13 Treatment of Degenerative Disc Disease/Disc Regeneration: Stem Cells, Chondrocytes or Other Cells, and Tissue Engineering
Steven Presciutti and Howard An
Abstract
Intervertebral disc (IVD) degeneration remains a significant treatment challenge, but the use of stem cells has emerged as one of the most promising and exciting techniques to restore the degenerated disc. Many challenges remain. The use of stem cells in treating disc disease as well as the obstacles that remain are reviewed in this chapter in an evidence-based manner.
Keywords: intervertebral disc degeneration, mesenchymal stem cell, MSC, stem cells, tissue engineering
13.1 Historical Perspective
The intervertebral disc (IVD) is composed of the nucleus pulposus (NP), the annulus fibrosus (AF), and the two end plates of adjacent vertebral bodies. Adult NP primarily contains chondrocyte-like cells capable of synthesizing proteoglycans (PGs) and type II collagen and is contained within the AF, which princi pally contains fibroblast-like cells capable of synthesizing type I collagen.1,2 Intervertebral disc degeneration (IDD) affecting the NP is characterized by attenuation of PG synthesis, develop ment of NP dehydration, and an increase in waste products.3,4,5,6 Alterations in the cell population of the IVD have been partic ularly implicated in the pathogenesis of IDD.7 Loss of chondrogenic NP cells and their replacement by fibroblast-like cells has also been implicated in the later stages of IDD.8 Over time, an imbalance in the production and degradation of extracellular matrix (ECM) components can lead to a perturbed mechanical balance between the NP and the AF, leading to structural com promise of the disc. This can ultimately result in a variety of clinically significant spinal disorders including radiculopathy and spinal stenosis. IDD has also been linked to low back pain itself; however, the exact relationship between the two remains unclear.9,10
Conventional treatment options include multiple nonopera tive modalities (i.e., physical therapy, medication, epidural steroid injections) as well as eventually surgical options including spinal fusion or artificial disc replacement if nonoperative treatment fails.11 Although short-term pain relief with these treatment modalities has been reported, long-term outcomes have been plagued with high rates of recurrent pain.12 In addi tion, none of the aforementioned treatment strategies focus on halting or reversing the underlying disc degeneration process. In this regard, regenerative cellular therapy has attracted signif icant attention because of its potential to directly address the degenerative process. Cell-based therapies are particularly attractive in treatment of more advanced IDD, such as Pfirrmann grades IV or V(▶ Fig. 13.1).
Fig. 13.1 Grading system for the assessment of lumbar disc degeneration. (a) Grade I: The structure of the disc is homogeneous, with a bright hyperintense white signal intensity and a normal disc height. (b) Grade II: The structure of the disc is inhomogeneous, with a hyperintense white signal. (c) Grade III: The structure of the disc is inhomogeneous, with an intermediate gray signal intensity. (d) Grade IV: The structure of the disc is inhomogeneous, with a hypointense dark gray signal intensity. (e) Grade V: The structure of the disc is inhomogeneous, with a hypointense black signal intensity. Grading is performed on T2-weighted mid-sagittal images. (Reprinted with permission from Pfirrmann et al. Magnetic resonance classification of lumbar intervertebral disc degeneration. Spine 2001;26 (17):1873–1878.)
Substantial progress has occurred in the fields of regenerative medicine, tissue engineering, and stem cell therapies with the aim of treating and reversing IDD as well as augmenting and enhancing current treatments. A stem cell can renew itself through cell division and can exert beneficial effects on other cell types through a variety of mechanisms (i.e., paracrine effects, cell–cell interactions). These characteristics make stem cells worthy of further investigation as a source of cells for treating IDD. Although not currently routinely employed in the management of IDD, these therapeutic characteristics of mesenchymal stem cells (MSCs) have led to an increasing number of preclinical and clinical studies attempting to demonstrate the efficacy and safety of progenitor cells for the treatment of IDD. The first reported use of cell-based therapies to treat IDD in humans was in 2005 by Haufe et al13 and those initial posi tive results brought about intense interest among researchers and have resulted in many more preclinical and clinical studies investigating the role of cell-based therapies to treat IDD.
