Introduction
Traumatic brain injury (TBI) is a major cause of death and disability in the United States. Each year approximately 2.5 million people are evaluated in a hospital emergency room for a TBI. In 2019, 223,135 individuals were hospitalized indicating that they had suffered a moderate or severe TBI. There were 64,362 TBI-related deaths in 2020. Falls are one of the main causes of TBI-related hospitalizations, especially among older adults. The highest rates and numbers of TBI-related hospitalizations and deaths occur in patients 75 and older. Children aged 0 to 4, adolescents aged 15 to 19, and adults 65 and older are most likely to sustain a TBI. Falls are the leading cause among children, and motor vehicle accidents for adolescents and persons aged 15 to 44. Motor vehicle accidents are the principal cause of TBI-related death. From 2010 to 2016 there were on average 283,000 TBI-related emergency room visits each year in persons under the age of 17 for sports and recreation-related TBIs and approximately 45% of the injuries were due to contact sports. The direct and indirect lifetime cost of TBIs is estimated at $76.5 billion/year and 90% is attributed to the moderate and severe TBIs that require hospitalization.
In this chapter we will review moderate and severe TBI, but the emphasis will be placed on concussion, including sports concussion, as concussion is a common problem encountered in primary care practice.
MODERATE AND SEVERE TRAUMATIC BRAIN INJURY
Definition
When a patient suffers a head injury the initial concern is whether the traumatic insult has resulted in a severe traumatic brain injury (TBI). If, based on the clinical presentation of the injured person, a severe TBI is suspected then the patient should be immediately transported to the hospital emergency room. Indications for performing imaging of the brain using the New Orleans and Canadian criteria are listed in Box 6.1 .
-
Abnormal neurologic exam including testing of gait
-
Progressive headache
-
Recurrent vomiting >2 episodes
-
loss of consciousness
-
Anterograde amnesia longer than 30 minutes
-
Age >60
-
Seizure
-
GCS score <15 2 hours post injury
-
Skull fracture
-
Signs of basilar skull fracture including hemotympanum, raccoon eyes, Battle’s sign with ecchymosis around the mastoid, CSF rhinorrhea or otorrhea
-
Alcohol or drug intoxication
-
Coagulopathy
-
Dangerous mechanism including fall from >5 feet, ejection from a motor vehicle, pedestrian struck by a vehicle
-
Severe injuries include cerebral contusion, subarachnoid hemorrhage, subdural and epidural hemorrhage, intraparenchymal hemorrhage, cerebral edema, skull fracture, and diffuse axonal injury. These injuries may result in death or major disability and must be diagnosed and treated without delay. While brain magnetic resonance imaging (MRI) will detect more intraparenchymal lesions it may miss skull fractures and a computed tomography (CT) scan is the modality of choice. CT can be performed expeditiously and will accurately detect acute structural abnormalities. The Glasgow Coma Scale (GCS) ( Table 6.1 ) provides diagnostic guidance in the acute setting with scoring using eye, motor, and verbal scales. Moderate TBIs have a GCS score of 9 to 12 and severe, 8 or less. Moderate TBIs, as defined by the World Health Organization (WHO) and the Center for Disease Control (CDC) classifications, have abnormal or normal structural imaging, loss of consciousness of 30 minutes to 24 hours, altered mental state of >24 hours and posttraumatic amnesia >1 day and <7 days. Severe TBIs usually have abnormal structural imaging but occasionally imaging will be normal. Loss of consciousness and alteration of mental status are >24 hours, and posttraumatic amnesia is >7 days.
Best eye response (E) | Spontaneous—open with blinking at baseline | 4 |
Opens to verbal command, speech, or shout | 3 | |
Opens to pain, not applied to face | 2 | |
None | 1 | |
Best verbal response (V) | Oriented | 5 |
Confused conversation, but able to answer questions | 4 | |
Inappropriate responses, words discernible | 3 | |
Incomprehensible speech | 2 | |
None | 1 | |
Best motor response (M) | Obeys commands for movement | 6 |
Purposeful movement to painful stimulus | 5 | |
Withdraws from pain | 4 | |
Abnormal (spastic) flexion, decorticate posture | 3 | |
Extensor (rigid) response, decerebrate posture | 2 | |
None | 1 |
Pathogenesis
Both moderate and severe TBIs are serious injuries and require management in a specialized neurocritical care unit. Patients have often suffered multiple bodily injuries requiring multidisciplinary treatment. The mechanism of TBI, including closed head injury, penetrating head injury, blast injury, or crash injury, may provide valuable information regarding treatment and prognosis. The traumatic insult causes an injury to the skull or intracranial structures through direct, rotational, and shearing forces leading to focal contusions, intracranial hemorrhage, subarachnoid hemorrhage, cerebral edema, skull fracture, or diffuse axonal injury. Secondarily, damage occurs from oxidative stress, free radical formation, apoptosis, calcium-related axonal injury, and inflammation. Increased intracranial pressure, cerebral hypoxemia, and edema potentiate the damage.
