Introduction
Cranial irradiation is used in settings ranging from definitive treatment of primary brain and head and neck cancers to palliation of metastatic disease and prophylaxis, as a single modality or in combination with surgery or systemic therapy. Common side-effect considerations include neurocognitive and inflammatory effects as well as damage to critical brain structures, which are discussed elsewhere in this text (see Chapter 24 for a discussion of radiation necrosis and Chapter 25 for neurocognitive dysfunction). Here, we provided a case-based review of rare complications of cranial irradiation. A comprehensive understanding of potential complications of radiation therapy is necessary for optimal multidisciplinary management of oncology patients. Generally, complications are related to the disease site, radiation field, radiation dose, age at time of treatment, receipt of combination therapies, and time since treatment completion.
Clinical cases
Case . A 67-year-old man with a 25 pack-year smoking history and squamous cell carcinoma of the right base of the tongue treated with a cumulative dose of 70 Gy using conventional fractionation with concurrent carboplatin. One-year posttreatment, he presented with numbness and tingling sensations in the lower extremities, elicited with neck movements, and dull, intermittent cervical neck pain exacerbated by palpation. Review of systems was otherwise negative. Neurological examination was otherwise unremarkable, and cranial nerves II–XII were intact. Imaging showed no evidence of local disease recurrence. Magnetic resonance imaging (MRI) of the spine showed mildly T2 hyperintense signal within a prominent cord at the C3–C7 levels ( Fig. 26.1 ). The patient’s symptoms and imaging are consistent with radiation myelopathy with intermittent Lhermitte syndrome. On review of radiation treatment records, the maximum dose to the spinal cord was 37 Gy. Treatment with hyperbaric oxygen was initiated and improved radiation-related xerostomia; however, radiation myelitis did not improve. Treatment with steroids decreased severity of the lower extremity nerve pain after 2 weeks. The patient now continues on long-term pentoxifylline and vitamin E.

Teaching Points . Radiation myelitis is the most common of the rare complications of spinal cord irradiation and can present as a transient early or irreversible late reaction. Other rare complications include paralysis secondary to ischemia, hemorrhage, and lower motor neuron syndrome.
Early radiation-induced myelopathy . Early radiation-induced myelopathy typically develops within 2–6 months following spinal irradiation and usually spontaneously resolves over 3–6 months without intervention. The characteristic clinical presentation is a positive Lhermitte’s sign, an electric shock-like sensation radiating to the extremities or trunk upon flexion of the neck. The incidence is estimated to be between 3–15% among patients receiving radiation to the head, neck, and upper respiratory tract. Diagnosis is based on history, physical examination, and evaluation for alternative causes of myelopathy. The differential includes compressive disease (metastatic tumor, cervical spondylosis), demyelinating disease (multiple sclerosis), chemotherapy toxicity (platinum agents and taxanes), vitamin B 6 or B 12 deficiency, rheumatoid disease, and trauma, among other causes of transverse myelitis. Positron emission tomography demonstrating increased spinal cord metabolic uptake in the setting of Lhermitte’s sign has also been described but is not necessary for diagnosis. The mechanism of injury is attributed to transient damage to myelin-producing oligodendroglial cells in the irradiated field resulting in demyelination of the posterior columns of the spinal cord leading to hyperexcitability. Early radiation-induced myelopathy is a self-limited condition that does not require treatment. Neuropathy and muscle spasm can be symptomatically managed with medications such as gabapentin or carbamazepine.
Late radiation-induced myelopathy . Late radiation-induced myelopathy is chronic and typically, but not always, progressive and irreversible. Onset ranges between 6–30 months posttreatment, with one series reporting a mean latency of 18.5 months following a single course of irradiation and 11.4 months following re-irradiation. Symptoms are variable, ranging from minor to major sensorimotor deficits or paraplegia. Late radiation-induced myelopathy is a diagnosis of exclusion based on history, physical examination, and imaging. The differential is similar to that of early radiation-induced myelopathy with special attention to the evaluation of new metastases and disease progression. On MRI, radiation-induced myelopathy is typically characterized by intramedullary T1 hypointensity, possible focal contrast enhancement, and T2 hyperintensity ( Fig. 26.1 ). , Pathogenesis is multifactorial and predominantly attributed to white matter injury of the spinal cord as well as vascular changes. White matter injury includes myelin breakdown, nerve fiber degeneration and necrosis in the setting of damage to neurons and supporting cells including oligodendrocytes, astrocytes, and immune microglia cells. Vasculature-related changes include altered vascularity, telangiectasias, hyaline degeneration, edema, fibrosis, inflammation, degeneration, impaired blood flow, and hemorrhage. Treatment with radiosensitizing agents may increase risk of radiation toxicity by potentiating these mechanisms.
