© Springer International Publishing AG 2018
Maria Muzik and Katherine Lisa Rosenblum (eds.)Motherhood in the Face of TraumaIntegrating Psychiatry and Primary Carehttps://doi.org/10.1007/978-3-319-65724-0_66. Trauma Exposure: Consequences to Maternal and Offspring Stress Systems
(1)
Department of Psychology, DePaul University, 2219 N Kenmore Ave., Chicago, 60614, IL, USA
Abstract
Trauma exposure is a common experience for childbearing-age women: among women over age 18 in the USA, one in three has experienced intimate partner violence, and more than 40% has experienced sexual violence during their lifetime (Breiding et al. 2014; Tjaden and Thoennes, 2000). In this chapter, we discuss associations between traumatic experiences and physiological stress system functioning among adults, with particular emphasis on the experiences of mothers and their young children. We focus on abnormalities of two interdependent physiological systems that orchestrate the stress response: the hypothalamic-pituitary-adrenal axis and the autonomic nervous system. Dysfunction in these systems has been implicated in the development of multiple physical and psychological problems (Shonkoff et al. 2009). Importantly, stress system alterations may help explain the negative outcomes observed among children of trauma-exposed women or the intergenerational transmission of trauma. First, stress system dysfunction is likely to permeate functioning across a range of functional domains, including parenting (Leerkes et al. 2015), shaping children’s development. Second, dysregulation of stress responses can directly affect fetal development during gestation, when maternal and fetal biology are intrinsically connected, leading to increased risk for physical and psychological disease (Talge et al. 2007). Stress response alterations may be fruitful targets of interventions that seek to address the needs of trauma-exposed mothers and promote better outcomes for their offspring.
6.1 Trauma and HPA Axis Functioning
The stress response consists of a set of adaptive physiological and behavioral changes, such as increased heart rate and blood pressure, inhibition of vegetative functions, and activation of fight/flight responses that are designed to prepare the organism to face an environmental challenge or threat (Gunnar and Quevedo 2007). The physiological stress response system is comprised of two interdependent systems: the hypothalamic-pituitary-adrenocortical (HPA) axis and the autonomic nervous system (ANS). The HPA axis consists of the hypothalamus, the anterior pituitary, and the adrenal cortex. After receiving information from the amygdala, the hippocampus, and the autonomic nervous system, the HPA axis initiates a stress response, increasing hypothalamic release of corticotropin-releasing hormone (CRH) and arginine vasopressin (AVP). CRH stimulates adrenocorticotropic hormone (ACTH) and B-endorphin (B-E) release from the pituitary gland into the circulatory system and onto the adrenal cortex, where secretion of cortisol is stimulated. Cortisol is released into the body and the brain, inducing stress-associated changes in blood pressure, glucose metabolism, growth, and reproduction, as well as cognition, memory, and emotion; however, chronic exposure to high levels of circulating cortisol has catabolic, lipogenic, antireproductive, and immunosuppressive effects (Gunnar and Quevedo 2007).
Once the stressor has passed, HPA axis activity is downregulated through an inhibitory negative feedback loop. Cortisol binds to hippocampal mineralocorticoid (MR) and glucocorticoid receptors (GR). GR activation is a key to effectively cope with stress, and GR availability is regulated by the NR3C1 gene (Palma-Gudiel et al. 2015). Increased binding exerts an inhibitory effect in production and release of CRH and ACTH, which results in a return to baseline cortisol levels and termination of the HPA stress response (Gunnar and Quevedo 2007). An effective HPA stress response is triggered rapidly in response to internal or external stressful stimuli, inducing increased release of CRH, ACTH, and cortisol, and returns rapidly to the baseline state of homeostasis through the inhibitory effects of hippocampal receptors once the threat has ceased (Herbert et al. 2006). Notably, cortisol levels are influenced by multiple factors (e.g., exercise, eating, etc.), and secretion is influenced by a naturally occurring circadian rhythm of cortisol secretion, characterized by a peak about 30 min after awakening and declining levels throughout the day that reach nadir at nighttime (Herbert et al. 2006). Given the complexity of the stress response system, a number of indicators have been used to reflect HPA axis activity and are thought to reflect different characteristics of HPA functioning.
