Trends in Endovascular Surgery
Objectives: Upon completion of this chapter, the reader should be able to identify with advances in the endovascular management of cerebral ischemic diseases, arteriovenous malformations, and aneurysms.
Accreditation: The AANS* is accredited by the Accreditation Council for Continuing Medical Education (ACCME) to sponsor continuing medical education for physicians.
Credit: The AANS designates this educational activity for a maximum of 15 credits in Category 1 credit toward the AMA Physician’s Recognition Award. Each physician should claim only those hours of credit that he/she spent in the educational activity.
The Home Study Examination is online on the AANS Web site at: http://www.aans.org/education/books/controversy.asp
* The acronym AANS refers to both the American Association of Neurological Surgeons and the American Association of Neurosurgeons.
The development of endovascular surgery over the past two decades has dramatically affected methods for the management of cerebrovascular disease today. Endovascular surgery is in its infancy, and its potential for the future is great. In this chapter, we describe our perspective on the future of endovascular surgery in the management of cerebral ischemic disease, intracranial arteriovenous malformations (AVMs), and aneurysms.
Management of Cerebral Ischemic Disease
Patients who experience cerebrovascular ischemic symptoms today face a substantially lower risk of death, recurrent symptoms, and stroke than they did a decade ago. Progress in the management of cerebral ischemic disease is, in part, because of advances made in the treatment of coronary artery disease. Success observed in the management of coronary artery disease resulted from the rapid transfer of knowledge gained from laboratory investigations to the clinical setting; execution of well-designed, randomized, placebo-controlled trials; and technical innovation and refinement of percutaneous coronary intervention procedures. This systematic approach allowed the development of evidence-based management protocols. The three main strategies currently used in the management of cerebral ischemic disease reflect lessons learned from cardiology. The strategies are restoration and maintenance of blood flow at the site of occlusion, reduction of infarct size, and stabilization of the cerebrovascular vessel wall and vessel wall interaction with the bloodstream.
Restoration and Maintenance of Cerebrovascular Flow at the Lesion Site
This strategy aims to optimize the use of mechanical revascularization techniques and pharmacologic agents that affect coagulation, fibrinolysis, and platelet function to achieve timely, adequate, and durable cerebral reperfusion. In the past 10 years, significant progress has been made in the development and assessment of thrombolytic therapy for ischemic stroke. Recombinant tissue plasminogen activator (tPA) is currently the only drug approved by the FDA for acute thrombolysis in stroke. The National Institute of Neurological Disorders and Stroke trial demonstrated that patients treated with intravenously administered tPA within 3 hours of stroke symptom onset were 30% less likely than patients treated with placebo to have disabilities at 3 months. Unfortunately, symptomatic intracranial hemorrhage occurred in 6.4% of patients, and the overall rate of mortality at 3 months was 17%. The main limitations of systemic thrombolysis are the narrow therapeutic window of 3 hours, the need for high doses of thrombolytic agents leading to an increased risk of systemic or intracranial hemorrhage, and the inability to visualize the occlusion site by means of angiography or other imaging modality. In the cardiology literature, some evidence exists that a better strategy for acute myocardial revascularization may be prompt intra-arterial intervention. Theoretically, intra-arterial delivery of thrombolytic agents is more likely to increase the reperfusion rates and decrease the morbidity and mortality rates associated with higher doses of thrombolytics administered for systemic treatment. These theoretical advantages have been evaluated in trials of intra-arterial thrombolysis with prourokinase, tPA, and urokinase for the management of acute ischemic stroke (Table 2-1). Although these agents did not receive FDA approval for clinical use, the results of these trials demonstrated therapeutic efficacy, even when the agent was administered late in the ischemic process. The average time from symptom onset to treatment was 5 hours.
The future of intra-arterial therapy for the management of acute stroke depends on improving recanalization rates and safety. At the University at Buffalo Department of Neurosurgery, we used reteplase, a third-generation recombinant tPA, for intra-arterial thrombolysis. Our preliminary results with the administration of heparin (activated coagulation time of 250 seconds) in combination with a maximum dose of 8 units of reteplase demonstrated neurological improvement (defined as a >4-point decrease in National Institutes of Health Stroke Scale score) in 7 (44%) of 16 patients at 24 hours.8 Complete or near-complete recanalization (thrombolysis in myocardial infarction, TIMI; grade 3 or 4 flow) was achieved in 14 (88%) patients, and symptomatic intracerebral hemorrhage occurred in only one patient. Reteplase has a longer half-life and binds less to fibrin than alteplase (second-generation recombinant tPA). These improvements permit more penetration within the thrombus and prolonged thrombolytic activity. Despite the theoretical advantages of third-generation thrombolytic agents (reteplase), their efficacy in obtaining TIMI grade 3 or 4 flow is often limited. One limitation is that thrombolytic therapy stimulates the production of thrombin and the consequent reactivation of platelets. Platelet aggregation leads to the formation of a thrombolytic-resistant thrombus and the recurrence of thrombosis. In addition, platelets secrete plasminogen activator inhibitor (PAI)-l, which inhibits tPA penetration of thrombus. We are evaluating the safety and effectiveness of mechanical thrombolysis using balloon angioplasty, snare, and stents in conjunction with intra-arterial reteplase for ischemic stroke.
