Fig. 22.1
Electroporation (a) By application of brief electric pulses it is possible to transiently permeabilise the cell membrane (electroporation). This allows free passage of molecules to the cell cytosol over a matter of minutes. Bleomycin cytotoxicity is augmented over 300-fold when administration is combined with delivery of electric pulses. (b) As the electric pulses are subsequently applied, cells are permeabilised and the drug enters. After a few minutes, cells reseal and the extracellular drug is washed out while the internalized molecules remain trapped intracellularly. (c) Bleomycin is cytotoxic once in the cytosol and it works by cleaving DNA strands, quantified as 10–15 DNA strand breaks per DNA molecule. Bleomycin induces single-strand and double-strand DNA breaks with a ratio of 10:1
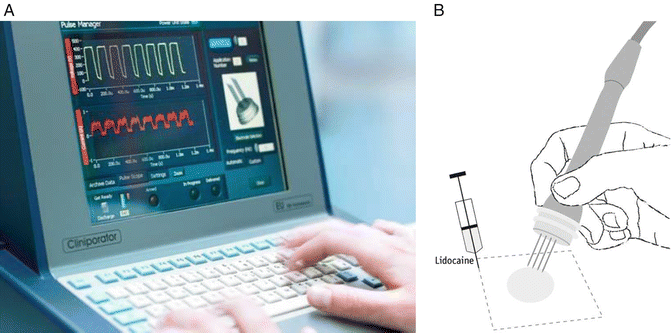
Fig. 22.2
The electrochemotherapy procedure. (a) The cliniporator equipment allows monitoring of voltage and current during the pulse. (b) The application of pulses to skin tumors must be preceded by local or general anesthesia
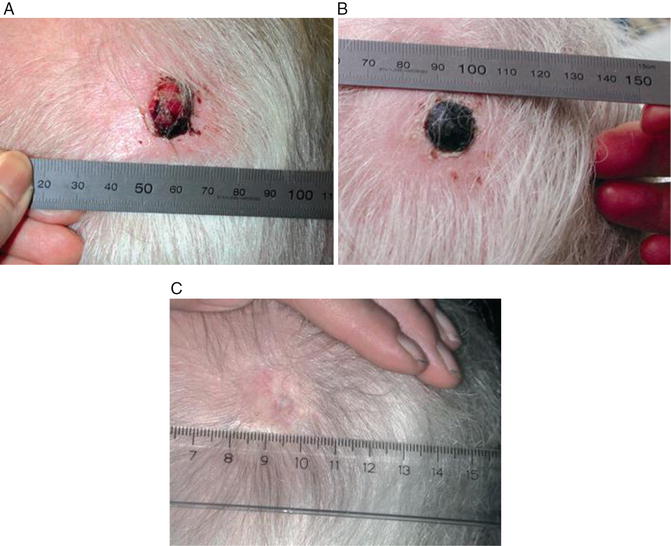
Fig. 22.3
Treatment result of electrochemotherapy in the skin. Course of treatment for patient with malignant melanoma metastases, one of eight treated is shown. The patient was treated with electrochemotherapy in general anaesthesia and intravenous injection of bleomycin. Pictures shows (a) Before treatment the metastases was ulcerated and caused haemorrhage, pain and discomfort, (b) 1 month after treatment the lesion is covered with a crust, needle marks in normal tis- sue are visible due to treatment of the tumor margin as well. Note that there is no necrosis of normal skin, and (c) 6 months after treatment the treated metastases is in CR (complete response) showing normal skin that had healed underneath the nodule (From Gehl, Ugeskrift for laeger, 2005, with permission)
To perform electrochemotherapy, it is necessary to apply electric pulses to the tissue via electrodes. The electrodes available up until now have primarily been designed to be used on skin (Fig. 22.2a, b). Different groups are now working at developing electrodes for use in internal organs, and we have in corporation with a medical device company developed an electrode suitable for brain tissue. Indeed, for the initial definition of treatment parameters, important data has been obtained by treating cutaneous metastases. This chapter reviews the basis for electrochemotherapy as well as preliminary results on its use in the brain and lists perspectives for the technology.