Risk of damage to the spinal cord can be mitigated with consideration of radiation dose, volume, and fractionation. One radiobiologic indicator of tissue sensitivity to radiation dose per fraction is the alpha/beta ratio. The spinal cord has a low alpha/beta ratio indicating high sensitivity to dose per fraction. Based on the Quantitative Analysis of Normal Tissue Effects in the Clinic (QUANTEC), a benchmark set of reviews on normal tissue toxicity secondary to radiation, the risk of radiation-induced myelopathy is less than 1% and less than 10% for total doses of 54 Gy and 61 Gy, respectively, using conventional radiotherapy fractionation of 1.8–2 Gy/fraction to the full-thickness cord.
More recently, where clinically appropriate, alternative fractionation schemes have been adopted including hypofractionation, delivery of radiation over a shorter period of days or weeks compared with standard radiation therapy, and stereotactic body radiation therapy, which is the use of high doses of radiation given in a highly precise and conformal manner to a target volume over a small number of fractions. QUANTEC analysis shows that when the maximum spinal cord dose is limited to the equivalent of 13 Gy in one fraction or 20 Gy in three fractions, the risk of myelopathy is <1%.
Re-irradiation is considered for both palliation and definitive management of local disease progression or new disease sites. Treatment may encompass the entirety of the previously treated spinal cord or only a portion of this volume. Given the complexity of these cases, careful consideration is given to the prior radiation plan including spinal cord volume treated, dose and fractionation received, and time interval since treatment completion. Per QUANTEC, when the cumulative dose from both initial and repeat irradiation to the cord is less than 60 Gy in 2 Gy equivalents, no cases of myelopathy were reported. Preclinical and metaanalysis data further suggest partial recovery of subclinical damage to the cord beginning at 6 months and increasing over 2 years posttreatment, and thus a minimum time interval of 6 months from initial to repeat irradiation is recommended. ,
Treatment of radiation myelitis focuses on addressing the suspected mechanisms of injury and supportive care as clini cal data are limited. High-dose glucocorticoids are often empirically prescribed to reduce inflammation and edema. In a 14-patient, double-blind, placebo-controlled, randomized clinical trial of symptomatic radiation necrosis of the brain, the monoclonal antibody against vascular endothelial growth factor, bevacizumab, led to radiographic and neurologic symptom improvement for all patients in the intervention arm, and case reports have suggested some improvement in radiation myelopathy with bevacizumab. , Hyperbaric oxygen is considered based on application to treating soft tissue and osteoradionecrosis; however, evidence for treating radiation myelitis is limited to case reports and preclinical data. , Anticoagulation with heparin and warfarin was evaluated in a small study of 11 patients with late radiation-induced nervous system injuries including cerebral radionecrosis and myelopathy unresponsive to glucocorticoids, which showed some recovery of function in over half of the patients, presumably due to impact on small vessel endothelial injury. The combination of vitamin E and pentoxifylline, a phosphodiesterase inhibitor that reduces inflammation and also decreases erythrocyte resistance to deformation and blood viscosity, have been used in management of radiation-induced fibrosis and could be considered for radiation myelitis. ,
Lower motor neuron syndrome is a delayed radiation complication with time to onset over a period of years following radiation to the lower spinal cord and cauda equina, characterized by progressive lower extremity weakness with minimal impairment of sensory, bowel, and bladder function. The etiology is controversial given the lack of radiation dose–effect relationship. Proposed mechanisms include preceding viral infection and radiculopathy secondary to vasculopathy of proximal spinal roots without significant damage to the lower motor neurons in the cord. ,
Spinal cord hemorrhage, telangiectasia, and cavernous malformations can develop following radiation, though reports are exceedingly rare. Potential complications include symptomatic infarct or compression and subsequent effects. ,
Case . A 31-year-old woman with history of medulloblastoma at age 4 was treated with combination chemotherapy and radiation to a cumulative dose of 54 Gy using conventional fractionation and remains in remission. Twenty-six years posttreatment, she presented with 2 weeks of recurrent migraines and loss of her right visual field. With the exception of visual field cut, neurologic examination was nonfocal. On MRI, post-contrast T1-weighted images showed enhancement of the cerebral gyri sparing the white matter in the occipital lobe. On review of prior records, the region of enhancement corresponded with the prior radiation field. Given symptoms and MRI findings consistent with stroke-like migraine attacks after radiation therapy (SMART) syndrome, no further diagnostics such as biopsy were indicated. Symptoms were managed conservatively with avoidance of headache triggers, a brief course of steroids, and verapamil for migraine prophylaxis. Migraines resolved 2 weeks later, and visual field was restored. The patient has now been 1 year without recurrent episodes.