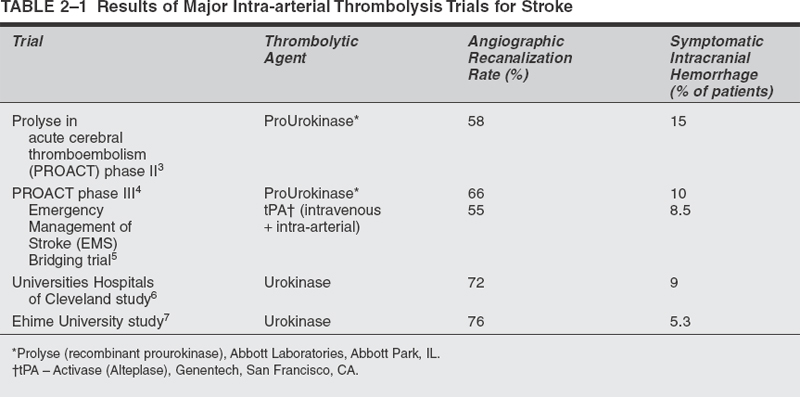
In the cardiology experience, numerous advantages of administering adjunctive antiplatelet agents along with thrombolytic therapy were recognized. Among these advantages are accelerated reperfusion, decreased incidence of reocclusion, improved microvascular reperfusion, and enhanced safety and efficacy. Major trials have demonstrated the effects of the combination of a full dose of thrombolytic agents and Ilb/IIIa inhibitors for the treatment of coronary artery disease (Table 2-2). Two trials have tested half-dose thrombolytic (alteplase or reteplase) therapy with full-dose abciximab for the management of acute myocardial infarction.13,14 An added benefit of this approach might be a decreased risk of hemorrhagic complications. In the TIMI-14 trial,14 the most effective regimen consisted of half-dose tPA (alteplase), full-dose abciximab, aspirin, and heparin. This regimen restored TIMI grade 3 flow in 77% of patients at 90 minutes, with a major hemorrhage rate of 1 %. In the Strategies for Patency Enhancement in the Emergency Department (SPEED) trial,13 the use of a half dose of reteplase (two 5-unit boluses 30 minutes apart) along with full-dose abciximab, aspirin, and heparin resulted in restoration of TIMI grade 3 flow in 62% of patients with myocardial infarction at 60 to 90 minutes after initiation of therapy. In Buffalo, we have started a prospective, nonrandomized, open-label trial to evaluate the safety of an escalating dose of intra-arterial reteplase in combination with intravenous abciximab for patients with acute ischemic stroke. According to the protocol, patients received intravenous heparin (30 units/kg bolus) to maintain an activated coagulation time between 180 and 250 seconds and then intravenous full-dose abciximab (0.25 mg/kg bolus followed by a 12-hour infusion at 0.125 mcg/kg/min to a maximum dose of 10 mg/min) and intra-arterial reteplase (maximum total dose of 2 U) .8 If the results from interventions for acute myocardial infarction are transferable to those for acute stroke, we can expect improvement in reperfusion rates with reduced rates of intracranial hemorrhage.
Another reperfusion strategy that has been explored for the treatment of coronary artery disease is the combination of fibrinolytic therapy with mechanical thrombolysis (stent and/or percutaneous transluminal coronary angioplasty).15–17 TIMI grade 3 flow was achieved in 73% of patients who underwent percutaneous revascularization with primary angioplasty, and the average door-to-balloon time (interval from emergency room evaluation until treatment) was 114 minutes. In the Primary Angioplasty in Myocardial Infarction (PAMI) Stent trial (primary stenting),18 TIMI grade 3 flow was achieved in 89% of patients, and the door-to-stent time was 135 minutes. In the Plasminogen-Activator Angioplasty Compatibility trial (PACT),17 patients received half-dose tPA followed by immediate angiography and percutaneous revascularization if needed. At coronary angiography, 33% of patients had TIMI grade 3 flow in infarct-related arteries. This observation is of great importance because it suggests that patients can benefit from early intravenous thrombolysis while awaiting the initiation of percutaneous revascularization therapy. The combination of pharmacologic and mechanical intervention is a venue to be carefully explored in the management of acute stroke. The treatment of acute stroke is benefiting from future development of pharmacologic (heparinoid, antithrombin agents, and antiplatelet agents) and mechanical (using clot retrievers18 and stents) thrombolysis strategies.
An interesting tendency observed in the cardiology literature is to perform early invasive revascularization therapy for non-ST segment elevation acute coronary syndromes. This syndrome involves nonocclusive coronary stenosis in patients with unstable angina. The lesions are more or less the equivalent of intracranial stenosis with “misery perfusion” or stage 2 hemodynamic failure (i.e., the presence of reduced blood flow and increased oxygen extraction fraction) documented by positron emission tomography (PET). New imaging technology (perfusion magnetic resonance imaging, PET) for the assessment of cerebral blood flow will improve our ability to identify those asymptomatic patients with intracranial stenosis who are at the greatest risk of stroke and who thus may derive the greatest benefit from revascularization therapies.
Reduction of Infarct Size
This strategy for the management of cerebral ischemic disease focuses on ways to attenuate the adverse functional consequences of ischemia and reperfusion. Ischemia induces the formation of a multitude of adaptive cellular responses. Some of these responses can induce cell death. Among the more deleterious cell responses to ischemia are the formation of free radicals and the release of proteolytic enzymes and cytokines. There are many possible therapeutic targets for these pathways. Unfortunately, promising results obtained in the laboratory are not being replicated in the clinical arena, possibly because of differences in animal models and humans in anatomy and physiology that lead to differences in dose-response efficacy. At this point, it may be premature to draw conclusions about the influence of “brain protection” on the management of acute stroke.
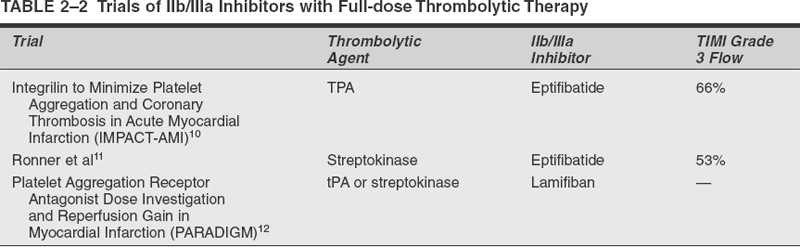
Stabilization of the Cerebrovascular Vessel Wall and Vessel Wall Interaction with the Bloodstream
This strategy focuses on maintaining long-term vascular stability to prevent new strokes. The development and progression of atherosclerotic plaque seems to be an inflammatory process. The use of statin drugs to lower low-density lipoprotein levels to subnormal levels promotes stabilization of coronary plaque. Some evidence exists that a similar effect is seen in the carotid arteries.19,20 Another important issue is the prevention of in-stent stenosis. The use of pharmacological coating for stents, P-radiation brachytherapy, and biodegradable stents to prevent in-stent stenosis is promising.