A traumatic subarachnoid hemorrhage occurs when blood flows into the subarachnoid space from a tear of small capillaries. Unlike a subarachnoid aneurysmal bleed, the blood enters the space at low pressure ( Fig. 6.1 ). An intraventricular hemorrhage may occur leading to hydrocephalus.

Contusions are the result of a direct injury to the brain and are described as a coup injury at the site of impact and contrecoup on the contralateral side ( Fig. 6.2 ). Clinical signs and symptoms can be quite variable ranging from mild to severe.

An epidural hematoma ( Fig. 6.3 ) is usually the result of direct trauma to the temporal region causing disruption of the middle meningeal artery and at times a skull fracture. The bleeding is outside of the dura and characteristically does not cross suture lines. The injury usually results in loss of consciousness followed by a “lucid” period where the patient is essentially asymptomatic, followed by a rapid decline due to increased intracranial pressure. Herniation leading to death may occur.

Subdural hematomas ( Fig. 6.4 ) are the result of direct trauma causing a tear of a bridging vein and can cross suture lines. The acute form is usually associated with a significant brain injury due to mass effect on the underlying brain and cerebral edema and is dependent on the size of the hematoma. Subacute, chronic, and mixed forms of subdural hematomas may occur. A chronic subdural hematoma usually occurs in patients over the age of 60 who have brain atrophy. The most common initial complaint is headache, but the patient may then slowly develop cognitive difficulty and a hemiparesis and occasionally seizures. Small subdural hematomas may have very few symptoms.

Traumatic intraparenchymal hemorrhage ( Fig. 6.5 ), due to vascular injury and disruption, portends a poor prognosis. Hematomas with associated brain edema tend to expand over time and a volume of more than 50 mL is associated with a very high mortality. Coagulopathies contribute to hematoma formation and expansion.

Vascular stretching may lead to arterial dissection with disruption in the intimal layer resulting in an intramural hematoma that can cause stenosis, limiting blood flow and increasing the risks of embolic infarcts. The cervical internal carotid artery just below the skull base is most often affected.
Diffuse axonal injury is the result of significant rotational acceleration-deceleration forces causing extensive axonal shearing. Hemorrhagic foci are seen in the thalamus, brainstem, internal capsule, corpus callosum, and corona radiata. The diffuse injury is associated with disorders of consciousness, and prognosis for recovery is poor. MRI scan is more sensitive than CT in detecting diffuse axonal injury ( Fig. 6.6 ).

Acute Management
Patients who have suffered a moderate to severe acute TBI may require intubation and mechanical ventilation to protect airways. Cardiac arrest may occur. A head CT must be performed as soon as possible to define the intracranial pathology and neurosurgery should be consulted. The cervical spine must be immobilized, and a cervical CT performed to evaluate for fracture or subluxation. The occurrence of early posttraumatic seizures significantly increases the risk of developing posttraumatic epilepsy and to reduce the risk all patients with moderate to severe TBIs should receive an antiepileptic medication for 7 days. There are no data to support treatment for a longer period. Expedited surgical intervention is warranted for epidural and subdural hematomas when there is significant mass effect, and for intraparenchymal hematomas and contusions with a large volume of blood.