This chapter reviews the biology of stem cells, the clinical evi dence concerning the use of MSCs, the hindrances to clinical use, and the potential risks associated with the application of MSCs for the treatment of IDD. Due to the fact that this specific subject matter uses terminology that can often times be confus ing, a list of definitions is provided to the reader in ▶ Table 13.1.
Table 13.1 Cell-based therapy terminology
Adult stem cell | An undifferentiated cell found in a differentiated tissue that can renew itself and (with certain limitations) differentiate to yield all the specialized cell types of the tissue from which it originated |
Allogenic | Two or more individuals (or cell lines) are stated to be allogenic to one another when the genes at one or more loci are not identical in sequence in each organism. |
Autologous trans plant | Transplanted tissue derived from the intended recipient of the transplant. Such a transplant helps avoid complications of immune rejection. |
Bone marrow | The soft, living tissue that fills most bone cavities and contains hematopoietic stem cells, from which all red and white blood cells evolve. The bone marrow also contains mesenchymal stem cells that a number of cell types come from, including chondrocytes, which produce cartilage. |
Bone marrow stem cell (BMSC) | One of at least two types of multipotent stem cells: hematopoietic stem cell and mesenchymal stem cell |
Chondrocytes | Cartilage cells |
Differentiation | The process whereby an unspecialized early embryonic cell acquires the features of a specialized cell such as a heart, liver, or muscle cell |
Ectoderm | The upper, outermost of the three primitive germ layers of the embryo; it gives rise to skin, nerves, and brain. |
Embryonic stem (ES) cells | Primitive (undifferentiated) cells from the embryo that have the potential to become a wide variety of specialized cell types |
Endoderm | Lower layer of a group of cells derived from the inner cell mass of the blastocyst; it later becomes the lungs and digestive organs. |
Hematopoietic stem cell (HSC) | A stem cell from which all red and white blood cells evolve |
Mesenchymal stem cells (MSCs) | Cells from the immature embryonic connective tissue. A number of cell types come from mesenchymal stem cells, including chondrocytes, which produce cartilage. |
Mesoderm | The middle layer of the embryonic disk, which consists of a group of cells derived from the inner cell mass of the blastocyst. This middle germ layer is known as gastrulation and is the precursor to bone, muscle, and connective tissue. |
Multipotent stem cells | Stem cells that have the capability of developing cells of multiple germ layers |
Pluripotent stem cell (PSC) | A single stem cell that has the capability of developing cells of all germ layers (endoderm, ectoderm, and mesoderm) |
Precursor cells | In fetal or adult tissues, these are partly differ entiated cells that divide and give rise to differ entiated cells. Also known as progenitor cells. |
The three initial embryonic germ layers—endoderm, mesoderm, and ectoderm—from which all other somatic tissue-types develop | |
Somatic cell | Any cell of a plant or animal other than a germ cell or germ cell precursor |
Stem cell | A cell that has the ability to divide for indefinite periods in culture and to give rise to specialized cells |
Totipotent | Having unlimited capability. The totipotent cells of the very early embryo have the capacity to differentiate into extra embryonic membranes and tissues, the embryo, and all postembryonic tissues and organs. |
13.2 Determining the Ideal Cell Type
Although considerable research has been dedicated to strat egies that boost the ability of native disc cells to synthesize and maintain the ECM, the introduction of exogenous cells such as stem cells is gaining popularity as a potential treatment for IDD.7 The goal of stem cell therapy is to replace or replenish dis eased tissue through the localized differentiation of trans planted stem cells into native cells, which advance the healing process or directly restore the tissue physically. In addition, therapeutic stem cells also release trophic factors that have been shown to stimulate the metabolism of IVD cells as well as suppress inflammatory reactions.14 Choosing the appropriate cell source, however, is vital for the successful outcome of cell therapy.15 The choice of cells is based on their abundance, ease to obtain, and the capacity to differentiate into a desired cell type. The cells of choice also need to be viable in the hypoxic and the hypoglycemic environment found within the disc, with minimal or no immune response. They should have low or no risk for tumor growth.