Management of Intracranial Arteriovenous Malformations
Endovascular embolization procedures have undergone dramatic evolution and improvement in recent years. The role of endovascular embolization in the treatment of AVMs is increasing as procedural safety and durability are demonstrated. The future of AVM embolization will involve a combination of advances in embolic materials, imaging technology, catheter designs, and embolization strategies.
Embolic Materials and Catheter Designs
A wide variety of embolic materials has been used for endovascular embolization of brain AVMs. The materials currently available include balloons, polyvinyl alcohol (PVA) particles, coils, and liquid embolic agents.
Balloons are effective for occlusion of the arteries that supply an AVM. Unfortunately, they have no effect on treating the AVM nidus. With the availability of embolic agents capable of penetrating the AVM nidus, the use of balloons became obsolete.
Polyvinyl alcohol particles are rarely used for AVM embolization in the United States since the approval of M-butyl cyanoacrylate (NBCA) for clinical use by the FDA in 2000. Currently, the use of PVA particles is reserved for presurgical embolization because the particles rarely provide permanent occlusion of the AVM.
Coils are used mainly as an adjunct to liquid embolic agents. They are placed in high-flow fistulas within the AVM. This strategy is very helpful to slow arterial flow to avoid premature penetration of liquid embolic agent in the venous portion of the AVM. Another application of coils is in the treatment of AVM flow-related aneurysms.
The use of liquid embolic agents is becoming the mainstay of endovascular strategies for the treatment of AVMs. Liquid agents currently used for AVM embolization include Trufill (NBCA; Cordis Neurovascular Inc., Miami Lakes, FL); Neuroacryl M21; Onyx, formerly called Embolyx E (Micro Therapeutics Inc., Irvine, CA), dissolved in dimethyl sulfoxide (DMSO), which is an organic solvent22, and ethyl alcohol.23 These materials are substantially more effective than nonliquid embolic agents for long-term occlusion of an AVM. The focus for the next generation of embolic materials will be improved control of penetration of the material into the nidus to avoid premature occlusion of inflow or outflow areas of the AVM. Stereotaxis, Inc. (St. Louis, MO) is developing the concept of delivery of liquid embolics guided by a magnetic field. Compatibility with current magnetic resonance imaging technology is a limitation of this magnetic field technology. Nonadhesion to delivery catheters and long-term occlusion rates of liquid embolic materials must also be demonstrated.
The over-the-wire and flow-guided microcatheters used for AVM embolization can be positioned optimally (close to the nidus) in most instances. Technical advances in microcatheters will probably also involve the use of an external magnetic field to help guide the microcatheter to a wedge position whenever conventional techniques fail.
Embolization Strategies
Embolization has a significant role in the multimodality treatment of brain AVMs by either enabling or facilitating subsequent microsurgical or radiosurgical treatment. In selected cases, endovascular therapy alone may lead to a total and permanent cure. Appropriately targeted embolization in otherwise untreatable AVMs represents a reasonable form of palliative treatment by either ameliorating the patient’s symptoms or reducing the potential risk of hemorrhage. The goal of the embolization should be determined before the procedure is begun. Embolization strategies such as distal coil protection, transvenous retrograde nidus sclerotherapy under controlled hypotensive anesthesia (TRENSH approach),24 cardiac standstill25, and general anesthesia may increase the chances of reaching the predetermined goal.
A commonly used strategy for AVMs with high-flow fistulas is to embolize the fistula with “liquid coils” before injecting the glue. The liquid coils remain on the fistula side and prevent the migration of glue to the draining vein.
According to the proposed TRENSH treatment approach,24 liquid embolic agent (sclerosant) is injected in a retrograde fashion through the venous side of the AVM after the induction of hypotensive anesthesia and with or without the aid of temporary balloon occlusion of the primary arterial feeder. Conceptually, the controlled hypotension leads to a reduction in arterial inflow such that the agent can permeate the nidus without filling arterial feeders and their branches to normal brain tissue. Hypothetically, the TRENCH approach may provide more complete permeation of the nidus while preventing ischemic complications associated with arterial delivery. Experimental studies directed at assessing the feasibility of this approach are justified before potential future clinical application.
Adenosine-induced cardiac pause maybe a viable method of partial flow arrest in the treatment of brain AVMs. The idea of flow arrest during injection of NBCA is to allow a more controlled, deep, and complete embolization.
Embolization of AVMs can be performed with or without general anesthesia. The use of general anesthesia is recommended for those cases in which the patient cannot cooperate with treatment, for instance, during pediatric cases.
Embolization of brain AVMs is a technical challenge that requires experience and skill on the part of the physician and further improvements in endovascular device technology. However, the endovascular surgeon can now choose from several strategies to achieve the goal of embolization, whether it is adjunctive, palliative, or curative.
Management of Intracranial Aneurysms
Endovascular techniques for the treatment of intracranial aneurysms have evolved in the past two decades. Serbinenko reported his experience with endovascular techniques in 1979,26 when he described embolization with intravascular balloons. Balloon embolization became the endovascular procedure of choice in the 1980s27–34 but was not ideally suited to selective occlusion of the aneurysm with preservation of the patency of the parent artery. Although it is sometimes possible to inflate a detachable balloon within the aneurysm while preserving flow through the parent artery, the disadvantage of this technique is that the size and shape of the balloon may not conform to that of the aneurysm, resulting in stretching of the aneurysm wall or incomplete filling of the aneurysm. The inability to customize a balloon to the configuration of an aneurysm led to the development of coil systems for aneurysm embolization.