Medical interventions to lower intracranial pressure (ICP) should be instituted. Elevation of the head of the bed and hyperosmolar fluids, such as mannitol or hypertonic saline, may be used acutely to reduce intracranial pressure. The use of prophylactic hyperventilation is not recommended as it will cause vasoconstriction and decrease in cerebral blood flow. Studies evaluating therapeutic hypothermia to decrease oxidative stress have revealed mixed results as it effectively reduces intracranial pressure but does not improve outcomes. Glucocorticoids are not recommended and a large study comparing the use of steroids within 8 hours of presentation to placebo revealed that mortality was higher in the group that received the steroid. Patients may be placed in a medically induced coma to reduce the metabolic demand in the brain. Prophylactic use of phenobarbital is not recommended as it has not been shown to influence functional outcome or mortality and will induce systemic hypotension and lead to a more prolonged stay in the intensive care unit. There are some data that support the use of the antifibrinolytic agent, tranexamic acid, in reducing mortality and death when administered within 3 hours after the severe traumatic brain injury but a systematic review and meta-analysis on the efficacy and safety of the drug found no statistically significant difference between patients treated with tranexamic and placebo for mortality, long-term outcome, or risk of adverse events.
An intracranial pressure monitoring device may be placed after the patient is stabilized to reduce early mortality as both cytotoxic and vasogenic edema causing cerebral hypoperfusion may result in death. The intracranial compartment comprises brain parenchyma (83%), cerebrospinal fluid (CSF) (11%), and arterial and venous blood (6%). The structures create a transcortical pressure gradient referred to as intracranial pressure. The homeostatic environment is dependent on each of these contents. An increase in intracranial volume in the traumatized brain due to blood, edema, and venous congestion may result in pathological compression of the brain. ICP may be effectively lowered by CSF drainage. Intracranial monitoring for ICP is indicated if the head CT is abnormal and the GCS score after resuscitation is between 3 and 8. If the CT is normal and the GCS score is 3 to 8 it is still indicated with two of the following: the systolic blood pressure is less than 90, motor posturing is observed, and the patient is over 40 years of age. CSF drainage using the external ventricular drain will effectively reduce pressure but carries the risks of infection and hemorrhage. Refractory elevation of the intracranial pressure may require a decompressive craniectomy.
Immediate and Delayed Complications
There are several complications that may occur in the acute setting including a coagulopathy, most significantly in patients who are on an anticoagulant. Immediate reversal must be administered with either fresh frozen plasma or prothrombin complex concentrates. No mortality benefit has been shown with the use of platelet transfusions for patients on antiplatelet therapy.
Thrombosis of venous sinuses along fracture lines is a common complication and increases the risk of in-hospital mortality. Treatment is difficult as full anticoagulation is contraindicated and there are no data to support the use of antiplatelet therapy or venous thrombosis prophylaxis. Decompressive craniectomy can be considered if there is rapid clinical deterioration.
Cerebral vasospasm is common following a traumatic subarachnoid hemorrhage resulting in cerebral hypoperfusion and ischemia. The vasospasm begins in the first three days and usually persists for 5 to 10 days. Transcranial doppler may detect vasospasm before the onset of ischemic complications and if suspected then a CT or MR angiogram should be performed. Digital subtraction angiography, however, remains the gold standard. The 1,4-dihydropyridine calcium channel blocker, nimodipine, administered orally or intravenously is the treatment of choice.
In the weeks after the patient has regained consciousness neurobehavioral symptoms may be observed including delusions, agitation, impulsiveness, aggression, and cognitive disturbance. Treatment with propranolol, clonidine, and sertraline has been shown to be efficacious. Amantadine, an N -methyl- d -aspartate receptor antagonist which may protect neurons against glutamate excitotoxicity, has been demonstrated to accelerate functional recovery, improve cognition and reduce aggression. Disruption of the sleep-wake cycle is a common sequela of TBI. Insomnia or hypersomnia may occur due to involvement of the reticular activating system, posterior hypothalamus, and cortical projections. Insomnia is treated with cognitive behavioral therapy and benzodiazepines. Hypersomnia responds to stimulants such as modafinil and methylphenidate.
Approximately 10% of patients who sustain a head injury will suffer a seizure during the first week with 50% occurring in the first 24 hours. The incidence is higher in children and correlates with the severity of the injury. Seizures during the first week are referred to as early seizures. Late seizures are those that occur after 1 week and as the risk of recurrence following a late seizure is 70% one episode is sufficient to make a diagnosis of posttraumatic epilepsy. Eighty percent of initial late seizures occur within 2 years of the injury. Ten percent of patients who are hospitalized with a head injury will suffer a seizure. The cumulative incidence of posttraumatic epilepsy (PTE) in patients who have suffered a severe TBI is 25% at 5 years and 32% at 15 years. Three percent of patients who do not suffer an early seizure will develop PTE, however PTE will develop in 25% who experienced an early seizure. Patients who have suffered a concussion may exhibit convulsive activity characterized by tonic posturing followed by myoclonic jerks of the extremities. These episodes, however, may not be epileptic but rather similar to what is observed in convulsive syncope. The incidence of epilepsy is not increased in patients who have suffered a concussion. Risk factors that increase the risk of posttraumatic epilepsy include subdural hematoma, brain contusion, penetrating head injury, depressed skull fracture, focal neurologic deficits, early posttraumatic seizures, premorbid alcohol use disorder, and severity of the injury. Eighty percent of posttraumatic seizures are generalized and 20% are focal. Treatment is with antiepileptic medication.