Adult stem cells, like all stem cells, share at least two charac teristics. First, they can make identical copies of themselves for long periods of time; this ability to proliferate is referred to as long-term self-renewal. Second, they can give rise to mature cell types that have characteristic morphologies (shapes) and speci alized functions. The product of a stem cell undergoing division is at least one additional stem cell that has the same capabilities of the originating cell. Typically, stem cells generate an intermediate cell type or types before they achieve their fully differentiated state. The intermediate cell is called a precursor or progenitor cell. Progenitor or precursor cells are partly differen tiated cells that divide and give rise to differentiated cells. Such cells are usually regarded as “committed” to differentiating along a particular cellular development pathway (▶ Fig. 13.2).
Fig. 13.2 Distinguishing features of progenitor/precursor cells and stem cells. The product of a stem cell undergoing division is at least one additional stem cell that has the same capabilities of the originating cell. Shown here is an example of a hematopoietic stem cell (HSC) producing a second generation stem cell and a neuron. This is in contrast to a progenitor cell, which can yield two specialized cells upon cell division. Shown here is an example of a myeloid progenitor undergoing cell division to yield two specialized cells (a neutrophil and a red blood cell).
Most scientists use the term pluripotent to describe stem cells that can give rise to cells derived from all three embryonic germ layers—mesoderm, endoderm, and ectoderm. These three germ layers are the embryonic source of all cells of the body. All of the many different kinds of specialized cells that make up the body are derived from one of these germ layers (▶ Table 13.2). Pluripotent cells have the potential to give rise to any type of cell, a property observed in the natural course of embryonic development and under certain laboratory conditions.
Table 13.2 Embryonic germ layers from which differentiated tissues de velop
Embryonic germ layer | Differentiated tissue |
Endoderm | Thymus |
Thyroid, parathyroid glands | |
Larynx, trachea, lung | |
Urinary bladder, vagina, urethra | |
Gastrointestinal (GI) organs | |
Lining of GI tract | |
Lining of respiratory tract | |
Mesoderm | Bone marrow (blood) |
Adrenal cortex | |
Lymphatic tissue | |
Skeletal, smooth, and cardiac muscle | |
Connective tissues (including bone, cartilage) | |
Urogenital system | |
Heart and blood vessels | |
Ectoderm | Skin |
Neural tissue (neuroectoderm) | |
Adrenal medulla | |
Pituitary gland | |
Connective tissue of the head and face | |
Eyes, ears |
Because NP cells share similarities with chondrocytes in both phenotype and molecular markers, cells with the capacity of differentiating toward chondrocytes have largely been considered ideal in the treatment of IDD. Given this, autologous chondrocyte implantation has been attempted as a treatment strategy for IDD. Articular chondrocytes, such as NP cells, produce aggrecan and type II collagen and can be surgically obtained from the non–weight-bearing parts of the knee. In a study by Gorensek and colleagues,16 the NP of rabbit IVDs was removed and autologous chondrocytes were transferred to the defect site. Discs injected with chondrocytes produced a hyaline-like cartilage in the center without any elastic fibers, but with a higher collagen to aggrecan ratio than in NP ECM. In a porcine denucleation model, autologous chondrocytes injected with a fibrin carrier showed dense cartilage formation by 3 months and cell via bility up to 12 months after transplantation.17 In vitro co-culture experiments have also suggested cartilage tissue stimulates NP cell anabolism through moderation of inflammation.18 However, chondrocytes produce a lower PG to col lagen ratio than NP cells,19 so further work needs to be done to establish restoration of disc biochemical and biomechanical properties. Chondrocyte transplantation also suffers from some of the same limitations as NP cells (inva sive second surgery and low supply of donor tissue), so chondrocytes themselves may not be an ideal source for cell therapy.