Coil treatment permits conformation of the coil mass to the shape of the aneurysm, which represented a significant improvement over balloon embolization. Initially, pushable coils were used for treatment of cerebrovascular lesions.35,36 The major disadvantage of this system was the inability to remove coils that did not assume a favorable position or configuration within the aneurysm. This problem was addressed by the introduction of mechanically detachable37,38 and electrolytically detachable39–42 coils. First described by Guglielmi et al40,42 for the experimental treatment of cerebrovascular lesions, electrolytically detachable coils were favored by clinical interventionists because of concerns about the forces applied within the aneurysm for detachment of mechanically detachable coils. The design of the Guglielmi detachable coil (GDC; Boston Scientific/ Target, Fremont, CA) combines the advantages of soft compliant platinum, retrievability (a coil can be withdrawn, repositioned, or replaced before detachment), and atraumatic detachment. Subsequent to the approval of the GDC system by the FDA in 1995, there has been a trend toward the preferential use of endovascular therapy for the treatment of intracranial aneurysms. Early series reported use of GDC embolization solely for high-risk surgical cases (i.e., for patients of poor clinical grade or those with aneurysms deemed inoperable) .43–48 Since that time, however, many centers have begun using endovascular treatment as first-line therapy for intracranial aneurysms.
Evidence of the efficacy of endovascular treatment for patients with subarachnoid hemorrhage presenting in poor clinical condition52 prompted some centers to adopt a policy of reserving clip ligation for aneurysms in patients felt to be at high risk for complications from coil embolization. At these centers, the anatomy of the aneurysm is evaluated with consideration for the ability to fill the aneurysm with coils without compromising the parent artery lumen. Favorable aneurysm anatomy includes a dome-to-neck ratio of greater than 2 and a small aneurysm neck diameter, usually less than 5 mm.53 In addition, aneurysm location may be a factor involved in treatment decisions, with lower rates of technical success for coil embolization seen for middle cerebral artery aneurysms.54 The size of the aneurysm dome and neck influences both the ability to occlude the aneurysm with coils and the rate of subsequent regrowth of the coil-treated aneurysm.55 The presence of a large intraparenchymal hematoma with mass effect may favor a decision to perform open surgery to reduce intracranial pressure. Conversely, evidence of significant brain swelling without a mass lesion may increase the risk of surgical retraction, resulting in reduction in local blood flow and ischemic injury.56 The overall trend has been to consider endovascular treatment first, reserving surgical therapy only for aneurysms with unfavorable geometry or other clear surgical indications, such as intraparenchymal hematoma.
One of the major shortcomings of endovascular therapy, despite the widespread enthusiasm for its indications, was the inability to treat wide-necked aneurysms adequately. The propensity for coil herniation and parent vessel compromise made complete filling of the aneurysm nearly impossible and coil compaction or aneurysm regrowth a significant concern. Currently, techniques available for treating wide-necked aneurysms include balloon-assisted coil embolization, direct stenting or stent-assisted coiling, liquid embolic agents, and modification of the standard coiling technique (such as three dimensional coils).
Researchers have described the treatment of wide-necked aneurysms with stent-assisted coiling in experimental models.57,58 Hemodynamic evaluation in experimental aneurysm models demonstrated significant flow alterations within the aneurysm sac after stent placement across the ostium.59–63 Flow models may be used to quantify flow velocity and bulk flow within the aneurysm or the parent vessel. Studies using these models demonstrated that the greatest shear stress occurs at the distal aneurysm neck, a common site for aneurysm regrowth after coiling. Also, bulk flow may be reduced after parent artery stenting to as little as 5 % of the presenting baseline.63 Clinical application of these findings followed soon afterward.64–69 Successful deployment of a stent across the aneurysm ostium permits reconstruction of the parent vessel lumen and protects against coil prolapse. With this protection in place, coils may be packed more tightly within the aneurysm without fear of parent vessel compromise, thereby reducing the risk for residual aneurysm or aneurysm regrowth.
One concern about stent-assisted coiling is the fate of normal arterial branches after stents are placed across them. As most intracranial aneurysms occur at vessel bifurcations or branch points, a normal branch often arises near the aneurysm and may be difficult to avoid during stent positioning. In our experience, however, these branches do not become occluded after stenting. In a review of 10 patients with 10 branch arteries crossed by stents (to treat aneurysms in seven patients and intracranial stenosis in three patients) and angiographic follow-up ranging from 4 days to 35 months (average follow-up, 10 months), the branches remained angiographically patent, and no patient experienced an associated clinical ischemic episode.70 Flow demand for angiographically demonstrable branch arteries is sufficient to maintain patency after stents are placed across them. Another concern about stent-assisted coiling is the ability to deliver a stent to the target site through the tortuous cerebral vessels. Stent delivery devices tend to be rigid—more rigid than balloon catheters without stents, and much more rigid than microcatheters. Accordingly, it may be quite difficult to navigate a tortuous carotid siphon or cervical vertebral artery loop. In our series of intracranial stenting for all indications, we were able to successfully deploy a stent at the target site in 19 of 27 attempts.71 Unsuccessful attempts were related to the inability to access the proximal vessel from a highly tortuous arch (one case), arterial injury (two cases), and inability to navigate the stent into position (five cases). In the difficult navigation cases, the loop of artery that was proximal to the target lesion tended to have a small radius of curvature in comparison with those cases in which stent deployment was successful. Therefore, when we are considering treatment by intracranial stenting, we give more consideration to proximal vascular anatomy than to the presence of normal branches that may be compromised during stent positioning.