Clinical deterioration with ataxia, worsening cognitive function, urinary incontinence, lethargy, and lack of progress with rehabilitation may be observed in patients who develop posttraumatic communicating hydrocephalus. Placement of a ventriculoperitoneal shunt is required.
Neuroendocrine disorders occur commonly in patients who have suffered a severe TBI. Problems include diabetes insipidus, syndrome of inappropriate adrenocorticotropic hormone (SIADH), human growth hormone, and adrenocorticotropic hormone deficiencies. The disorders may impede emerging consciousness and should be promptly treated. Patients who suffer a severe TBI have increased caloric needs and there is evidence to suggest that early enteral feeding can decrease neuroendocrine complications.
Spasticity with impaired motor function may occur with moderate to severe TBI and may be painful and interfere with rehabilitation. Management includes physical therapy, medication including baclofen or tizanidine and Onabotulinum Toxin A injections.
Paroxysms of sympathetic hyperactivity, usually beginning 1 to 2 weeks after the TBI, may occur with symptoms including hypertension, diaphoresis, tachycardia, tachypnea, and hyperthermia. Pharmacologic treatment may include propranolol, clonidine, and bromocriptine.
Posttraumatic agitation and neuropsychiatric disorders including depression, anxiety, psychosis, aggression, and posttraumatic stress disorder should be managed with the assistance of Psychiatry. Some patients will exhibit a pseudobulbar affect with inappropriate outbursts of laughing or crying which may respond to a combination of dextromethorphan and quinidine sulfate.
Long-term rehabilitation of patients who have suffered a severe TBI consists of a multimodal interdisciplinary approach with intensive care provided by physical, occupational, and speech therapy, Psychiatry and Rehabilitation Medicine. A brain MRI is helpful in defining the extent of brain damage. Behavioral and environmental strategies are extremely important. Patients who receive intensive inpatient rehabilitation demonstrate earlier gains in independence.
CONCUSSION
Concussion has been recognized as a clinical entity for more than 1000 years. Throughout the 20th century it was studied extensively in boxers but did not pique the interest of the general population, as it is the ultimate goal of a boxer to inflict a concussion on their opponent. In 2005 Dr. Bennet Omalu, a neuropathologist, published a report of a postmortem examination which he had performed on a former football player. Mike Webster played the position of center for the Pittsburgh Steelers and competed in 245 games from 1974 until his retirement in 1991. He died from a myocardial infarction in 2002 at the age of 50 and his family reported that he had exhibited behavioral issues and cognitive difficulty in the 10 years prior to his death. The autopsy revealed amyloid plaques, neurofibrillary tangles, and tau deposits in the neocortical areas interpreted as consistent with chronic traumatic encephalopathy. The documented presence of a neurodegenerative disorder, presumably due to multiple concussions sustained over a long career, raised the level of appreciation of the gravity of the cumulative effect of repetitive mild head injuries that had previously been minimized and often been described as “getting your bell rung.” Since that time concussion has been a frequent topic of conversation and has become a major focus of sports programs in communities and schools at all levels. All 50 states and the District of Columbia, and the NCAA have enacted laws and rules to protect the athlete. The National Football League has made significant changes to prevent the occurrence of concussion, immediately recognize athletes who may have suffered a concussion, and properly treat and manage the injured player. It is the responsibility of medical practitioners who care for patients who have suffered a concussion to have a thorough understanding of the disorder, and know how to recognize, manage, and treat the postconcussion symptoms. Additionally, if the injured patient is an athlete, determine when and if they may resume participation in their sport.
