Abbreviations
AIS
American Spinal Injury Association Impairment Scale
CRPS
complex regional pain syndrome
EES
epidural electrical stimulation
EMG
electromyography
FBSS
failed back surgery syndrome
IPG
implantable pulse generator
MRI
magnetic resonance imaging
SCI
spinal cord injury
SCS
spinal cord stimulation
SCSr
spinal cord stimulator
Introduction
Spinal cord injury
Spinal cord injury (SCI), which literally means damage to the spinal cord, is a devastating and debilitating neurological condition, affecting millions of people worldwide. Traumatic SCI caused by external impacts accounts for the majority of all SCI cases and always accompanies a secondary injury that causes the further death of neurons and glial cells, leading to long-term neurological defects and paralysis. It can significantly harm the spinal cord’s functions and burden patients, their families, and friends with substantial personal and socio-economic pressures. According to the 2020 SCI datasheet reported by the National Spinal Cord Injury Statistical Center, approximately 294,000 persons are living with SCI in the United States, with about 17,810 new cases every year, while the World Health Organization (WHO) had estimated that 250,000–500,000 people suffer an SCI each year around the world ( ; ). Clearly, SCI has caused a significant burden on the healthcare system all over the globe.
Etiologically, SCI can be categorized into two types: traumatic SCI and non-traumatic SCI. Traumatic SCI is caused by external physical impacts such as vehicle accidents, sports injuries, violence, or falls. In a traumatic SCI, the external impact firstly causes bone, blood vessel, and ligament damage of the spine, then the debris and dislocation subsequently hurt and rip the spinal cord tissue, initiating the primary injury. After that, it leads to a secondary injury cascade ( Fig. 1 ), resulting in the death of glial cells and neurons, and a long-term neurological defect ( ). Differently, non-traumatic SCI is usually caused by other chronic or degenerative disorders such as tumors or infection, which however can induce a similar level of neurological deficit to a traumatic one. Despite its existence, non-traumatic SCI rarely happens compared with the traumatic SCI that accounts for over 90% of all SCI cases ( ).

With the improvement in emergency healthcare and medical services, many patients can survive from traumatic SCI and usually live up to a very optimistic life expectancy, however, frequently accompanied by terribly devastating disabilities. The injury cascade leads to complications such as inflammation, ischemia, and subsequently ruin in organization and structure of the spinal cord. Indeed, the clinical outcome is also heavily subject to the location and severity of the injury, since the patients may be partially or wholly paralyzed—depending on the lesion intensity—lose the sensory and motor functions below the level of the lesion, resulting in different extents of disability such as paraplegia and quadriplegia. If the sensory and motor function is totally lost below the injury level, it is clinically defined as a complete SCI; but if there is still any spare function left, it is called an incomplete SCI ( ). Regardless of different levels of disability, the loss of independence and ability returning to a job and daily life causes immense difficulties, extra works, and costs in caring for SCI patient’s daily living. The lifetime cost for each SCI patient is estimated to be US$2.35 million ( ), which is definitely a considerable amount for many families, especially under the circumstance that the patient themselves cannot often work in their regular jobs anymore. Besides the loss of sensorimotor functions, chronic pain is another common problem, which frequently happens after SCI, with a rate of about 30%–80% and strikingly one third of which suffer from severe pain ( ; ). Consequently, understanding SCI’s pathophysiological mechanism and developing effective treatment to this condition is critical and highly significant.
Treatments for SCI
Over the years, although substantial research and efforts have been contributed to study the SCI, there is still no known method to reverse or cure the damage of SCI at present. Fortunately, different strategies have been found to improve the lost sensorimotor functions, self-independence, and life quality of SCI patients; and other promising treatments are also in progress. Currently, available treatments for SCI are mainly in three aspects: intervention shortly after the injury happens (acute stage), compensatory strategies, and functional recovery in sub-acute and chronic stages ( ). The primary purpose of interventions after acute SCI is to decompress the spinal cord and minimize secondary injury. The typical early intervention approach includes surgical and pharmacological interventions. Though applications on animal models had suggested beneficial outcomes of surgical interventions, its effect on human trials remains unclear ( ). Also, despite some successful cases, the ability of pharmacological interventions to improve neurological function remains controversial at present and requires more demonstrations in patients ( ). The compensatory strategies usually help improve functions by utilizing assistive devices or training modalities to shift the tasks to unaffected body parts. Compensatory strategies are commonly employed to complete SCI, which has relatively limited restoring capability of the spinal cord functions ( ; ). On the other hand, functional recovery strategies attempt to restore the functions after SCI by means such as stimulations or neurorehabilitation training. These strategies normally show better outcomes for less severe SCI since incomplete SCI has a higher chance of functional recovery; however, stimulation-induced motor function recovery after complete SCI is also heavily investigated ( ; ). A promising rehabilitative strategy to SCI in recent years is spinal cord stimulation (SCS), which has shown encouraging outcomes in not only functional recovery but also SCI pain relief even in patients who did not expect to improve any function with classical interventions ( ). In this chapter, we have discussed the principles behind the SCS-mediated rehabilitation of sensorimotor functions after SCI, introduced several notable spinal cord stimulators, and illustrated the procedures of their clinical application.