Teaching Points . SMART syndrome is a delayed complication of brain irradiation characterized by recurrent episodes of complicated migraines. This is a rare entity with approximately 40 reported cases, although it is likely underdiagnosed. , Time to onset in these cases ranges from 1–35 years, with a median of 14 years post-irradiation. Common symptoms include headache, seizure, hemianopsia, hemiparesis, and aphasia. Symptoms are often recurrent over a period of hours to weeks but generally considered reversible, although incomplete neurologic recovery has been reported. ,
Differential diagnosis . The differential includes tumor recurrence, infarct, vasculitis, mitochondrial encephalomyelopathy with lactic acidosis and stroke (MELAS), posterior reversible encephalopathy (PRES), meningoencephalitis, status epilepticus, familial/sporadic hemiplegic migraine, radiation vasculopathy, cerebral autosomal dominant arteriopathy with subcortical infarcts and leukoencephalopathy (CADASIL), and toxic-metabolic derangements. Proposed diagnostic criteria by Black et al. include (1) a remote history of cranial irradiation with absence of residual or recurrent tumor; (2) prolonged characteristic symptoms of complex migraine, seizures, and/or stroke-like symptoms referable to a unilateral cortical region; (3) characteristic brain MRI findings; and (4) exclusion of alternative etiologies.
Imaging findings . Post-contrast T1-weighted MRI may show unilateral cortical gadolinium enhancement of the cerebral gyri sparing the white matter within the prior radiation field, usually in the temporal, parietal, or occipital lobes. Transient T2 and fluid attenuated inversion recovery (FLAIR) hyperintensity, as well as susceptibility-weighted imaging (SWI) with numerous hypointensities within the periventricular white matter attributed to radiation-induced cavernous hemangiomas, have been described. These characteristic MRI findings appear 2–7 days following symptom onset. , , In addition to symptom management, recognition of this entity is critical for preventing unnecessary invasive diagnostics such as brain biopsy.
Pathogenesis . The pathogenesis is poorly understood. Most cases are associated with a radiation dose of 50 Gy or higher, but reported doses have ranged from 15–64 Gy with highly variable treatment volumes and concurrent systemic therapies. , It has been hypothesized that SMART could result from radiation-induced injury to the vasculature or trigeminovascular system, lowered threshold for cortical- spreading depression and endothelial dysfunction resulting in characteristic migraines, cortical enhancement, and seizures. Notably, surgically-induced SMART syndrome has also been reported.
Treatment . Treatment for SMART is limited to conservative medical management given the dearth of clinical cases and data. Similar to management of complex migraines, avoidance of headache triggers is encouraged, and antiepileptics or a brief course of steroids may be considered. Limited case reports suggest aspirin and verapamil, a calcium channel blocker used for migraine and cluster headache prophylaxis and thought to prevent vasospasm, may reduce the frequency and severity of SMART syndrome episodes. ,
Case . A 30-year-old woman with history of right sinus and orbit rhabdomyosarcoma diagnosed at age 3 was treated with chemotherapy and radiation therapy to 59.8 Gy using conventional fractionation. At age 7, she presented with growth failure and precocious puberty. At age 9, she developed temperature dysregulation, difficulty sleeping, and excessive daytime somnolence. Examination was notable for poor dentition and impaired growth of facial bones. Imaging for chronic rhinosinusitis showed bilateral sphenoid sinus stenosis. The patient was jointly followed by pediatrics, radiation oncology, endocrinology, and neurology. She was diagnosed with panhypopituitarism and dysfunctional circadian drive but did not have adrenal insufficiency. Collectively, her conditions indicated hypothalamic and pituitary dysfunction. She was treated with growth hormone replacement and started on leuprolide followed by oral contraceptives for precocious puberty and menstrual regulation. The patient also takes levothyroxine for hypothyroidism. She was counseled on sleep hygiene and takes melatonin as needed.
