Fig. 9.1
Functional plasticity of T cells and associated genetic factors may mediate autoimmunity in autism. Naïve CD4+ T cells become differentiated into four different effector cell subtypes, which show functional activities promoting or preventing autoimmunity . Reported altered levels of specific cell types, transcription factors, and secreted cytokines are shown. The increase in Th2 function and decrease in Th1 and Treg function, promote an immune activation leading to autoimmunity. In addition, variants of genes associated with autoimmunity have been identified in ASD, particularly haplotypes of the human leukocyte antigen (HLA-DR4/HLA-DR13) and mutations in no HLA genes related to innate immunity (C3a–C4a genes) and signaling pathways like PTEN
Th1 cells are induced by IL-12 and they express T-bet, a Th1 transcription factor. They produce IFN-γ as their main proinflammatory cytokine, which is responsible for some pathogenic effects observed in both autoimmune and inflammatory disorders (Romagnani et al. 2000; Romagnani 2000) . Th2 helper cells are induced by IL-4 and they express GATA3 (Mjosberg et al. 2012; Hoyler et al. 2012) . This cell type produces IL-5, IL-9, IL-10, and IL-13 cytokines, all associated with the humoral immunity .
The balance of Th1/Th2 cells is tightly controlled, and an overactivity of either of these cell types results in immune disease. There is evidence of a shift from Th1 to Th2 cells in autism, observed as decreased production of IL-2 and IFN-γ and an increase in IL-4 levels (van Gent et al. 1997; Gupta et al. 1998) , Fig. 9.1. However, an increase of IL-12 levels in the plasma of autistic patients has been reported (Singh 1996) . Authors proposed that this abnormal production of IL-12 could be a compensatory mechanism in ASD individuals, but this hypothesis has not been further tested. Moreover, a more recent report showed that cells from ASD patients express more Th1 and Th2 cytokines in absence of a compensatory increase of IL-10, a cytokine that modulates the inflammatory response (Molloy et al. 2006) , reinforcing again the immune deregulation observed in these children.
The expression of GATA3, a transcription factor that is needed for Th2 differentiation, has been associated with autism. In a neuron-like PC-12 cell model, GATA3 expression levels are increased after their treatment with valproate, thalidomide, or alcohol, three teratogens known to cause ASD (Rout and Clausen 2009) . As GATA3 plays a key role in the differentiation of some neuronal types (like serotonergic neurons; (van Doorninck et al. 1999)) , it has been hypothesized that an excessive differentiation of neurons expressing GATA3 could cause anomalies in the development of brain resulting in autistic symptoms. So, GATA3 could represent a molecular link between immune and neuronal dysfunction in ASD.
Naturally occurring regulatory T (nTreg) cells are a central component of peripheral tolerance as they suppress the adaptive T cell response, show an anti-inflammatory role, and prevent autoimmunity . These cells were originally defined by high CD25 expression and primarily characterized by the expression of the transcription factor Foxp3 (forkhead/winged helix transcription factor). After differentiation, they produce TGF-β. Stable expression of Foxp3 is essential for the development, homeostasis, and suppressive function of nTreg cells (Sun et al. 2007; Liu et al. 2010; Ueda et al. 2012) . At least one report has shown a decrease in the number of Treg cells in children with autism (Mostafa et al. 2010) , Fig. 9.1. In addition, plasma levels of TGF-β were overall decreased in autistic individuals and they showed an inverse correlation with behavioral scores (Ashwood et al. 2008b; Okada et al. 2007) , indicating that regulatory T cell responses are decreased in ASD and that the lack of suppressor activity of the immune system could be involved in the expression of autistic behavior.
Th17 cells, a newly defined lineage of T helper cells, differ from other T helper cells subsets both in their gene expression and regulatory function (Ueda et al. 2012; Dong 2008) . These cells are remarked by the production of the hallmark cytokines IL-17 and IL-17F. The IL-17 cytokine family is composed by 6 cytokines, IL-17 A–F, and besides its role for Th17 differentiation, they mediate inflammatory functions and autoimmunity . Stimulation of T cells by TGF-β together with IL-6 or IL-21 promotes Th17 development (Harrington et al. 2006) . IL-17 is augmented in autistic children (Suzuki et al. 2011) , Fig. 9.1.