Clinical Challenges of Brain Metastases
It is a paradox that general improvement of cancer treatment today is both leading to prolonged survival of cancer patients and to an increased number of patients who live long enough to develop brain metastases. The risk of developing brain metastases increases in breast cancer patients, when there are metastases to the liver, lungs and lymph nodes (Ryberg et al. 2005), i.e. with the advancing stage of the cancer. The treatment of brain metastases are, for single or a limited number of metastases, surgery or stereotactic radiosurgery. For multiple brain metastases the evidence based treatment is whole brain radiation therapy (WBRT) and in some cases systemic chemotherapy or other anti-cancer drugs. Still, the overall prognosis is poor with a reported median survival of 10–12 months after treatment with surgery or stereotactic radiosurgery and a reported median survival of 4–6 months after WBRT (Videtic et al. 2009).
The patient’s quality of life is related to their neurocognitive function, which is the ability to do daily activities (ADL), such as recognizing safe and unsafe behavior, memory, and compliance with medical treatments. It has been established, that tumor regression of brain metastases after whole brain radiation therapy (WBRT) is correlated with an increased survival and preservation of neurocognitive function (Li et al. 2007). Another study concluded that the tumor volume before treatment was the only predictor of decreased neurocognitive function (Meyers et al. 2004). Some patients have a better prognosis than others, and several positive prognostic factors have been found, including good performance status, age > 60 years; less than three brain metastases and no extracranial metastases (Sperduto et al. 2008). Some cancer histologies also have a better prognosis, for example, in a study of 43 patients with metastatic breast cancer the median survival was 23 months after the diagnosis of brain metastases (Gori et al. 2007). Still, the effect of the available treatments is limited, and when the brain metastases progresses after whole brain radiation therapy, there are no additional standard treatments left to offer for a large number of the patients.
The role of re-irradiation of the whole brain for recurrent/progressive brain metastases is controversial, mainly due to the data on the subject being retrospective and published over a large number of years while the oncological treatments have evolved. These studies of re-irradiation of the brain may therefore have underestimated the toxicity of the treatment and treated different types of patients, with respect to disease status and performance status, which is known to influence patient survival. Response to treatment and median survival differ among the studies, probably reflecting both the difference in radiation doses, patient characteristics and period of time, respectively (AbdelWahab et al. 1997; Cooper et al. 1990; Hazuka and Kinzie 1988; Kurup et al. 1980; Sadikov et al. 2007; Shehata et al. 1974; Wong et al. 1996). The most recent studies indicate that a majority of the patients may benefit from re-irradiation of the brain in the form of complete or partial resolution of symptoms (Cooper et al. 1990; Sadikov et al. 2007; Wong et al. 1996). Opposed to these results is Hazuka et al.’s cohort treated from 1975–1986, where over half the patients failed to respond or deteriorated and 2 patients died from brain necrosis, possibly as a direct consequence of the re-treatment (Hazuka and Kinzie 1988).
Previously, cancer patients died of general disease progression, but today it is not uncommon that the only site of progression is the brain, while the cancer elsewhere in the body is controlled. For example, in the previously mentioned study of 43 breast cancer patients, 60 % of the patients only had disease progression in the brain (Gori et al. 2007). It is therefore increasingly important to prevent brain metastases and explore better treatment options for these patients.
Electroporation
Electroporation (sometimes referred to as electropermeabilization) is basically a technique used to transfer exogenous molecules into cells, by applying a voltage difference across the target tissue. The technique stems from observations made in the 1960s and 1970s showing that cell membranes are made transiently more permeable by the action of voltage pulses thus allowing diffusion of hydrophilic molecules and ions from the extracellular space to the cell cytosol and eventually to the cell nucleus (Gehl 2003). With electroporation a substantial enhancement of transport across cellular membranes can be achieved for certain molecules.