Teaching Points . Dysfunction of the hypothalamus most often involves the hypothalamic-pituitary axis but can also be limited to the hypothalamus alone. The hypothalamus is located in the ventral brain above the pituitary gland and may fall within the radiation field during cranial irradiation for numerous indications including prophylactic irradiation (≤30 Gy), total-body irradiation (10–14 Gy) and treatment of primary brain, nasopharyngeal, sinonasal, skull base, and metastatic lesions (≤60 Gy). The hypothalamus releases hormones that act on the pituitary gland, forming the hypothalamic-pituitary axis to regulate multiple endocrine systems and is also involved in nonendocrine functions of temperature, appetite, and autonomic nervous system regulation. Disruptions of either the hypothalamic or pituitary component of the axis can lead to endocrine dysfunction, both of which require care coordination with endocrinology, with a focus on managing hormonal derangements.
Neuroendocrine dysfunction as a result of hypothalamic-pituitary irradiation is common among both children and adults. Hormones released by the hypothalamus include growth hormone–releasing hormone (GHRH), gonadotropin-releasing hormone (GnRH), thyrotropin-releasing hormone (TRH), corticotropin-releasing hormone (CRH), somatostatin, and dopamine. Each of the corresponding hormone axes have variable sensitivity to radiation dose, with the growth hormone (GH) axis being the most radiosensitive, followed by the thyroid hormone, adrenocorticotropic hormone (ACTH), and gonadotropin axes ( Table 26.1 ).
Condition | Dose | Presentation | Diagnostics |
---|---|---|---|
GH deficiency | ≥18 Gy | Children: Growth delay Adults: Variable cognitive, cardiovascular, metabolic abnormalities | IGF-I, ITT, glucagon, clonidine |
Central precocious puberty | ≥18 Gy | Early onset of puberty | FSH, LH, estradiol (female), testosterone (male) |
FSH/LH deficiency | ≥30 Gy | Delayed puberty and linear growth in children | FSH, LH, estradiol (female), testosterone (male), GnRH |
TSH deficiency | ≥30 Gy | Fatigue, weight gain, cold intolerance, brittle hair and constipation | TSH, FT4, TRH |
ACTH deficiency | ≥30 Gy | Weight loss, anorexia, nausea, vomiting | Cortisol, ITT, Synacthen |
Hyperprolactinemia | ≥40–50 Gy | Pubertal delay in children, impotence in men, galactorrhea in women | Prolactin level |
Growth hormone–releasing hormone (GHRH) . GHRH stimulates the anterior pituitary gland to secrete GH. Time to onset among children is influenced by dose and estimated to be 60 months, 36 months, and 12 months for radiation doses of 15–20 Gy, 25–36 Gy and >60 Gy, respectively. , A longitudinal study of adults receiving radiation for primary nonpituitary brain tumors reported dose-dependent GH deficiency among 32% of patients. Similarly, a meta-analysis showed that among adults receiving radiation for nonpituitary lesions, the median prevalence of GH deficiency was 45%. GH deficiency in children causes growth impairment, and, in adults, severe deficiency has been associated with cognitive, cardiovascular, neuromuscular, metabolic, and bony abnormalities. Diagnosis is based on low GH levels following one of various GH secretion-stimulating tests, of which the insulin tolerance test remains the gold standard for radiation-induced GH deficiency. Children treated with cranial irradiation are recommended to undergo routine physical examinations every 6 months until growth is complete and, if poorly growing, undergo evaluation with assessment of nutritional status, thyroid studies, and radiographs for bone age. Referral to pediatric endocrinology is indicated for further evaluation and GH replacement. Due to the theoretical risk of GH replacement stimulating cancer recurrence, therapy is typically avoided for 2 years following treatment completion. Adults with severe symptomatic GH deficiency may be selectively considered for GH replacement by endocrinology.