It has been estimated that up to 3.8 million sports-related concussions occur in athletes annually, but the exact number is unknown. Despite the NCAA mandatory concussion education program for student athletes, an anonymous survey of a cohort of college athletes from the University of Pennsylvania revealed that 43% had deliberately concealed their symptoms. In another survey of NCAA women’s ice hockey athletes, 34.2% of players reported concussion-like symptoms after a head impact and 82.8% continued to play. An astounding 66.8% of the players did not disclose their symptoms. The studies give us an indication of the extent to which underreporting may take place. Given the magnitude of the problem, which has reached epidemic proportions, and the possibility of long-term neurologic sequelae, it is essential that we are able to diagnose concussion, provide effective treatment, and prevent recurrent episodes.
DEFINITION OF CONCUSSION
The term “concussion” comes from Latin concussus which means “to shake violently.” While the first recorded description of concussion has been attributed to Hippocrates approximately 2400 years ago, it was not until the 10th century AD that the great Persian physician, Rhazes, made the distinction between concussion as an abnormal physiologic state as opposed to a brain injury. A European physician, Lanfrancus, in the 13th century described a transient paralysis of cerebral function secondary to head trauma from which patients would completely recover and referred to it as “commotio cerebri” in contrast to “contusio cerebri” which implied structural brain damage.
Concussion is often used interchangeably with mild traumatic brain injury (mTBI); however, it is a descriptive term for the mildest form of mTBI. The WHO and CDC consider a nonpenetrating head injury to be mild if structural imaging is normal, loss of consciousness is less than 30 minutes, posttraumatic amnesia is less than 24 hours, the GCS score is 13 to 15, and altered awareness is less than 24 hours. Most clinicians would argue that if their patient was rendered unconsciousness, for the sake of argument, for 29 minutes the injury would be considered far from mild. With a concussion, more than 90% of patients do not lose consciousness and if it does occur it is for only a short period of time. The anterograde amnesia is less than 30 minutes, and the GCS score is 14 or 15. Some patients may experience a slight sense of confusion for several hours. In 2013 the American Academy of Neurology (AAN) defined concussion as a “clinical syndrome of biomechanically induced alteration of brain function typically affecting memory and orientation, which may involve loss of consciousness.” While this description is accurate it is not particularly helpful for the clinician who is evaluating a patient, attempting to determine if a concussion has occurred. The Zurich Symposium on Concussion in Sport in 2016 placed emphasis on the presence of physical signs and symptoms following the head injury when diagnosing a concussion. From a clinician’s standpoint the AAN, in 1997, provided the most useful definition describing concussion as a “trauma induced alteration in mental status that may or may not result in loss of consciousness.”
Concussion occurs as a result of direct trauma, rapid acceleration-deceleration of the head such as a “whiplash” injury, or a blast injury commonly seen in military personnel serving in a war zone. In its mildest form, the patient is dazed or “star struck” and may be momentarily confused. Depending on the severity of the injury, loss of consciousness may occur followed by a brief period of amnesia, but loss of consciousness is seen in less than 10% of patients who suffer a concussion. The loss of consciousness is a result of the rotational forces exerted at the junction of the midbrain and thalamus causing a transient disruption of the reticular activating system.
While loss of consciousness does not always correlate with the outcome of a TBI, it does provide incontrovertible evidence that a significant injury has occurred and prolonged loss of consciousness is indicative of a more severe traumatic brain injury rather than a concussion or mTBI. There are some data that suggests that loss of consciousness may accentuate postconcussive cognitive dysfunction. The duration of posttraumatic amnesia, both anterograde (an inability to assimilate new memory) and retrograde (memory of events preceding the injury) may be an indication of the severity of the injury, however, it is often difficult to assess as the patient may recall what was related to them after the event by witnesses, family, friends, teammates, and coaches.
Research has been conducted to determine if blood biomarkers, including astroglial (glial fibrillary acidic protein- GFAP and S-100 calcium-binding protein B) and axonal (tau and ubiquitin C -terminal hydrolase- UCH-L1), would provide objective evidence of a concussion. To date they have shown promise in determining the severity of an injury and may predict outcomes but not specifically in identifying who has suffered a concussion.
Due to the confusion regarding the definition of concussion it is even more difficult to arrive at a consensus at to what represents a subconcussive injury. Clinicians who strictly adhere to the WHO definition of mild traumatic brain injury would likely consider a concussion without loss of consciousness or amnesia to be a subconcussive injury. What is commonly identified as a subconcussive injury may represent a concussion with axonal injury and disruption of neuronal integrity but without loss of consciousness or significant postconcussive symptoms. This type of injury can potentially be quite dangerous, especially if it occurs repeatedly in an athlete, as it is usually unrecognized and untreated and may potentially have long-term ramifications.