The nature of Foxp3 expression in induced Treg (iTreg) cells in vitro (Ueda et al. 2012) combined with the potential of Foxp3+ nTreg cells to differentiate into Th17 cells in the presence of IL-6 and TGF-β, suggested that Foxp3-expressing Treg cell subsets manifest functional adaptation under certain conditions (Ayyoub et al. 2012; Ueda et al. 2012; Bettelli et al. 2007; Maloy 2008) . Although there is not a complete understanding on the signaling mechanism inducing Th17 differentiation, studies have shown that the key transcription factors in determining the differentiation of this T cell lineage are retinoid-related orphan receptor γt (RORγt) and RORα which can be induced by the combination of IL-6 and TGF-β (Ueda et al. 2012; Yurchenko et al. 2012) . Thymic or peripheral Foxp3+ nTreg cells may also manifest prominent functional plasticity and readily reprogram into Th1 and Th17 effectors’ cells, particularly in the gut microenvironment or sites of parasitic infection (Ahern et al. 2008) . As it will be discussed later, this could lead to the chronic gastrointestinal inflammation observed in ASD.
9.4 Other Genes Regulating the Immune System in ASD
Family studies have provided some evidences for a higher genetic risk for autism in siblings compared to the general population (Holt et al. 2010) . Early findings suggested that mothers of children with ASD share HLA haplotypes with their children more often than the typically developing mother–child pairs. In addition, these children showed a higher rate of these haplotypes when compared with the healthy population (Gregg et al. 2008) . HLA haplotypes, considered among the strongest predictors of risk for autoimmune conditions, have also been associated with ASD (Fig. 9.1).
Studies in children and adults with autism have indicated other various candidate genes, many of them related to the immune system. These genes include the null allele of C4B in class III region, MHC haplotypes B44-SC30-DR4, HLA-DR β1, DR4, and DR13, all reinforcing also the factors lined as guttering in the development of autoimmunity (Daniels et al. 1995; Lee et al. 2006; Torres et al. 2006; Warren et al. 1995) . Genes regulating signal pathways related to the development of both neural and immune systems have also been referred to as altered in ASD: macrophage migration inhibitory factor (MIF) (Grigorenko et al. 2008) , MET encoding tyrosine kinase, (Correll et al. 2004) , the serine and threonine kinase C gene PRKCB1 (Lintas et al. 2009) , PTEN (Herman et al. 2007; Butler et al. 2005), and the reelin gene RELN (Garbett et al. 2008; Pardo and Eberhart 2007 ; Fig. 9.1).
9.5 Maternal Immune Activation and the Risk for ASD
As mentioned above, not only the adaptive but also the innate immune system response appears altered in ASD. These abnormal responses in children with autism could result from prenatal infection or exposure to toxic substances, and/or they could have a genetic component.
Various viral and bacterial infections during pregnancy have been linked to a higher risk for autism. More than 40 years ago, a higher risk for autism was reported in the offspring of maternal rubella infection cases (Chess 1971) . Since then, prenatal exposure to different virus have been linked to increased risk for ASD, including herpes simplex virus (DeLong et al. 1981; Ghaziuddin et al. 1992; Gillberg 1986; Greer et al. 1989) , cytomegalovirus (Ivarsson et al. 1990; Markowitz 1983; Stubbs 1978; Yamashita et al. 2003) , measles (Ring et al. 1997; Singh and Jensen 2003) , and viral meningitis (Barak et al. 1999; Ring et al. 1997) . Moreover, a larger study showed an increase in the prevalence of autism in children born to mothers hospitalized for viral infections during the first trimester of pregnancy or for bacterial infections during the second trimester (Atladottir et al. 2010) . These evidences support the idea that the maternal response to viral infection can be a risk factor for autism (Ciaranello and Ciaranello 1995) .
How can different pathogenic infections, all result in an increased risk for autism? Evidence from experimental animal models show that prenatal and early life inflammation can alter behavior later in life (Coe and Lubach 2005; Meyer et al. 2006, 2007, 2009; Depino 2006; see also Chap. 8). Therefore, different infectious agents, autoimmune diseases, and stressful events could elicit the expression of immune factors affecting brain development and resulting in the behavioral symptoms (Fig. 9.2).