Gonadotropin-releasing hormone (GnRH) . GnRH regulates release of follicle-stimulating hormone (FSH) and luteinizing hormone (LH) from the anterior pituitary, thereby controlling sex-specific hormone secretion and onset of puberty. Central gonadotropin deficiency and hypogonadism are late onset and can occur following doses >30 Gy at a cumulative incidence of 20–60% among children and adults, with risk of dysfunction increasing with dose. , In children, doses causing severe GnRH deficiency lead to concomitant GH deficiency. This combined deficiency can lead to delayed puberty and delayed linear growth in children. Conversely, radiation doses ≥18 Gy can cause central precocious puberty, with higher risk in girls and children receiving radiation treatment at a younger age. In children, there is an active inhibition of GnRH secretion prepuberty. Puberty is initiated with a sustained increase in pulsatile release of GnRH from the hypothalamus. It is hypothesized that doses of 15–50 Gy injure inhibitory neurons, leading to increased GnRH secretion. Children must be carefully followed, as precocious puberty can mask GH deficiency and lead to decreased height potential due to accelerated epiphyseal closure. In adults, hypogonadism can present as fatigue, decreased sexual function and drive, and infertility. Gonadotropin deficiency is diagnosed based on history and serum levels of estrogen/testosterone, FSH, or LH. Primary pituitary versus hypothalamic hypogonadism are differentiated based on response to GnRH stimulation. Hypogonadism may be treated with hormone replacement therapy consisting of GnRH for hypothalamic hypogonadism and with replacement of appropriate sex-specific hormones for pituitary etiology.
Thyrotropin-releasing hormone (TRH) . TRH stimulates the release of thyroid-stimulating hormone (TSH) and prolactin from the anterior pituitary gland. Thyroid hormone deficiency from hypothalamic-pituitary irradiation is dose dependent, with 5-year hormone deficiency rates of 4%, 25%, and 43% from median hypothalamic and pituitary doses of ≤20 Gy, 20–40 Gy, and ≥40 Gy, respectively. In addition, the thyroid itself is highly radiation-sensitive, and children undergoing craniospinal irradiation or adults receiving head and neck irradiation are at high risk of primary hypothyroidism. , Patients may present with fatigue, weight gain, cold intolerance, brittle hair, and constipation. Testing for annual free T4 and TSH is recommended. Deficiency can be corrected with administration of levothyroxine.
Corticotropin-releasing hormone (CRH) . CRH stimulates the release of ACTH from the anterior pituitary. This axis is relatively radioresistant, as ACTH deficiency occurs in less than 5% of patients receiving cranial irradiation with hypothalamic-pituitary doses of <40 Gy. , , ACTH deficiency is a late onset side-effect that is typically subclinical but may present with weight loss, anorexia, nausea, and vomiting; hypoglycemia and dilutional hyponatremia are unlikely. The insulin tolerance test, blood cortisol level measurement, and the cortisol stimulation test are commonly employed for diagnosis. Deficiency is treated with cortisol hormone replacement therapy.
Prolactin . Prolactin is released from the pituitary gland and primarily promotes lactation. Dopamine output from the hypothalamus inhibits release of prolactin. Although up to 72% of patients may have an elevated prolactin level following radiation to the hypothalamic-pituitary axis, hyperprolactinemia manifesting as pubertal delay in children, impotence in men, or galactorrhea in women is rare. , Symptomatic hyperprolactinemia may be managed with the dopamine agonists cabergoline or bromocriptine.