Given this, multiple sources of stem cells that have the ability to become chrondrocyte-like cells (i.e., NP cells) have also been explored as potential candidates and are discussed below. A list of the completed human clinical trials using cell-based thera pies to treat IDD is provided in ▶ Table 13.3.13,20,21,22,23,24 Simi larly, ▶ Table 13.4 provides a list of the current ongoing clinical trials. 25,26,27,28,29,30
13.2.1 Embryonic Stem Cells and Induced Pluripotent Stem Cells
Embryonic stem (ES cells) are pluripotent stem cells derived from discarded early-stage frozen embryos.31One major draw back is that there are only a limited number of cell lines avail able at present for study. ES cells are able to differentiate toward all derivatives of the three primary germ layers, includ ing chondrocytelike cells.32 Sheikh et al were able to show that the subsequent transplantation of these cells into the IVD of rabbits resulted in maintenance of the native cell’s viability as well as their appropriate morphology.
Induced pluripotent stem cells (iPSCs) are similar to ES cells in both phenotype and potential for differentiation, and have also been reported to have the capacity of differentiating toward chondrocytelike cells.33 iPSCs are derived from somatic cells by inducing the expression of ectopic reprogramming transcriptional factors. Unfortunately, some of these factors are known oncogenes. Although some studies showed that these transcriptional factors can be replaced by small molecules,34 tumorigenesis is still a major concern that limits the clinical utility of both ES cells and iPSCs.35,36
13.2.2 Mesenchymal Stem Cells
MSCs are multipotent cells derived from mesodermal tissues and they have the capacity to differentiate toward some or all of the specialized cell types of that original tissue. MSCs are reported to be nonimmunogenic and, in contrast to ES cells, do not as easily undergo malignant transformation.37,38 Moreover, MSCs have the capacity for self-renewal, enabling maintenance of an undifferentiated phenotype in multiple subcultures.39 However, in the appropriate environment, MSCs are capable of differentiating into multiple cell types including osteoblasts, adipocytes, chondrocytes, and neuronal cells.40,41 The differen tiation of MSCs toward chondrocytelike cells has been docu mented both in vitro and in vivo.42,43,44,45 Although MSCs can be differentiated into chondrocytelike cells similar to those found in the NP, whether those cells are truly NP cells remains to be seen.7 We next review the various tissues that have been explored as a source for MSCs.
Bone Marrow Stromal Cells
Bone marrow is an excellent source of MSCs for most orthopae dic applications including chondrogenic differentiation for the diseased IVD. Yamamoto and colleagues46 investigated bone marrow–derived stromal cells in a direct contact co-culture sys tem with native NP cells. The authors found significantly increased cell proliferation, deoxyribonucleic acid (DNA) syn thesis, and PG synthesis in the NP cells. This was most pro nounced in the NP cells that had direct cell-to-cell contact with the bone marrow MSCs. These in vitro findings have also been extended to an in vivo rat model by Allon and colleagues.47 After injecting bone marrow–derived MSCs into the discs or rabbits, the authors were able to demonstrate an increase in PG content as well as partial restoration of both disc height and disc hydration.48,49 Similarly in the ovine model, the injection of MSCs directly into the IVD has been shown to restore disc ECM, increase disc height, and reduce both radiological and histological grading scores 6 months following injection.50,51
Early clinical research has also been promising. Yoshikawa et al22 reported two cases of autologous MSC implantation in markedly degenerative IVDs. Symptomatic and radiological improvement was demonstrated without significant adverse effects. In addition, a larger pilot study was subsequently con ducted in which 10 patients suffering from low back pain asso ciated with IDD were injected with autologous bone marrow–derived MSCs.23The authors found that improvements in pa tient-reported outcomes were similar to those observed follow ing spinal fusion. These small human studies, along with numerous animal studies, suggest that MSC implantation could indeed be a useful tool for the treatment of IDD.
Adipose-Derived Stem Cells
Given the larger number of MSCs that can be obtained compared with bone marrow or other sources as well as the relative ease of procurement, adipose-derived stem cells (ADSCs) have gained significant attention in recent years. Multiple in vitro studies have been conducted that have found these MSCs to be a viable option for IVD regeneration.52,53 Choi and colleagues40 reported that chondrogenic differentiation of ADSCs was possi ble by co-culturing them with normal, healthy NP cells in monolayer. ADSCs cultured alone or with NP cells from degen erative discs were shown to have inferior results.