Perhaps the most significant shortcoming of endovascular treatment for intracranial aneurysms is the potential for regrowth or recurrence of an aneurysm after coiling. Large series have reported angiographic recurrence or growth of a remnant after coiling in 10 to 15% of cases.72–74 Although these same series report a low rate of recurrent hemorrhage for ruptured aneurysms treated with coiling and no cases of hemorrhage after coiling for unruptured aneurysms, hemorrhage remains the primary concern in cases of aneurysm regrowth. Current developments in the field of endovascular therapy for aneurysms are focusing on ways to reduce the incidence of aneurysm recurrence. Several design modifications primarily aimed at increasing the biological activity of GDCs have been proposed to stimulate the growth of endothelium over the aneurysm ostium at the coil surface. In a swine model, higher rates of aneurysm obliteration and re-endothelialization across the aneurysm ostium were achieved with collagen-coated platinum coils than with Dacron-fibered coils.75 Additional coil modifications have included ion implantation, protein coating, and coating with growth-factor-secreting tissue grafts.76,77 In vitro studies show increased endothelial cell-to-cell adhesion after exposure of coils to ion-implanted collagen coating than to nonimplanted collagen coating.78 If the processes of cell adhesion and tissue growth at the aneurysm-coil interface are enhanced by coil modifications, improved tissue healing across the aneurysm ostium would, in theory, reduce the risk of aneurysm recurrence or regrowth.
Another technique under consideration for prevention of aneurysm growth is the use of liquid polymers to fill aneurysms. The concept steins from the fact that aneurysm recurrence is reduced with a greater density of coil packing.79 In an in vitro study, Piotin et al79 demonstrated that dense coil packing represented filling of only 30 to 40% of the volume of the aneurysm dome. The implication is that the less residual volume left unfilled after treatment (i.e., the greater the density of packing), the lower the risk of aneurysm recurrence. Because coil filling is incomplete even in the most densely packed aneurysms, increased filling may require the use of a different medium. Animal studies have demonstrated excellent filling of aneurysms using cellulose acetate polymer (CAP).80,81 Subsequent clinical application was promising as well.82–84 Histological evaluation suggests that reendothelialization is more rapid and complete with CAP than with GDC.80 However, the same study suggests that complete filling of the aneurysm is more technically feasible with GDC than with CAP, underscoring the relative benefits of each. Another agent currently under clinical evaluation in the United States is the ethylene vinyl alcohol (EVAL) copolymer dissolved in dimethyl sulfoxide (DMSO). Clinical use of the ethylene vinyl alcohol mixture for aneurysm embolization was first reported by Nishi et al85; the parent vessel was occluded in two of three cases. Preservation of the parent artery requires temporary balloon occlusion during polymer delivery and precipitation, which may limit the use of polymers. The technology remains promising, however.
References
1. Tissue plasminogen activator for acute ischemic stroke. The National Institute of Neurological Disorders and Stroke rt-PA Stroke Study Group. N Engl J Med 1995;333:1581–1587
2. Weaver WD, Simes RJ, Betriu A, et al. Comparison of primary coronary angioplasty and intravenous thrombolytic therapy for acute myocardial infarction: a quantitative review. JAMA 1997;278: 2093–2098
3. del Zoppo GJ, Higashida RT, Furlan AJ, et al. PROACT: a phase II randomized trial of recombinant pro-urokinase by direct arterial delivery in acute middle cerebral artery stroke. PROACT Investigators. Prolyse in Acute Cerebral Thromboembolism. Stroke 1998;29:4–11
4. Furlan A, Higashida R, Wechsler L, et al. Intra-arterial prourokinase for acute ischemic stroke. The PROACT II study: a randomized controlled trial. Prolyse in Acute Cerebral Thromboembolism. JAMA 1999;282:2003–2011
5. Lewandowski CA, Frankel M, Tomsick TA, et al. Combined intravenous and intra-arterial r-TPA versus intra-arterial therapy of acute ischemic stroke: Emergency Management of Stroke (EMS) Bridging Trial. Stroke 1999;30:2598–2605
6. Suarez JI, Sunshine JL, Tarr R, et al. Predictors of clinical improvement, angiographic recanalization, and intracranial hemorrhage after intra-arterial thrombolysis for acute ischemic stroke. Stroke 1999;30:2094–2100
7. Ueda T, Sakaki S, Kumon Y, et al. Multivariable analysis of predictive factors related to outcome at 6 months after intra-arterial thrombolysis for acute ischemic stroke. Stroke 1999;30:2360–2365