Electroporation and the Transmembrane Potential
The biophysical basis of electroporation is often introduced in terms of the evoked change in the electrical potential across the membrane (transmembrane potential). The resting transmembrane potential of the cell is mostly between −90 and −70 mV. However, if the cell is exposed to an external voltage difference (electric field), the transmembrane potential is altered. The exact relation between the external electric field and the change in the transmembrane potential is complicated, and for simple practical calculations, approximations are applied.
Two important features need to be emphasized: (1) the transmembrane potential of the cell responds linearly to the electric field strength, e.g. a doubling of the electric field strength doubles the induced change of the transmembrane potential. (2) the change in the transmembrane potential for a given electric field strength, increases linearly with the diameter of (spherical) the cell, implying that it is generally easier to electroporate large cells compared to small cells. Electroporation occurs when the transmembrane potential of the cells in the treated tissue exceeds about 200 mV (Teissie and Rols 1993). If the applied electric field is very strong, producing a transmembrane potential above approximately 1,000 mV, the changes in the membrane phospho-lipid bilayer will be very pronounced, causing what is known as irreversible electroporation, which results in cell death due to prolonged adverse ion concentrations (Hojman et al. 2008). Transient or reversible electroporation is found to last for minutes at physiological temperature, depending on the voltage pulse parameters and tissue type, after which the cells regain their molecular homeostasis (Saulis et al. 1991).
Electroporation Affecting Parameters
The electrode device: To deliver the electric field to the target tissue a mechanical electrode arrangement (electrode device) is used. The size and shape of the electrodes have a large impact on the distribution of the electric field. Two types of electrode devices, the parallel plate electrode device and the needle electrode device, should be mentioned since they are common in clinical applications and commercially available. The parallel plate electrode device consists of two plates (electrodes), typically 1–2 cm2 each, and delivers the most uniform electric field, however, is applicable only in non-invasive procedures, for example treatment of small prominating tumors. The needle electrode device is available with different numbers of needles (electrodes) arranged in different formations. The needle electrode device, producing a less homogeneous electric field, is typically used to treat subcutaneous tumors because of its ability to penetrate up till about 3 cm tissue at a time.
The pulse parameters: The voltage generator charges the electrodes according to pulse parameters suitable for the particular clinical situation (Fig. 22.2a). The pulse protocols are specified by (1) the number of pulses (in a single treatment), (2) the amplitude of the pulse (voltage), (3) the duration of the pulse and (4) the frequency of the pulses (number of pulses per second). In clinical application only rectangular pulses are considered. In electrochemotherapy a complete treatment session typically consists of 8 pulses delivered as 1 pulse per second (1 Hz), and with pulse duration of 100 milliseconds.
Tissue parameters: Besides the cell diameter, other tissue related parameters may influence electroporation of the target tissue, for example the cellular density of the tissue, the shape of the cells and the general condition of the cells. For example necrotic cells within the treated tissue may distort the electric field distribution, because of local differences in the electric properties (e.g. conductivity) of the tissue. In anisotropic tissue, the efficiency of electroporation may depend on the orientation of the electrodes, since the direction of the electric field is affected by it.
The advantage of electroporation based drug and gene delivery is that by applying the optimal parameters for a given tissue, it is possible to customize delivery of a given drug or gene to a particular region encompassed by the electrodes. In neurological disease, the obvious targets are cancer in the brain (delivery of chemotherapy) and non-viral gene delivery for e.g. Parkinson’s disease. Electrodes for use in the brain are described in a subsequent section.
Choice of Chemotherapy
The chemotherapeutic drug of choice in the performance of electrochemotherapy is in our opinion bleomycin. Other chemotherapeutic drugs have been tested regarding the magnitude of enhancement of the cytotoxicity when using electroporation. When testing drugs commonly used in the clinical setting, results show an increase of cytotoxicity by a factor 300–700 for bleomycin (Gehl et al. 1998; Orlowski et al. 1988), a factor 3 and 2.3 for carboplatin and cisplatin, and for the drugs daunorubicin, doxorubicin, etoposide, and paclitaxel no effect of electroporation was found (Gehl et al. 1998). In concordance with this, other results show an enhancement of the cytotoxicity of bleomycin by a factor 5,000, and confirmed a much more limited effect of electroporation when using the drugs carboplatin and cisplatin (Jaroszeski et al. 2000). The next paragraph will clarify the nature of bleomycin, and explain additional reasons why we prefer this drug to others for electrochemotherapy.