More recent studies have focused on fine-tuning the chondrogenic differentiation of these ADSCs in order to be more similar to actual NP cells. Lu and colleagues54 reported that co-culture of human ADSCs and NP cells in a three-dimensional (3D) micromass culture resulted in differentiation of ADSCs into an NP cell–like phenotype. Likewise, Gaetani et al55 showed that a 3D culture technique resulted in significantly increased matrix production and a 3D cell organization similar to IVD.
Other Mesenchymal Stem Cell Sources
Other stem cell sources have also been investigated for the treatment of IDD, although most of these other cell types are just beginning to be explored. Their true translational promise for the treatment of IDD remains to be seen. Vadala et al56 investigated the use of adult muscle tissue as a stem cell source. They used a co-culture in vitro model with NP cells and were able to show increased matrix production and DNA synthesis of the NP cells. Recent in vitro studies have also revealed the potential for human AF cells57 and NP cells58 to serve as a source of MSCs. The morbidity of harvesting one’s own disc cells is a concern for the induction of further degeneration, however. Others studies have recently investigated the use of synovial cells as a stem cell source for IVD tissue engineering.59,60,61
Sakai and colleagues62 demonstrated a population of progen itor cells within the actual NP itself. Although they were able to indentify such cells, the proportion of these cells within the disc was markedly reduced with age and degeneration. Other stud ies have also found highly proliferative cells expressing stem cell markers (i.e., Notch1, Delta4, Jagged1, C-KIT) within the disc.63,64 These cells have been successfully isolated and have been shown to be capable of differentiating down the osteogenic, chondrogenic, and adipogenic lineage.65 Although these endogenous stem cells offer huge potential, it remains to be seen whether they can be harnessed for regenerative purposes.
Human umbilical cord MSCs are multipotent and have been reported to differentiate into chondrogenic, adipogenic, and osteogenic lineages.66But the safety of allogenic transplantation in MSCs has not yet been established. Until further studies establish the immunologic safety of allogenic human umbilical cord MSC transplantation in humans, the utility of human umbilical cord MSCs is limited for the purpose of IDD treatment.
13.3 Understanding the Target Tissue
13.3.1 Influence of the Intervertebral Disc Microenvironment
To produce matrix, disc cells require an extracellular environ ment with sufficient oxygen (> 3%) and glucose (1 mM) and the pH must preferably not be acidic (optimally, ~7.0–7.2). In addi tion, the osmolarity must be high enough to stimulate matrix production as well as retain it locally (ideally, 350 mOsm). These conditions are present in a normal, healthy disc67 but in degenerative discs, osmolarity falls as aggrecan is lost, oxygen levels are variable, and the pH tends to become acidic.68These microenvironmental conditions are very specific, quite harsh, and differ from the native environments of the cells being con sidered as possible sources of MSCs (▶ Fig. 13.3). This could impair the functioning of any such implanted cells or even result in their death.69
Fig. 13.3 The intravertebral disc (IVD)-specific niche is an obstacle to successful IVD regener ation using cell therapy. The specialized micro environment of the IVD is characterized by low oxygen level, low pH, and poor nutrient supply, factors that present a major challenge to the survival and function of injected cells.