8. Qureshi AI, Ali Z, Suri MF, et al. Intraarterial third-generation recombinant tissue plasminogen activator (reteplase) for acute ischemic stroke. Neurosurgery 2001;49:41-48:
9. White HD. Future of reperfusion therapy for acute myocardial infarction. Lancet 1999;354:695–697
10. Ohman EM, Kleiman NS, Gacioch G, et al. Combined accelerated tissue-plasminogen activator and platelet glycoprotein Ilb/IIIa integrin receptor blockade with Integrilin in acute myocardial infarction. Results of a randomized, placebo- controlled, dose-ranging trial. IMPACT-AMI Investigators. Circulation 1997;95: 846–854
11. Ronner E, Dykun Y, van den Brand MJ, et al. Platelet glycoprotein IIB/IIIA receptor antagonists. An asset for treatment of unstable coronary syndromes and coronary intervention. Eur Heart J 1998;19:160 8–1616
12. Combining thrombolysis with the platelet glycoprotein IIb/IIIa inhibitor lamifiban: results of the Platelet Aggregation Receptor Antagonist Dose Investigation and Reperfusion Gain in Myocardial Infarction (PARADIGM) trial. J Am Coll Cardiol 1998;32: 2003–2010
13. Trial of abciximab with and without low-dose reteplase for acute myocardial infarction. Strategies for Patency Enhancement in the Emergency Department (SPEED) Group. Circulation 2000;101: 2788–2794
14. Antman EM, Giugliano RP, Gibson CM, et al. Abciximab facilitates the rate and extent of thrombolysis: results of the thrombolysis in myocardial infarction (TIMI) 14 trial. The TIMI 14 Investigators. Circulation 1999;99:2720–2732
15. Grines CL, Cox DA, Stone GW, et al. Coronary angioplasty with or without stent implantation for acute myocardial infarction. Stent Primary Angioplasty in Myocardial Infarction Study Group. N Engl J Med 1999;341:1949–1956
16. McGuire DK, Hudson MP, East MA, et al. Highlights from the American Heart Association 72nd Scientific Sessions: November 6 to 10, 1999. Am Heart J 2000;139:359–370
17. Ross AM, Coyne KS, Reiner JS, et al. A randomized trial comparing primary angioplasty with a strategy of short-acting thrombolysis and immediate planned rescue angioplasty in acute myocardial infarction: the PACT trial. PACT investigators. Plasminogen-activator Angioplasty Compatibility Trial. J Am Coll Cardiol 1999;34: 1954–1962
18. Smith WS, Sung G, Starkman S, et al. Safety and efficacy of Mechanical embolectomy in acute ischemic stroke: results of the MERCI trial. Stroke 2005;36:1432–1438
19. Hebert PR, Gaziano JM, Chan KS, et al. Cholesterol lowering with statin drugs, risk of stroke, and total mortality. An overview of randomized trials. JAMA 1997;278:313–321
20. Rosenson RS. Biological basis for statin therapy in stroke prevention. Curr Opin Neurol 2000;13:57–62
21. Kerber CW, Wong W, Knox K, et al. Neuroacryl M: a new liquid embolic agent-Initial clinical results (abstr). J Neurosurg 2000;92:196
22. Jahan R, Murayama Y, Gobin YP, et al. Embolization of arteriovenous malformations with Onyx: clinicopathological experience in 23 patients. Neurosurgery 2001;48:984–997
23. Yakes WF, Krauth L, Ecklund J, et al. Ethanol endovascular management of brain arteriovenous malformations: initial results. Neurosurgery 1997;40:1145–1154
24. Massoud TF, Hademenos GJ. Transvenous retrograde nidus sclerotherapy under controlled hypotension (TRENSH): a newly proposed treatment for brain arteriovenous malformations—concepts and rationale. Neurosurgery 1999;45:351–365
25. Pile-Spellman J, Young WL, Joshi S, et al. Adenosine-induced cardiac pause for endovascular embolization of cerebral arteriovenous malformations: technical case report. Neurosurgery 1999;44:881–887
26. Serbinenko FA. Six hundred endovascular neurosurgical procedures in vascular pathology. A ten-year experience. Acta Neurochir Suppl (Wien) 1979;28:310–311
27. Berenstein A, Choi IS. Surgical neuroangiography of intracranial lesions. Radiol Clin North Am 1988;26:1143–1151
28. Higashida RT, Halbach VV, Barnwell SL, et al. Treatment of intracranial aneurysms with preservation of the parent vessel: results of percutaneous balloon embolization in 84 patients. AJNR Am J Neuroradiol 1990;11:633–640
29. Higashida RT, Halbach VV, Cahan LD, et al. Detachable balloon embolization therapy of posterior circulation intracranial aneurysms. J Neurosurg 1989;71:512–519
30. Higashida RT, Halbach VV, Dowd C, et al. Endovascular detachable balloon embolization therapy of cavernous carotid artery aneurysms: results in 87 cases. J Neurosurg 1990;72:857–863
31. Higashida RT, Halbach VV, Dowd CF, et al. Intracranial aneurysms: interventional neurovascular treatment with detachable balloons-results in 215 cases. Radiology 1991;178:663–670
32. Higashida RT, Halback VV, Dormandy B, et al. Endovascular treatment of intracranial aneurysms with a new silicone microballoon device: technical considerations and indications for therapy. Radiology 1990;174:687–691