Bleomycin
Bleomycin is an antibiotic produced from the fungus Streptomyces verticillus and was discovered by Umezawa et al. in 1966 (Umezawa et al. 1966). It is formed by a mixture of peptides and contains a unique structural component, the bleomycinic acid, and a terminal alkylamine group (Lazo and Chabner 1996). Bleomycin is a hydrophilic and charged molecule with a molecular weight of 1500 Da, and it passes the intact plasma membrane poorly (Poddevin et al. 1991).
Bleomycin is a good chelator of metals and in the presence of oxygen it can bind to ions of iron, cobalt, zinc, and copper. The bleomycin-Fe2+ complex is the most active complex (Gothelf et al. 2003). When bleomycin chelates with iron in the presence of oxygen, a production of free radicals induce DNA breaks and mediate lipid peroxidation (Bokemeyer 2008; Lazo and Chabner 1996). Bleomycin induces single-strand and double-strand DNA breaks with a ratio of 10:1 (Lazo and Chabner 1996). In particular, the double strand breaks and resulting loss of chromosome fragments have a cytotoxic effect. Bleomycin has to be internalized in the cell cytosol to have an effect and the outer cell membrane is the limiting factor (Gothelf et al. 2003). Once in the cell cytosol it will effectuate its toxicity although its way to the cell nucleus is relatively unknown. There are two scenarios depending on how many molecules of bleomycin enter the cell: (i) the cells are arrested in the G2–M phase of the cell cycle and die in approximately three doubling times with low concentration of bleomycin, and (ii) pseudoapoptosis is induced and kills the cell within minutes with high concentrations of bleomycin (Tounekti et al. 2001).
Bleomycin’s mechanism of action is effective in all steps of the cell cycle, though cells in G2/M phase are considered more sensitive because the DNA is more accessible in this phase (Mir et al. 1996). Bleomycin is mostly used in the treatment of lymphoma (Hodgkin and non-Hodgkin) and testicular cancer in combination with other antineoplastic drugs (Bokemeyer 2008; Lazo and Chabner 1996). It is also the preferred drug in treating tumors with electrochemotherapy (Gothelf et al. 2003; Heller et al. 1998; Marty et al. 2006). Bleomycin is eliminated from the blood by renal excretion (Mir et al. 1996). The most important toxic reactions affect the lungs and skin, causing pulmonary fibrosis in about 10 %, with a mortality rate of 1 %, and erythema, induration, hyperkeratosis, and peeling of the skin (Lazo and Chabner 1996). The toxicity of bleomycin increases with and is directly related to the cumulative dose received. It should be noted that normally fever occurs 48 h after intravenous drug administration in 25 % of the patients (Lazo and Chabner 1996).
Because electrochemotherapy is a once-only treatment, the doses necessary are not even near the cumulative doses that are reported to cause serious adverse effects. Therefore, the anticipated adverse effects related to bleomycin should be of a mild nature, such as flu-like symptoms and a slight fever. In the treatment of electrochemotherapy bleomycin can be administered both intravenously and intratumorally, and the adverse effects of course depends on the route of administration. The mentioned adverse effects are seen when bleomycin is administered intravenously. In the next paragraph we will get into the adverse effects seen with intratumoral administration of bleomycin in the brain.