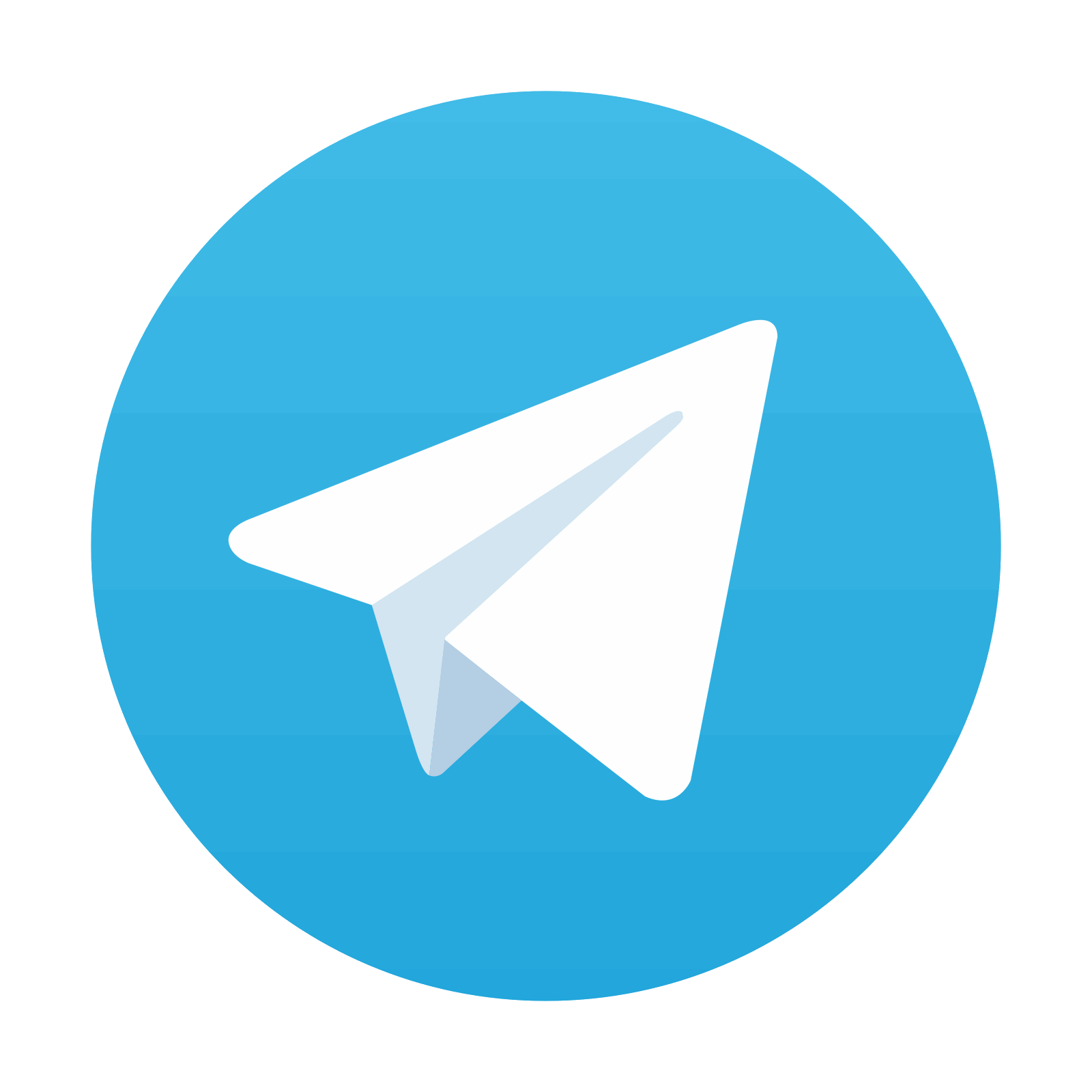
Stay updated, free articles. Join our Telegram channel

Full access? Get Clinical Tree
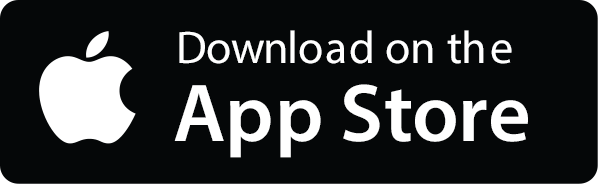
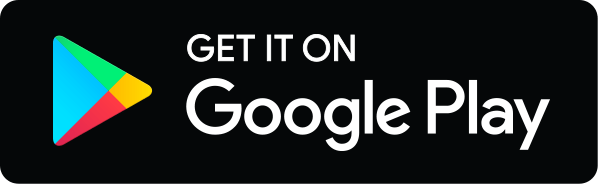