Early research focused on resting cortisol levels and suggested abnormally low levels among individuals with PTSD (Yehuda 2003), but more recent research reports mixed findings. Two meta-analyses reported no overall differences in basal cortisol levels among individuals with a current diagnosis of PTSD and healthy controls (i.e., no trauma history or no current mental health problems, respectively; Klaassens et al. 2012; Meewisse et al. 2007). However, one of these meta-analysis found that studies that sampled cortisol in the afternoon (n = 7), that included only women (n = 4), that included only individuals with PTSD related to physical or sexual abuse (n = 5), or that excluded trauma-exposed controls (n = 9) did find lower circulating cortisol levels among individuals with PTSD, compared to controls. Similarly, Morris et al. (2012) reported lower resting cortisol levels among trauma-exposed adults with and without PTSD, as compared to non-trauma-exposed adults, but only in the afternoon.
To address the observation that cortisol dysregulation may be most evident at certain times of the day (i.e., afternoon) and capture the characteristic pattern of daily HPA functioning, the cortisol awakening response (CAR) and diurnal patterns of cortisol secretion have been investigated. There are several advantages to using these indices to measure of HPA axis functioning: (1) they require assessing cortisol at multiple data points, enhancing reliability; (2) these indices convey information about output changes throughout the day that reflect the dynamic properties of the system; and (3) they do not require laboratory-based assessment, making sampling more ecologically valid and convenient. Initial studies report a flattened cortisol secretion profile among individuals with PTSD, characterized by decreased CAR and lesser decline in cortisol levels throughout the day (Wessa et al. 2006). In a recent meta-analysis, Chida and Steptoe (2009) found that adults with PTSD displayed lower CAR than the control group in two studies identified as having high methodological quality, although this result was not maintained when studies of variable methodological quality were included (n = 5).
While studies of naturally occurring cortisol levels provide information about baseline HPA axis functioning, challenged cortisol levels have also been examined to better understand how trauma exposure alters the reactivity of this system. Studies using laboratory procedures to elicit psychosocial stress to mobilize the cortisol response have reported mixed findings: two small studies reported cortisol elevations in anticipation to, during, and after a stressful task among individuals with PTSD (i.e., arithmetic task or traumatic script; Bremner et al. 2003; Elzinga et al. 2003), but another study reported no differences between individuals with combat-related trauma and trauma-unexposed controls (Liberzon et al. 1999). On the other hand, studies that use a biological challenge to mobilize the cortisol response report generally consistent findings. The adapted dexamethasone suppression test (DST) measures the sensitivity of the negative feedback loop to terminate the HPA response. Trauma exposure is associated with greater suppression of cortisol in response to DST compared to controls, and this alteration is likely a consequence of traumatization and not only observable after the development of PTSD symptoms: Klaassens et al.’s (2012) meta-analysis of six studies found that trauma-exposed adults showed augmented cortisol suppression after DST compared to controls, while studies that compared trauma-exposed adults with and without PTSD (n = 9) did not find significant differences post-DST.
Interpreting the associations between cortisol levels and trauma exposure is complicated because typically studies confound trauma exposure and PTSD symptoms. However, two of the meta-analyses did find significant HPA axis alterations among trauma-exposed non-PTSD adults, including evening basal cortisol and increased suppression in response to the DST biological challenge, as compared to non-traumatized controls (Klaassens et al. 2012; Morris et al. 2012). A second challenge in this field is the frequent co-occurrence of depression and PTSD among trauma-exposed adults, which has often not been taken into account by studies that focus exclusively on PTSD. Some research suggests that trauma may lead to both hyper- and hypocortisolism and specific patterns of dysregulation may be correlated with different symptom presentations (depression and PTSD, respectively). Heim et al. (2000) reported that depressed women with a history of childhood abuse had an increased cortisol response to a psychosocial stressor, as compared to trauma-exposed non-depressed women and healthy controls, and Morris et al. (2012) found higher evening cortisol among trauma-exposed adults with depression and PTSD and lower levels among trauma-exposed individuals with or without PTSD, as compared to non-traumatized controls. Characterization of psychological symptom profiles needs to be integrated into research to better understand the effects of traumatic experiences on HPA axis functioning.
