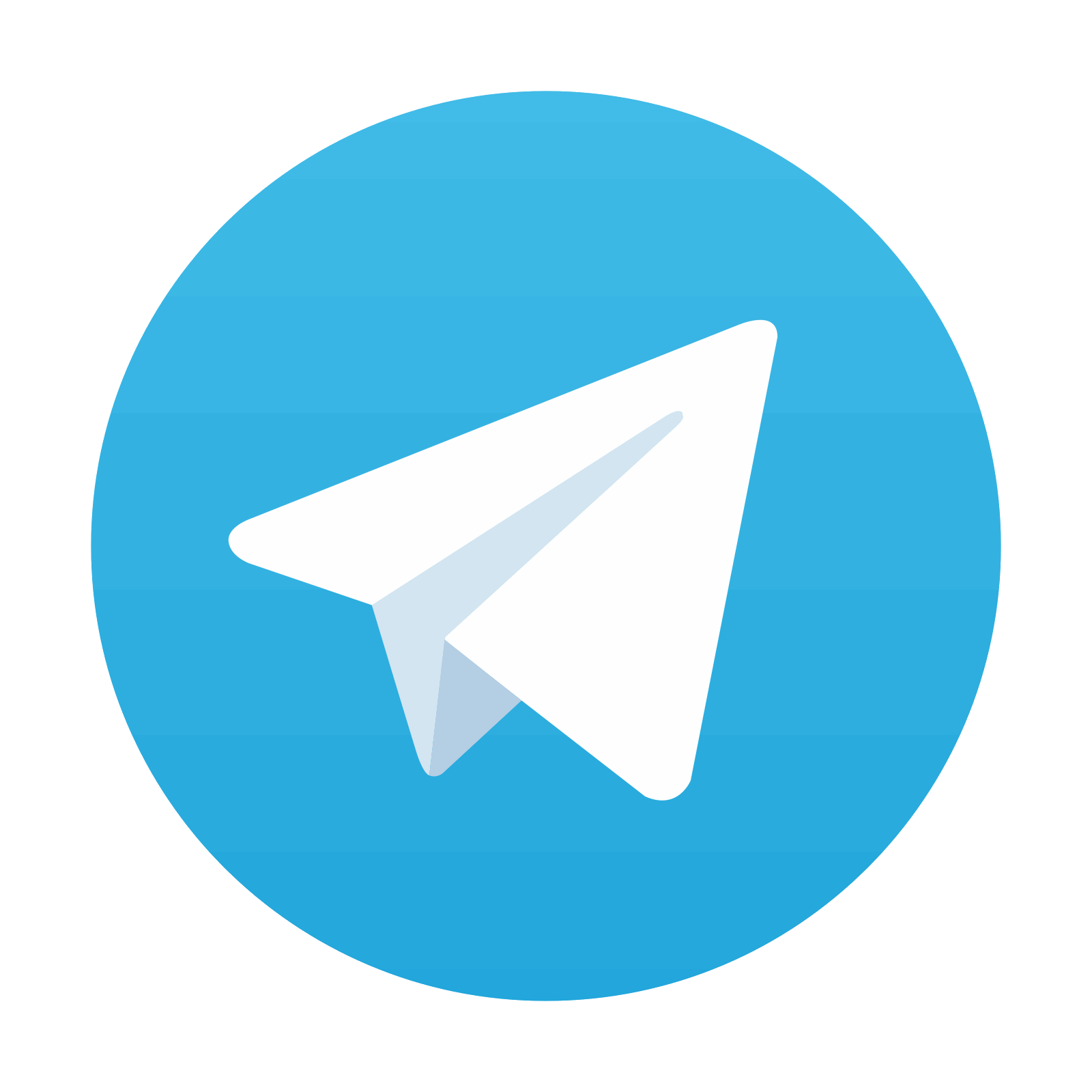
Stay updated, free articles. Join our Telegram channel

Full access? Get Clinical Tree
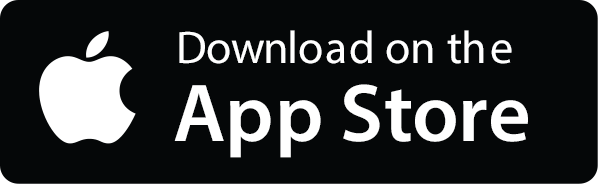
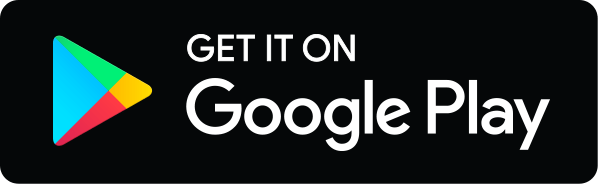