33. Pevsner PH, Doppman JL. Therapeutic embolization with a microballoon catheter system. AJR Am J Roentgenol 1980;134: 949–958
34. Zeumer H, Bruckmann H, Adelt D, et al. Balloon embolization in the treatment of basilar aneurysms. Acta Neurochir (Wien) 1985;78:136–141
35. Casasco AE, Aymard A, Gobin YP, et al. Selective endovascular treatment of 71 intracranial aneurysms with platinum coils. J Neurosurg 1993;79:3–10
36. Hanner JS, Quisling RG, Mickle JP, et al. Gianturco coil embolization of vein of Galen aneurysms: technical aspects. Radiographics 1988;8:935–946
37. Kinoshita A, Ito M, Skakaguchi T, et al. Mechanical detachable coil as a therapeutic alternative for cerebral aneurysm. Neurol Res 1994;16:475–476
38. Marks MP, Chee H, Liddell RP, et al. A mechanically detachable coil for the treatment of aneurysms and occlusion of blood vessels. AJNR Am J Neuroradiol 1994;15:821–827
39. Guglielmi G. Embolization of intracranial aneurysms with detachable coils and electrothrombosis. In: Vinuela F, Halbach V, Dion J eds. Interventional Neuroradiology: Endovascular Therapy of the Central Nervous System. New York: Raven Press; 1992: 63–75
40. Guglielmi G, Vinuela F, Dion J, et al. Electrothrombosis of saccular aneurysms via endovascular approach. Part 2: Preliminary clinical experience. J Neurosurg 1991;75:8–14
41. Guglielmi G, Vinuela F, Duckwiler G, et al. Endovascular treatment of posterior circulation aneurysms by electrothrombosis using electrically detachable coils. J Neurosurg 1992;77:515–524
42. Guglielmi G, Vinuela F, Sepetka I, et al. Electrothrombosis of saccular aneurysms via endovascular approach. Part 1: Electrochemical basis, technique, and experimental results. J Neurosurg 1991;75:1–7
43. Byrne JV, Adams CB, Kerr RS, et al. Endosaccular treatment of inoperable intracranial aneurysms with platinum coils. Br J Neurosurg 1995;9:585–592
44. Byrne JV, Molyneux AJ, Brennan RP, et al. Embolisation of recently ruptured intracranial aneurysms. J Neurol Neurosurg Psychiatry 1995;59:616–620
45. Graves VB, Strother CM, Duff TA, et al. Early treatment of ruptured aneurysms with Guglielmi detachable coils: effect on subsequent bleeding. Neurosurgery 1995;37:640–648
46. Richling B, Gruber A, Bavinzski G, et al. GDC-system embolization for brain aneurysms—location and follow-up. Acta Neurochir (Wien) 1995;134:177–183
47. Standard SC, Guterman LR, Chavis TD, et al. Endovascular management of giant intracranial aneurysms. Clin Neurosurg 1995;42: 267–293
48. Vargas ME, Kupersmith MJ, Setton A, et al. Endovascular treatment of giant aneurysms which cause visual loss. Ophthalmology 1994;101:1091–1098
49. Gruber DP, Zimmerman GA, Tomsick TA, et al. A comparison between endovascular and surgical management of basilar artery apex aneurysms. J Neurosurg 1999;90:868–874
50. Leber KA, Klein GE, Trummer M, et al. Intracranial aneurysms: a review of endovascular and surgical treatment in 248 patients. Minim Invasive Neurosurg 1998;41:81–85
51. Steiger HJ, Medele R, Bruckmann H, et al. Interdisciplinary management results in 100 patients with ruptured and unruptured posterior circulation aneurysms. Acta Neurochir (Wien) 1999;141:359–367
52. Martin N. Decision-making for intracranial aneurysm treatment: when to select surgery and when to select endovascular therapy. J Stroke Cerebrovasc Dis 1997;6:253–257
53. Debrun GM, Aletich VA, Kehrli P, et al. Aneurysm geometry: an important criterion in selecting patients for Guglielmi detachable coiling. Neurol Med Chir (Tokyo) 1998;38:1–20
54. Regli L, Uske A, de Tribolet N. Endovascular coil placement compared with surgical clipping for the treatment of unruptured middle cerebral artery aneurysms: a consecutive series. J Neurosurg 1999;90:1025–1030
55. Vinuela F, Duckwiler G, Mawad M. Guglielmi detachable coil embolization of acute intracranial aneurysm: perioperative anatomical and clinical outcome in 403 patients. J Neurosurg 1997;86:475–482
56. Yundt KD, Grubb RL Jr, Diringer MN, et al. Cerebral hemodynamic and metabolic changes caused by brain retraction after aneurysmal subarachnoid hemorrhage. Neurosurgery 1997;40:442–451
57. Geremia G, Haklin M, Brennecke L. Embolization of experimentally created aneurysms with intravascular stent devices. AJNR Am J Neuroradiol 1994;15:1223–1231
58. Szikora I, Guterman LR, Wells KM, et al. Combined use of stents and coils to treat experimental wide-necked carotid aneurysms: preliminary results. AJNR Am J Neuroradiol 1994;15:1091–1102
59. Aenis M, Stancampiano AP, Wakhloo AK, et al. Modeling of flow in a straight stented and nonstented side wall aneurysm model. J Biomech Eng 1997;119:206–212