A second challenge for the field is that much of the early research assessed men with PTSD secondary to military trauma, and those findings may not apply to victims of other traumatic stressors, including young mothers. Addressing this gap, a body of research has focused on victims of interpersonal violence, a highly common and impactful experience for women of childbearing age. A number of studies have reported neuroendocrine dysregulation among victims of intimate partner violence (IPV). Women exposed to IPV display lower levels of cortisol during the morning as compared to nonexposed women (Griffin et al. 2005) and higher levels of cortisol during the evening (Pico-Alfonso et al. 2004), reflecting a flattened profile of diurnal cortisol secretion. In addition, IPV victims display inefficient termination of the stress response (i.e., slower return to baseline after stress; Griffin et al. 2005), even when controlling statistically for the victims’ depression levels. Similarly, a history of childhood maltreatment is associated with lower morning cortisol levels and a blunted cortisol response to psychosocial stressors during adulthood, even when controlling for symptoms of psychological problems (Carpenter et al. 2011).
Overall, research suggests that trauma and consequent PTSD are associated with low resting cortisol levels (Klaassens et al. 2012; Morris et al. 2012), diminished CAR (Chida and Steptoe 2009), a flattened profile of secretion during the day (Wessa et al. 2006), and increased cortisol suppression in response to the DST (Klaassens et al. 2012), a biological challenge to the HPA axis. Findings also highlight the effects of trauma exposure for mothers, as women’s basal cortisol levels are more likely impacted by traumatic exposures (as compared to men’s; Klaassens et al. 2012), and women are also more at risk for physical or sexual abuse, traumatic events that are reliably associated with cortisol dysregulation (Griffin et al. 2005; Carpenter et al. 2011), even when statistically controlling for mental health problems. A hypoactive pattern of HPA activity reflects heightened sensitivity of the negative feedback loop, leading to excessive shutting down of this system (Yehuda 2003) and significant consequences for an individual’s ability to mount an appropriate behavioral response in the face of a stressor, as well as to recover in the aftermath of a stressful event (Morris et al. 2012). Also, reduced cortisol levels may impact cognitive and immune system functioning, leading to increased vulnerability for a variety of different disorders (Heim et al. 2000).
6.2 Trauma and the Autonomic Nervous System
Less research has evaluated a second system that is key for the physiological stress response: the autonomic nervous system (ANS). In response to stressors, the ANS rapidly mounts a biobehavioral response that prepares the organism to face the threat, including the release of catecholamines into the bloodstream, accelerations of heart and respiratory rates, elevations in blood pressure, increased sweat production, and other physiological changes (Gunnar and Quevedo 2007). The ANS comprises a sympathetic branch (SNS), in charge of initiating physiological arousal, and a parasympathetic branch (PNS), which modulates arousal and is responsible for recovery and restoring autonomic homeostasis (Gunnar and Quevedo 2007). Due to its diffuse effects throughout the body, ANS can be measured using a variety of indicators, including heart rate (HR), heart rate variability (HRV), skin conductance levels (SCL), and respiratory sinus arrhythmia (RSA), among others. These different physiological markers are thought to represent different ANS subsystems: (1) the social communication system, which includes the myelinated vagus nerve and the PNS and influences heart rate and respiratory sinus arrhythmia (RSA); (2) the mobilization system, which comprises the SNS and flight-or-fight behaviors and leads to increases in heart rate and skin conductance levels (SCL); and (3) the primitive immobilization system, which includes the unmyelinated vagus nerve and can be expressed via freezing behaviors (Porges and Furman 2011).
Early studies mostly focused on the SNS and documented elevated cardiovascular activity among individuals with PTSD as compared to traumatized healthy adults. For example, two meta-analyses have reported increased resting HR among individuals with PTSD as compared to those without PTSD (Buckley and Kaloupek 2001; Pole 2007). Although most of the studies have evaluated male military veterans, some research documents similar heart rate elevations, as measured when trauma reminders were presented, among victims of motor vehicle accidents (Blanchard et al. 1996) and adult victims of childhood sexual abuse (Orr et al. 1998). However, measures of heart rate can reflect both sympathetic and parasympathetic activity, making it hard to tease apart the specific dysfunction that is associated with PTSD. In contrast, SCL reflect the activity of the SNS and are not affected by PNS activation. When the SNS is activated, sweat accumulates in the sweat glands and sweat levels in the ducts of the palm surface increase (Dawson et al. 2011). A number of studies have reported higher SCL in response to laboratory stressors among individuals with PTSD secondary to war, terrorism, accidents, and sexual assault (Shalev et al. 1997; Orr et al. 1997), providing evidence of specific stress-induced SNS hyperactivity.