Spinal cord stimulation
Principles of SCS
SCS has been used to treat neuropathic pains for over 50 years. It is often applied to alleviate chronic pain for which conventional treatments have failed, such as congenital or acquired chronic low back and leg pains, complex regional pain syndrome (CRPS), failed back surgery syndrome (FBSS), and post-SCI pains, etc. ( ). SCS blocks pain but not cures it. SCS relieves pain by generating electric pulses at the spinal cord. Its working principle to block pain is the gate control theory of pain ( Fig. 2 ). Gate control theory indicates that other inputs will shut the “nerve gate” for the painful input in the spinal cord, preventing pain signals from reaching the brain so that one does not feel the pain ( ). Based on this theory, the stimulator produces electrical pulses on the spinal cord’s dorsal surface, which closes the “nerve gate” to pain signals, prevents them from being received by the brain, thus masks the neuropathic pains ( ).

Besides, SCS starts to serve as a promising tool for movement restoration after SCI. Both invasive and non-invasive SCS could be a powerful tool. The exact principle underlying the motor function improvement by SCS is largely unknown at present. Some findings suggest that even isolated from the brain the spinal circuitry is still able to generate locomotor activity driven by the externally applied stimulation ( ; ; ). According to this principle, the SCS replaces the brain to generate stimuli for triggering locomotor-like activity for the paralyzed. So SCS with different patterns, amplitudes, frequencies, pulse widths at different locations may result in different locomotor activities. Theoretically, these motion activities could happen only if SCS is applied to the patient sustainedly. However, another explanation suggests that instead of replacing the brain, the SCS enables the brain to recruit the spared and functionally silent nerves residue in SCI, so the reorganization of the residual neural pathway by the SCS promotes and improves locomotor functions ( ). In this theory, the brain is still the command center of the limb movement, and the role of SCS is just to activate the residual neural pathway important for locomotion. So, it is possible that after a period of SCS, the locomotor improvement became long-term and sustained even after the SCS is removed.
Functional rehabilitation with SCS
Though the detailed mechanisms remain largely unclear, many studies found that SCS has benefits on motor function recovery after incomplete or complete SCI, notably in patients’ voluntary movement, standing, and stepping recovery as well as cardiovascular and bowel functions management ( Table 1 ). propose that tonic SCS could modulate spinal cord circuitry and enable patients with paralysis after SCI to undertake tasks such as standing and stepping. They reported a case in 2011 that a paraplegic patient was able to undergo a full-weight bearing standing and some leg movements during task-specific SCS ( ). The subject in the study had a clinically complete loss of voluntary motor function but incomplete sensory SCI, so the regain of some voluntary functions in this study is interpreted by that the residual but silent neuropathway was reactivated or the neuroplasticity was promoted by the SCS ( ). However, in a later study reported in 2014, they found that even for the patients diagnosed with a complete motor and sensory SCI, SCS can still help to proceed detectable leg movements after the paralysis for years ( ). This finding uncovers that SCS could be a potential rehabilitative tool for diagnostically complete SCI even a long time after the injury happens. These previous studies apply a relatively low stimulation frequency—usually below 30 Hz—for SCS, and patients still need some external assistance to stand. In 2015, they also reported that two motor-complete but sensory-incomplete SCI patients were able to process full-body weight standing without using any external assistance ( ). In this study, a higher stimulation frequency (25–60 Hz) relative to that in previous studies (< 30 Hz) lends to an EMG pattern more effective for patients to stand. Also, a body weight-bearing sensory input could remarkably increase the EMG activity to sufficient for standing, while very little EMG activity was generated by lower limb muscles during epidural SCS. The research by Sayenko et al. also demonstrated that the stimulation parameters and sensory conditions significantly influence the SCS outcomes, showing different stimulation responses between standing and supination ( ). Besides lower limb movement recovery, SCS at the cervical level was also successfully applied to improve upper limb motor functions and promote voluntary hand movements for patients with tetraplegia ( ; ).