Data are limited on radiation-induced nonendocrine hypothalamic dysfunction. However, similar to the case presented here, it is likely that hypothalamic irradiation can lead to disruptions in thermal homeostasis, autonomic system regulation and maintenance of circadian rhythm. In addition, hypothalamic obesity is known to result from hypothalamic tumors or injury to the hypothalamic region (e.g., surgery or radiotherapy) and is seen in 30–75% of patients treated for craniopharyngioma. This form of obesity results from impaired leptin signaling, thus simulating a state of starvation where energy expenditure is reduced and adipogenesis and insulin secretion are increased. As lifestyle and pharmacologic interventions are minimally effective, the best strategy is to limit hypothalamic damage upfront, for example, by treating craniopharyngiomas with more conservative surgery followed by conformal radiotherapy as opposed to gross total resection. ,
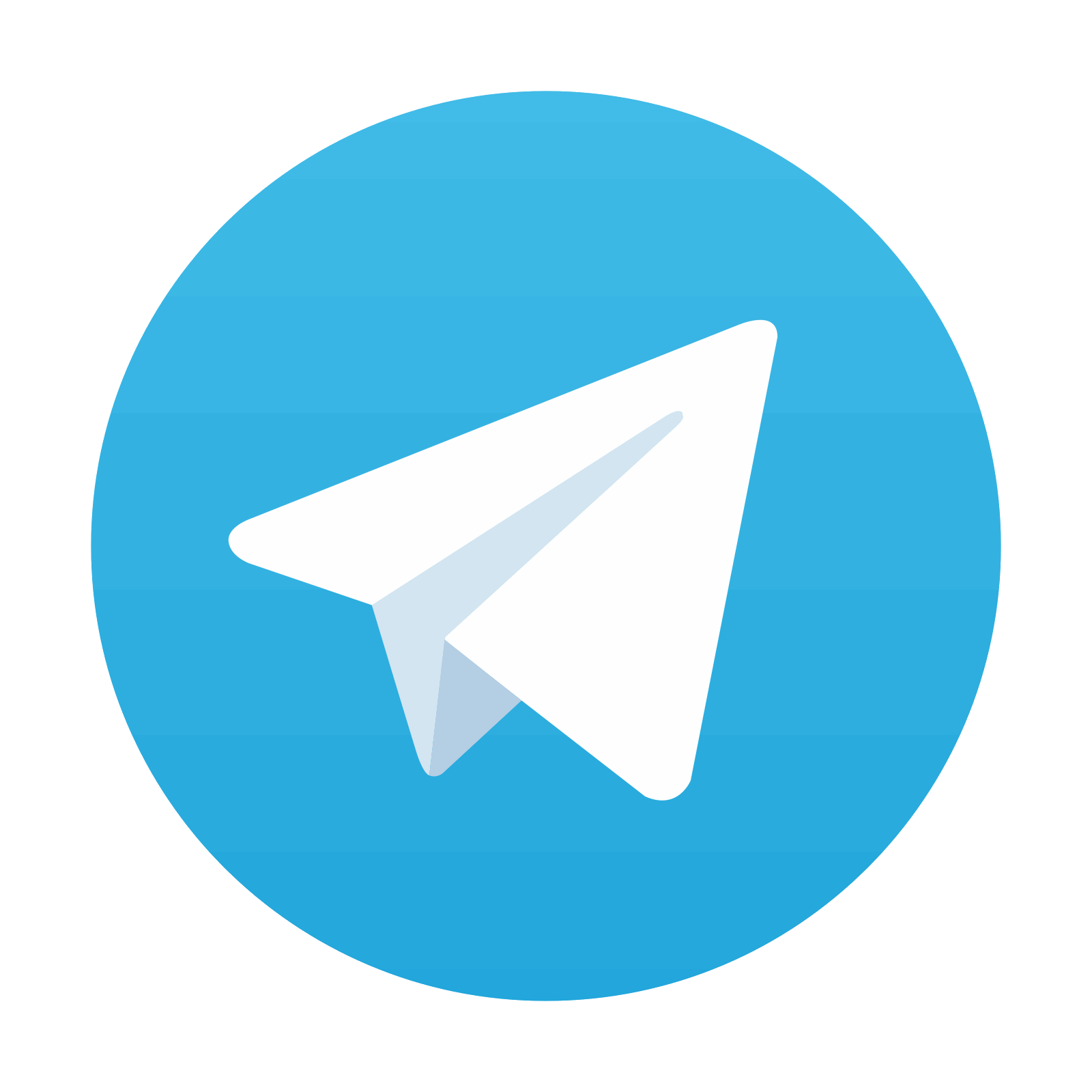
Stay updated, free articles. Join our Telegram channel

Full access? Get Clinical Tree
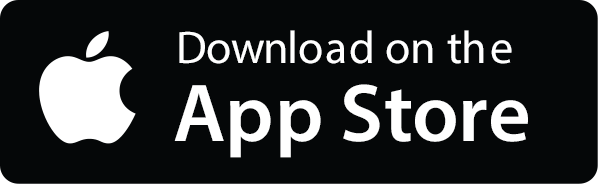
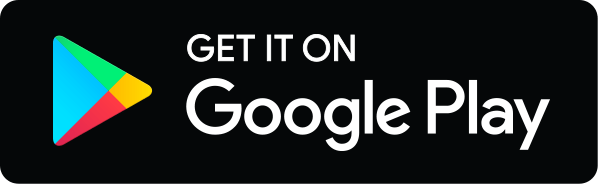