Fluctuations in heart rate can be used to better understand the efficiency and coordination between the SNS and PNS: in low-stress situations, heart rate is slowed, but when a threat is detected, the vagal response of the parasympathetic system is diminished, and the sympathetic nervous system induces increases in heart rate. Heart rate variability (HRV) reflects these changes over time, with more vagal control promoting more rapid adjustments to environmental inputs (high HRV). Low HRV represents higher sympathetic and lower parasympathetic tone and is associated with less optimal emotion regulation in response to stressful events and poor psychological outcomes (i.e., trauma exposure, depression, anxiety; Liddell et al. 2016). Research has documented lower resting HRV among adults with PTSD or other stress-related psychopathology (i.e., depression or intermittent explosive disorder), as compared to healthy controls (Cohen et al. 2000; Liddell et al. 2016). At rest, a high HRV is generally more favorable, and low resting HRV reflects decreased parasympathetic activity and increased sympathetic activity that is consistent with basal autonomic hyperactivation and arousal. Only one study has evaluated HRV during a stressful challenge (as opposed to resting conditions). Cohen et al. (2000) found that individuals with PTSD did not show the expected increased autonomic activity in response to trauma reminders but controls and individuals with panic disorder did. However, this study had relatively small groups of individuals with PTSD (n = 14 and 36), and results need further replication.
A third index, respiratory sinus arrhythmia (RSA), is used as a measure of PNS activity. The amplitude of RSA indicates the change in heart rate that occurs during breathing cycles: during inspiration HR increases and during expiration HR decreases (Porges and Furman 2011). Because parasympathetic activity affects heart rate very rapidly, changes in the high-frequency range of heart rate variability (0.15–0.50 Hz) are used to index RSA. Efficient decreases in vagal activity to adapt to environmental demands, or RSA withdrawal, is thought to reflect emotional and behavioral control (Porges and Furman 2011). Decreases in RSA in response to trauma reminders are characteristic of PTSD (Sack et al. 2004). Cyranowski et al. (2011) found that depressed women with high levels of lifetime trauma exposure had the lowest levels of RSA during a stressful laboratory task regardless of their PTSD status, as compared to non-depressed and depressed women with low traumatic exposures, suggesting diminished cardiac vagal control.
Again, it is difficult to disentangle the effects of trauma from biological correlates of PTSD because most of the studies reviewed compared individuals with PTSD to controls, but studies that compare trauma-exposed adults without PTSD to healthy controls are rare. In addition, a lot of this literature addresses PTSD-related deficits among adults who experienced military trauma, and their findings may not represent the ANS alterations of victims of other traumatic stressors. To address this deficit, a few studies have examined the effects of interpersonal violence on ANS functioning and provide preliminary evidence of significant alterations among trauma-exposed adults, which at least in part mirror the findings of the PTSD studies. Self-reported childhood maltreatment history is associated with high stress-induced SCL and low resting RSA during adulthood (Dale et al. 2009). Research also documents lower HRV when victims of partner violence diagnosed with PTSD are exposed to trauma reminders (Hauschildt et al. 2011).
In sum, PTSD is associated with ANS alterations that include increased SNS activity during resting and stressful or trauma-related conditions, higher sympathetic and lower parasympathetic tone during rest (Shalev et al. 1997; Orr et al. 1997), and less vagal (parasympathetic) control during stressful tasks (Cohen et al. 2000; Liddell et al. 2016; Sack et al. 2004). Some of the alterations (e.g., HR elevations during rest and lower HRV at rest and in response to trauma reminders) are likely biological correlates of PTSD, but others seem to be directly linked to trauma exposure: for example, lower RSA is characteristic of depressed women with high lifetime trauma exposure, regardless of their PTSD status (Cyranowski et al. 2011), and higher SCL and lower RSA are found among victims of childhood maltreatment and IPV as compared to women not exposed to interpersonal trauma (Dale et al. 2009). Heightened arousal paired with low parasympathetic control has significant functional implications, including poor health outcomes (i.e., cardiovascular disease, hypertension, diabetes, and obesity) and psychosocial problems (Liddell et al. 2016).