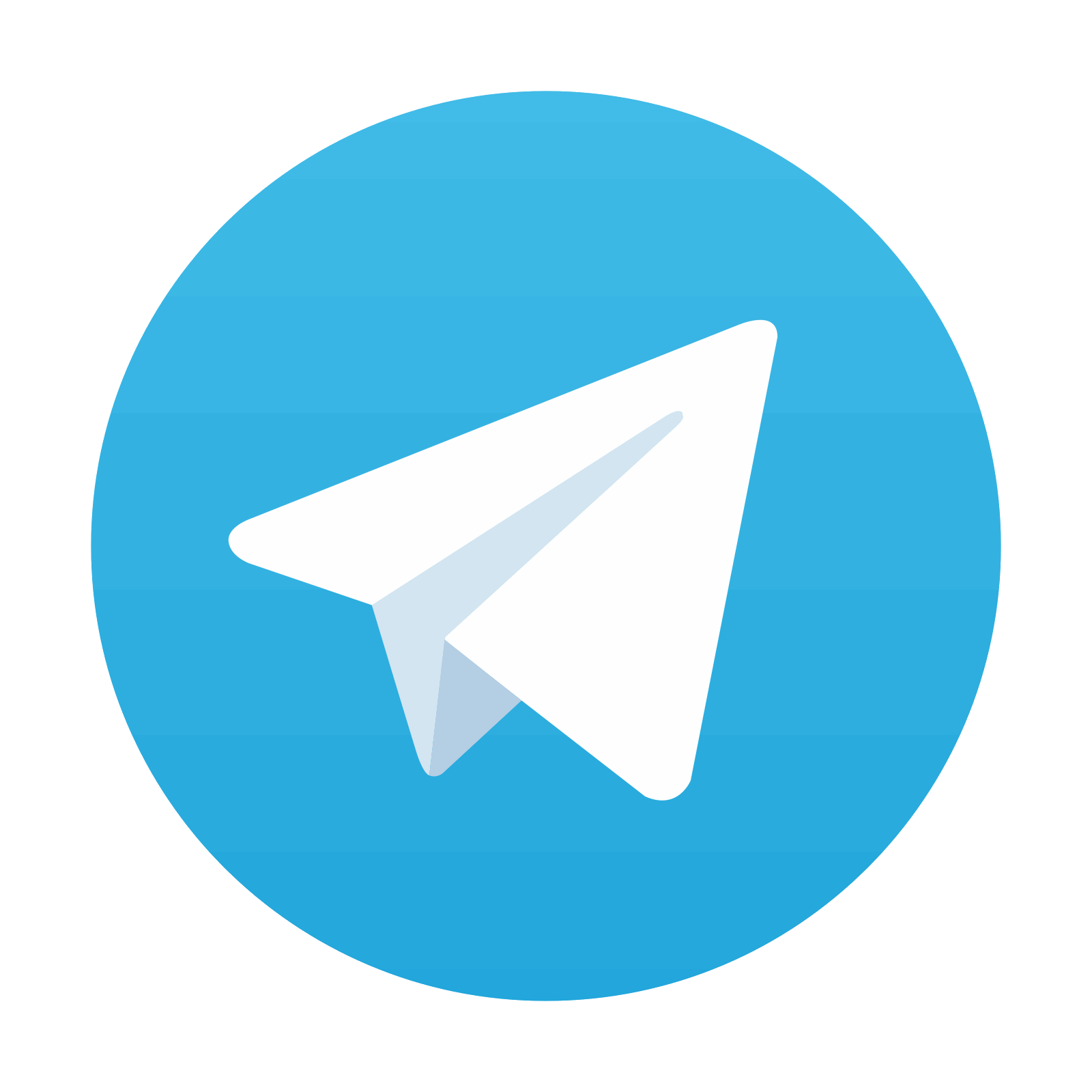
Stay updated, free articles. Join our Telegram channel

Full access? Get Clinical Tree
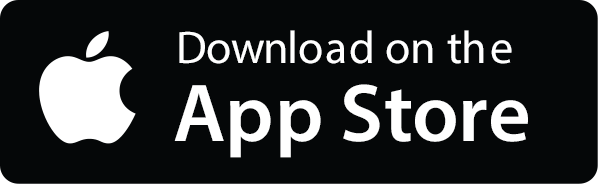
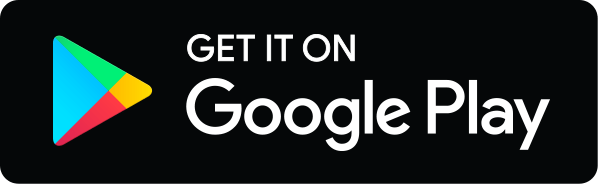