PATHOPHYSIOLOGY OF CONCUSSION
When trauma induces an alteration in neurologic function, a cascade of neurochemical changes develops over hours, as observed in animal studies. There is a sudden release of excitatory neurotransmitters, particularly glutamate, binding to NMDA receptors, causing the sudden release of potassium into the extracellular space followed by an influx of calcium into cell. This results in a transient hypermetabolic glycolytic state as ATP pumps become hyperactive to restore homeostasis causing depletion of energy stores and hyperglycolysis. The prolonged presence of intracellular calcium disrupts mitochondrial function. Lactate is produced impairing neuronal function and there is a reduction in cerebral blood flow. A diffuse spreading depression occurs and the state of impaired metabolism typically lasts 7 to 10 days in adult animals and during that time behavioral impairments in spatial learning are observed. During the time period of the altered metabolic state neural tissue is more vulnerable to repeated injury. Diminished blood flow to the brain may persist for days to weeks. Axonal injury occurs but there is generally little cell death, however chronic structural changes may evolve over time ( Fig. 6.7 ). MRI spectroscopy and functional MRI studies in humans following a concussive injury reveal neurometabolic changes that correlate with the animal model, but recovery may occur over a longer period of time in humans.

EVALUATION OF CONCUSSION
Most traumatic brain injuries are mild, and concussion is the mildest form. More than 90% of patients who have suffered a concussion do not lose consciousness and when it occurs it is brief, lasting usually no more than 30 seconds, and posttraumatic amnesia is less than 30 minutes. The neurologic exam does not reveal focal abnormalities and the GCS score is 14 to 15. Guidelines for performing an imaging study of the brain are discussed in the previous section. Conventional neuroimaging of the brain with CT and MRI scans usually contributes little to the evaluation of concussion as routine imaging does not detect microscopic axonal injury. Diffusion tensor imaging, however, is an MRI technique that assesses white matter microstructural integrity and has been shown to detect white matter injuries in mTBI but whether the findings are clinically useful remains controversial.
When a patient is evaluated in a hospital emergency room and diagnosed with a mild traumatic brain injury they are usually observed for a few hours. If their exam is normal, and there are no worrisome symptoms, they are discharged to home to the care of a responsible party with a written list of instructions including symptoms that would warrant a reevaluation at the hospital. After a thorough evaluation has been performed there are no data to support waking the patient up at regular intervals during the night.
POSTCONCUSSION SYNDROME
Postconcussion syndrome describes a constellation of symptoms that occur after suffering a concussion ( Box 6.2 ). Headache is the most common postconcussive symptom followed by dizziness which is more a sense of disequilibrium and imbalance than objective vertigo. Patients may also report orthostatic lightheadedness indicating mild autonomic dysfunction. A sense of mental “fogginess” is usually noted with word finding difficulty and mild problems with short-term memory and concentration. Reaction time is often slow. Nausea may be present in the first few days but usually quickly disappears. Emotional lability is not uncommon and other symptoms include photophobia, phonophobia, tinnitus, fatigue, and irritability. Initially patients experience hypersomnia but if symptoms persist, they usually develop insomnia. Patients report difficulty focusing with their vision and specifically problems with visual tracking. If symptoms persist, anxiety and depression may develop and patients with a history of an underlying mood disorder may experience a worsening of symptoms.

Full access? Get Clinical Tree
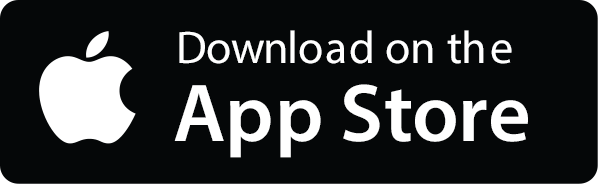
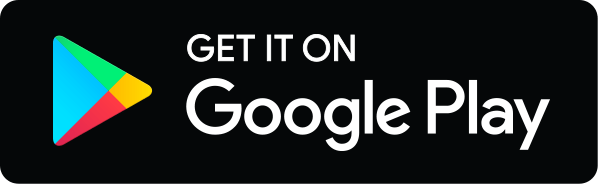