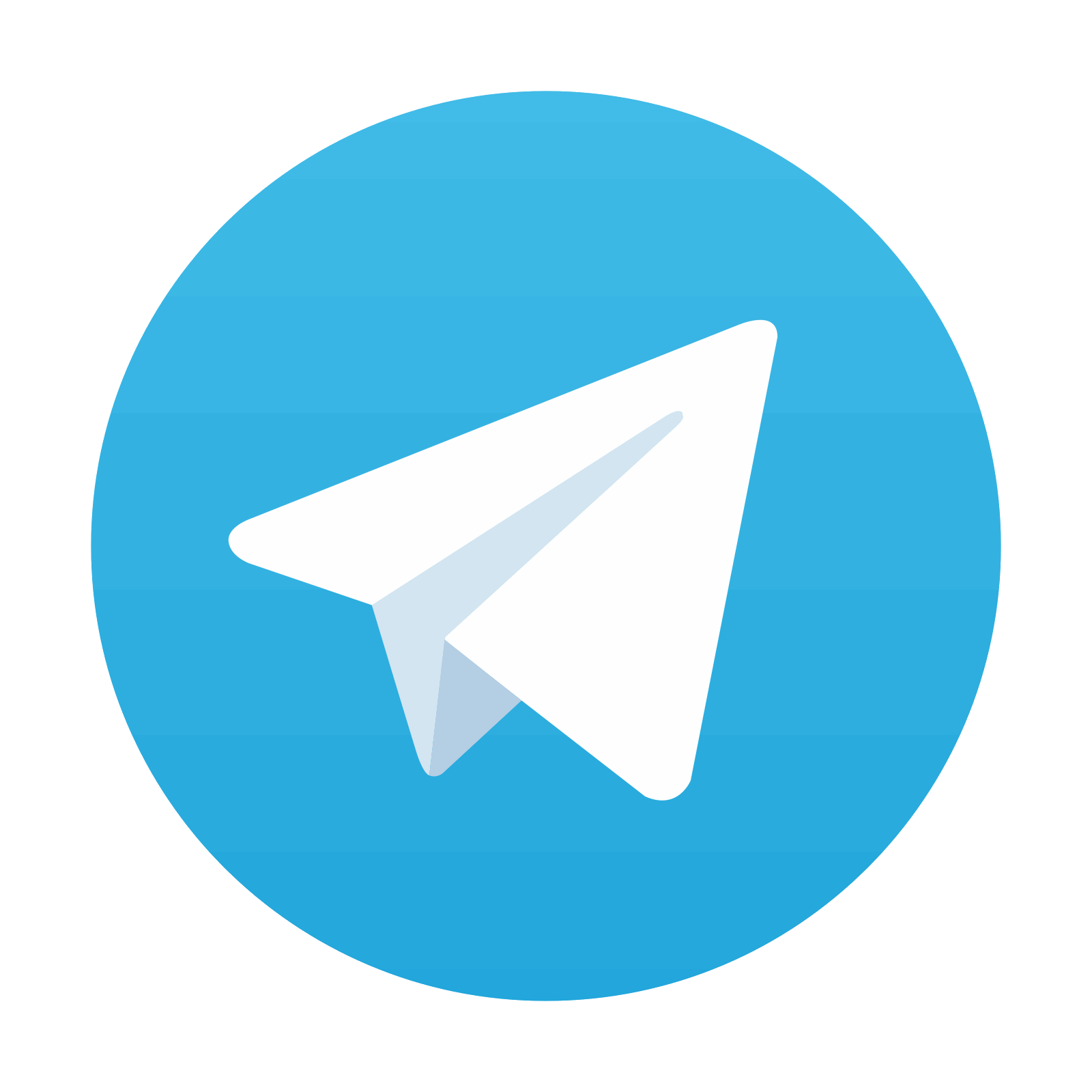
Stay updated, free articles. Join our Telegram channel

Full access? Get Clinical Tree
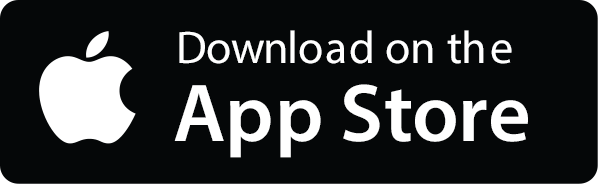
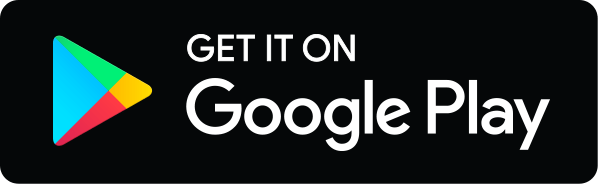