Paper | n | Motor complete/incomplete (AIS) | Spinal cord stimulator | Trainings | Therapy duration (months) | Therapy intensity (sessions/week) | Total number of training sessions | Training outcomes or the improvement in ISNCSCI Motor Score: mean (range) |
---|---|---|---|---|---|---|---|---|
5 | Complete (A) | Finetech Medical’s cough stimulation system + Ardiem Medical’s wire lead electrodes | Bowel management (cough restore) training | 5.25 | 14–21 | 294–441 | Restore cough and improve bowel management | |
2 | Complete (A) | Abbott’s Tripole and Proclaim Elite + 16-electrode paddle array | Simultaneous stimulation and assessment | No training period | No training period | No training period | Restore some supraspinal control over motor function below the level of injury: volitional motor control, cardiovascular function, bowel-bladder synergy | |
4 | Complete (A/B) | Medtronic’s Restore ADVANCED + 16-electrode array | Spinal cord epidural stimulation for cardiovascular function | 0.5 | 2.5 | 5 | Activate mechanisms to elevate blood pressures to normal ranges from a chronic hypotensive state in SCI | |
3 | Incomplete (C/D) | Medtronic’s Activa RC + 16-electrode paddle array | Cycling + locomotor training | 5 | 5 | 108 | 10.3 (4–16) | |
4 | Complete (A/B) | Medtronic’s epidural arrays + neurostimulators + programming devices | Locomotor training | Range 6–20 | 7 | 168–560 | 0.25 (0–1) | |
1 | Complete (A) | Medtronic’s RestoreSensor SureScan MRI + 5–6-5 Specify 16-electrode array | Locomotor training | 0.5 | 4 | 8 | Enable volitional control of task-specific muscle activity, rhythmic muscle activity to produce step-like movements while side-lying, independent standing | |