60. Wakhloo AK, Lanzino G, Lieber BB, et al. Stents for intracranial aneurysms: the beginning of a new endovascular era? Neurosurgery 1998:43:377–379
61. Lieber BB, Stancampiano AP, Wakhloo AK. Alteration of hemodynamics in aneurysm models by stenting: influence of stent porosity. Ann Biomed Eng 1997;25:460–469
62. Sekhon LHS, Morgan MK, Sorby W, et al. Combined endovascular stent implantation and endovascular coil placement for the treatment of a wide-necked vertebral artery aneurysm: technical case report. Neurosurgery 1998;43:380–384
63. Yu SC, Zhao JB. A steady flow analysis on the stented and non-stented sidewall aneurysm models. Med Eng Physiol 21:133-141, 1999
64. Higashida RT, Halbach W, Dowd CF, et al. Intracranial aneurysms. Evolution and future role of endovascular techniques. Neurosurg Clin N Am 1994;5:413–425
65. Higashida RT, Smith W, Gress D, et al. Intravascular stent and endovascular coil placement for a ruptured fusiform aneurysm of the basilar artery. Case report and review of the literature. J Neurosurg 1997;87:944–949
66. Lanzino G, Wakhloo AK, Fessler RD, et al. Efficacy and current limitations of intravascular stents for intracranial internal carotid, vertebral and basilar artery aneurysms. J Neurosurg 1999;91: 538–546
67. Lylyk P, Ceratto R, Hurvitz D, et al. Treatment of a vertebral dissecting aneurysm with stents and coils: technical case report. Neurosurgery 1998;43:385–388
68. Mericle RA, Lanzino G, Wakhloo AK, et al. Stenting and secondary coiling of intracranial internal carotid artery aneurysm: technical case report. Neurosurgery 1998;43:1229–1234
69. Wakhloo AK, Lanzino G, Lieber BB, et al. Stents for intracranial aneurysms: the beginning of a new era? Neurosurgery 1998;43: 377–379
70. Lopes D, Ringer A, Boulos A, et al. Fate of branch arteries after intracranial stenting. Neurosurgery 2003;52:1275–1279
71. Ringer A, Lopes D, Boulos A, et al. Current limitations to intracranial stent deployment (Abstract). J Neurosurg 2001;94:168A
72. Byrne JV, Sohn MJ, Molyneux AJ, et al. Five-year experience in using coil embolization for ruptured intracranial aneurysms: outcomes and incidence of late rebleeding. J Neurosurg 1999;90: 656–663
73. Cognard C, Weill A, Spelle L, et al. Long-term angiographic follow-up of 169 intracranial berry aneurysms occluded with detachable coils. Radiology 1999;212:348–356
74. Kuether TA, Nesbit GM, Barnwell SL. Clinical and angiographic outcomes, with treatment data, for patients with cerebral aneurysms treated with Guglielmi detachable coils: a single-center experience. Neurosurgery 1998;43:1016–1025
75. Dawson RC, Krisht AF, Barrow DL, et al. Treatment of experimental aneurysms using collagen-coated microcoils. Neurosurgery 1995;36:133–140
76. Murayama Y, Vinuela F, Suzuki Y, et al. Ion implantation and protein coating of detachable coils for endovascular treatment of cerebral aneurysms: concepts and preliminary results in swine models. Neurosurgery 1997;40:1233–1244
77. Nakagawa K, Touho H, Morisako T, et al. Long-term follow-up study of unruptured vertebral artery dissection: clinical outcomes and serial angiographic findings. J Neurosurg 2000;93:19–25
78. Murayama Y, Suzuki Y, Vinuela F, et al. Development of a biologically active Guglielmi detachable coil for the treatment of cerebral aneurysms. Part I: in vitro study. AJNR Am J Neuroradiol 1999;20: 1986–1991
79. Piotin M, Mandai S, Murphy KJ, et al. Dense packing of cerebral aneurysms: an in vitro study with detachable platinum coils. AJNR Am J Neuroradiol 2000;21:757–760
80. Macdonald RL, Mojtahedi S, Johns L, et al. Randomized comparison of Guglielmi detachable coils and cellulose acetate polymer for treatment of aneurysms in dogs. Stroke 1998;29:478–486
81. Sugiu K, Kinugasa K, Mandai S, et al. Direct thrombosis of experimental aneurysms with cellulose acetate polymer (CAP): technical aspects, angiographic follow up, and histological study. J Neurosurg 1995;83:531–538
82. Kinugasa K, Mandai S, Terai Y, et al. Direct thrombosis of aneurysms with cellulose acetate polymer. Part II: Preliminary clinical experience. J Neurosurg 1992;77:501–507
83. Kinugasa K, Mandai S, Tsuchida S, et al. Direct thrombosis of a pseudoaneurysm after obliteration of a carotid-cavernous fistula with cellulose acetate polymer: technical case report. Neurosurgery 1994;35:755–760
84. Kinugasa K, Mandai S, Tsuchida S, et al. Cellulose acetate polymer thrombosis for the emergency treatment of aneurysms: angiographic findings, clinical experience, and histopathological study. Neurosurgery 1994;34:694–701
85. Nishi S, Taki W, Nakahara I, et al. Embolization of cerebral aneurysms with a liquid embolus, EVAL mixture: report of three cases. Acta Neurochir (Wien) 1996;138:294–300
< div class='tao-gold-member'>
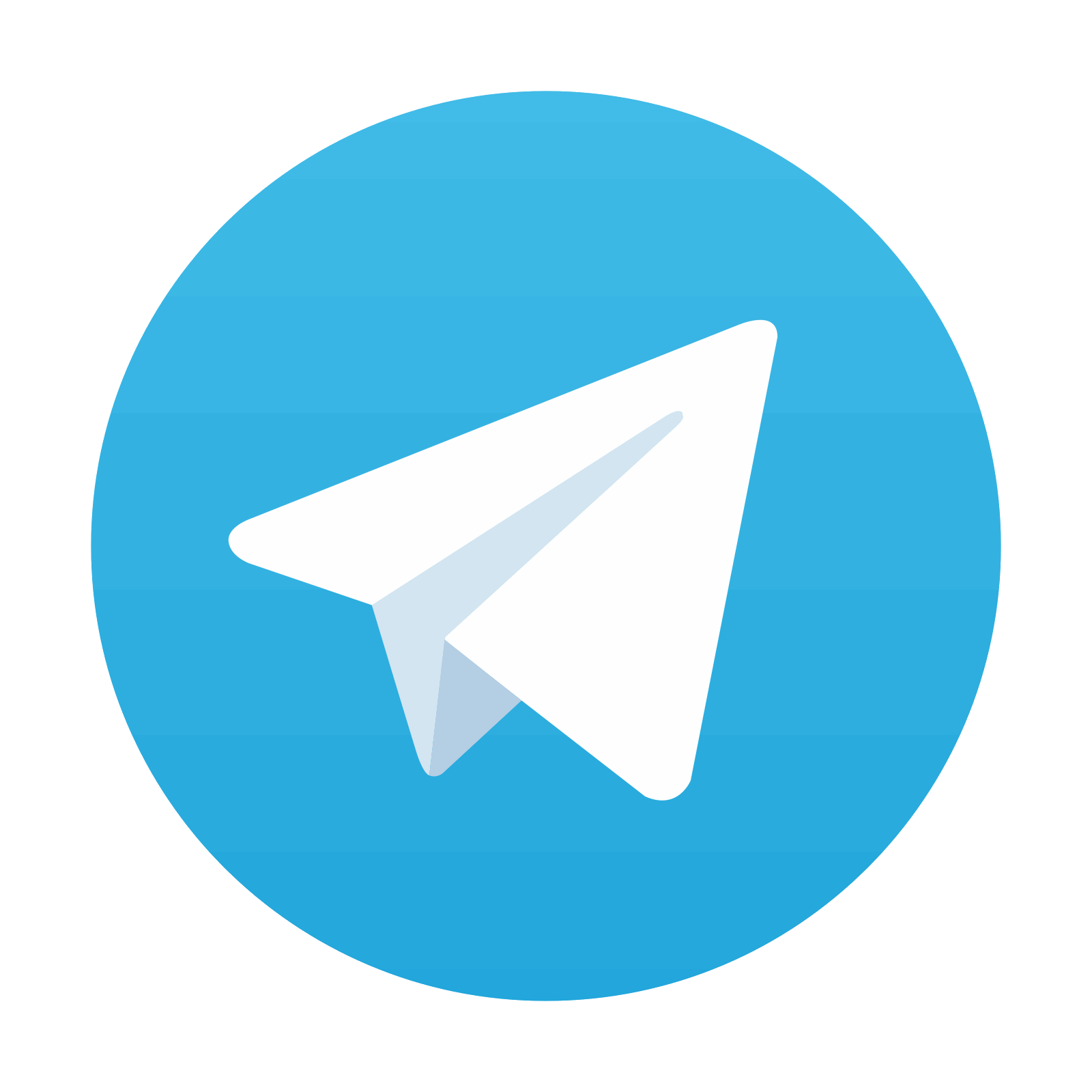