1 | Complete (B) | Medtronic’s Restore ADVANCED + 5–6-5 Specify 16-electrode array | Activity-based training/task-specific training | 16 | 5 | 80 | From no volitional muscle activation to standing, stepping, volitional leg movement | |
3 | Complete (B) | Boston Scientific’s Precision Plus + Artisan 16-electrode epidural array | Volitional hand function | 24 | 1–7 | 96–672 | 19.5 (16–23) | |
4 | Complete (A/B) | Medtronic’s Restore ADVANCED + 5–6-5 Specify 16-electrode array | Stand training | 16 | 5 | 80 | Stand over-ground bearing full body weight without any external assistance | |
4 | Complete (A/B) | Medtronic’s Restore ADVANCED + 16-electrode array | Locomotor training | 3–7 | 7 | 21–49 | Able to generate EMG activity and movement during ankle dorsiflexion in the presence of epidural stimulation | |
4 | Complete/incomplete (B/C) | Medtronics Restore ADVANCED + four 4-array fine wire electrodes | Locomotor training | 12 | 3–5 | 156–260 | 12.8 (1 − 21) | |
1 | Complete (B) | Medtronic’s Restore ADVANCED + 16-electrode array | Standing + locomotor training | 7 | 1.7 | 170 | Full weight-bearing standing for 4.25 min, supraspinal control of some leg movements | |
1 | Incomplete (C) | X-trel 3470 implanted receiver + implanted quadripolar electrode leads (PISCES-Quad Plus, Model 3888) + external transmitter (X-trel, Model 3425) | Locomotor training | 7 | 5 | 140 | Reduction in sense of effort for over ground walking from 8/10 to 3/10 (Borg scale), and was able to double his walking speed reached maximum walking speeds of 0.35 m/s, and was able to ambulate over 325 m |
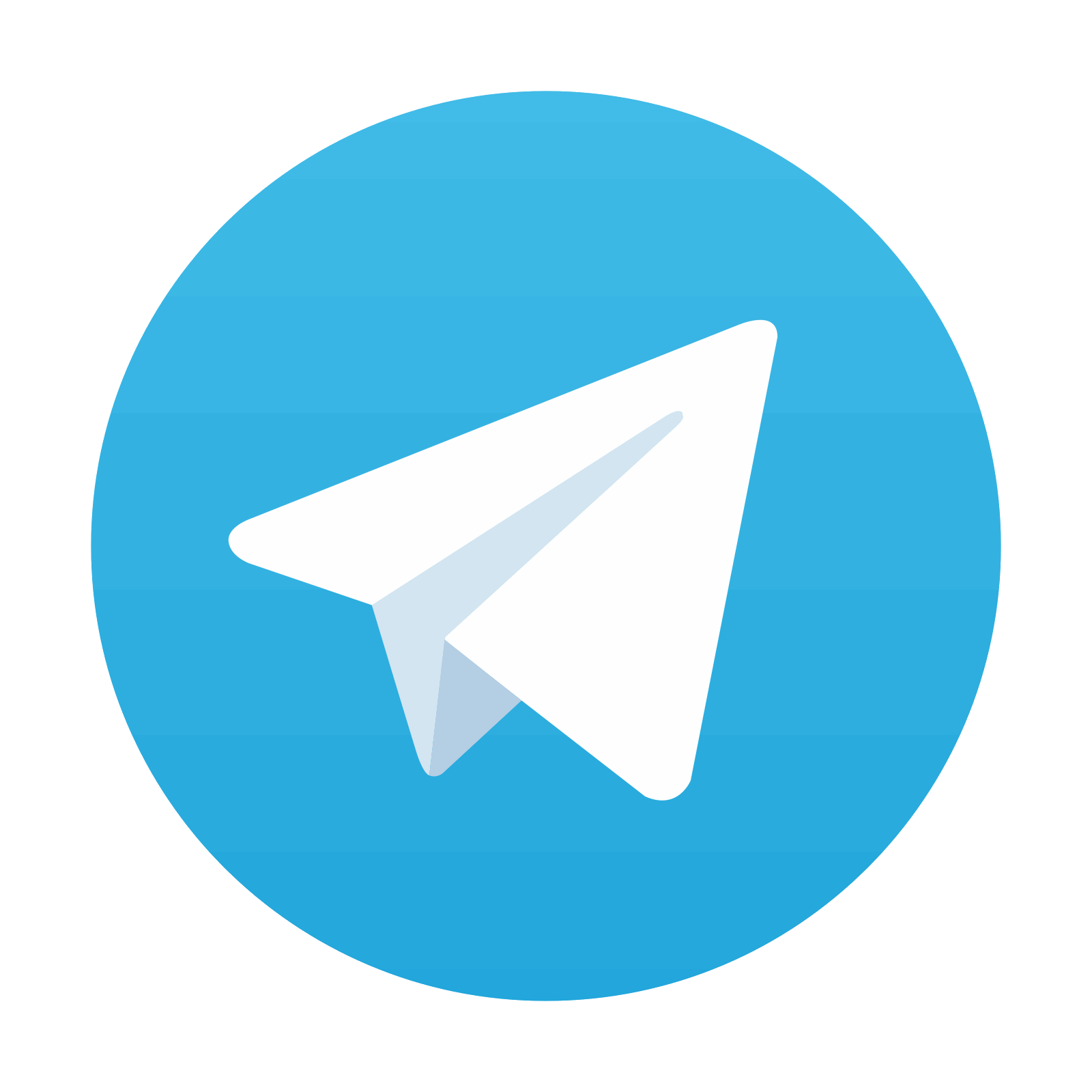
Stay updated, free articles. Join our Telegram channel

Full access? Get Clinical Tree
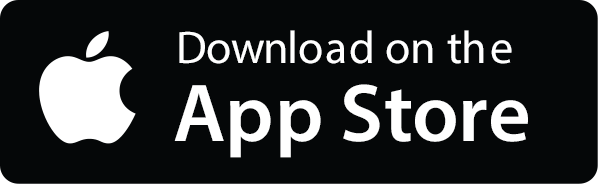
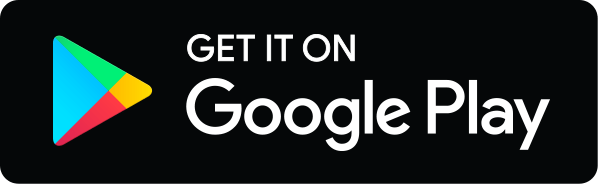