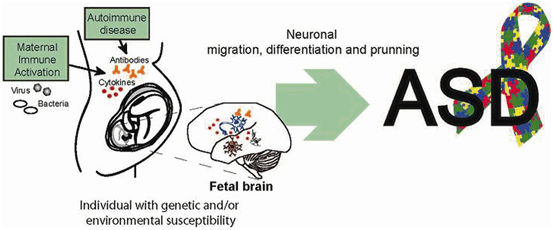
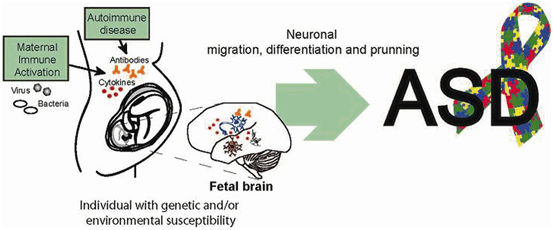
Fig. 9.2
Maternal immune activation can contribute to the development of ASD. Upon an infection, maternal cytokines and antibodies can reach the embryo’s brain and affect its development. In individuals genetically susceptible, these effects on neuronal development could result in ASD
Interestingly, fetal inflammatory response syndrome (FIRS) is a condition in which, despite an absence of cultivable microorganisms, neonates have very high circulating levels of inflammatory cytokines, such as IL-1, IL-6, IL-8, and TNF-α (Madsen-Bouterse et al. 2010) . Studies in animal models have shown that viral infection of the placenta triggers a fetal inflammatory response similar to the one observed in FIRS, even though the virus is not able to reach the fetus. In the case of human FIRS, these cytokines have been shown to affect the CNS and the circulatory system (Deverman and Patterson 2009) . Fetal morphological abnormalities in the animals have also been reported, probably caused by fetal proinflammatory cytokines such as IL-1, TNF-α, MCP-1, MIP-1β, and IFN-γ, and it has been suggested that beyond morphological effects on the fetal brain, the presence of FIRS increases the risk of autism, schizophrenia, neurosensorial deficits, and psychosis. Interestingly, all these factors induced at the neonatal period can predispose to diseases in adulthood (Romero et al. 2007) . Due to this, we propose that an inflammatory response in the placenta, altering the cytokine balance in the fetus, may affect the normal development of the fetal immune system leading to anomalous responses during childhood or later in life. In all these cases the parasite may never reach the placenta, but the inflammatory process at this level may affect the normal fetal development.
9.6 Gastrointestinal Immune Pathology Increase Susceptibility to ASD
Recently, it has been hypothesized that the presence of gastrointestinal inflammation makes a child with a genetic predisposition for ASD more prone to express the autistic phenotype, or that it increases the severity of the autistic behavior. A recent meta-analysis found that the reported prevalence of gastrointestinal symptoms in individuals with ASD can range from 9 to 91 % (Buie et al. 2010; Coury et al. 2012) . The variability observed could be mainly due to the lack of appropriate (nonrelated) control subjects, but the data is however consistent with a high prevalence of gastrointestinal symptoms and disorders in ASD individuals.
Gastrointestinal symptoms include abdominal pain or discomfort, constipation, diarrhea, and bloating (Afzal et al. 2003; Horvath and Perman 2002; Buie et al. 2010) . In addition, autism severity shows a strong correlation with gastrointestinal disturbances (Adams et al. 2011) , since studies in the field have mainly investigated immune dysfunction and the connection between the gut microbiome and the brain.
9.6.1 Gut Immune Dysfunction in ASD
Mucosal immune cells represent around 70 % of an individual’s immune cells, so their malfunction can affect the gastrointestinal environment. Clinical and pathological studies in ASD show an apparently atypical gastrointestinal immunopathology characterized by chronic ileocolonic lymphoid nodular hyperplasia and enterocolitis as key features, accompanied by a variable degree of acute inflammation and eosinophil infiltration (Wakefield et al. 2000; Furlano et al. 2001; Torrente et al. 2002) . Increased intestinal permeability in ASD also suggests an ongoing inflammatory process that may perturb the intestinal barrier function in these children (D’Eufemia et al. 1996) , probably through the release of peptides and mediators by the enteric nervous system, which can induce activated mast cells to release neurotoxins, proinflammatory mediators, and vasoactive substances that getting into the circulation can cross the blood–brain barrier (BBB) and affect the neuronal functions (Sun et al. 2007) , Fig. 9.3.
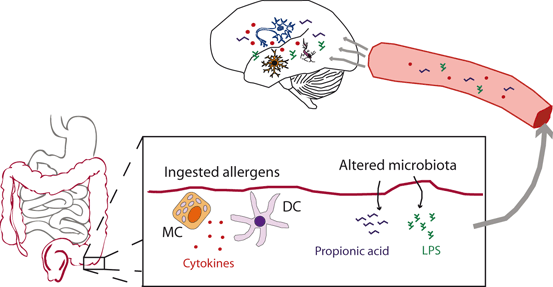
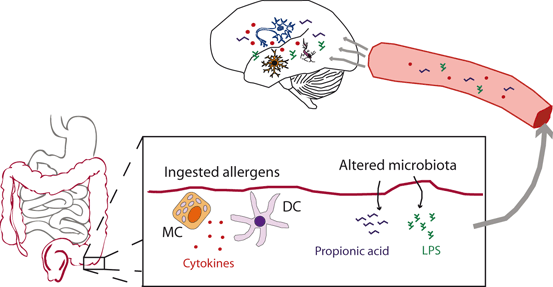
Fig. 9.3
Disturbances in the autistic gastrointestinal tract can affect brain function. Ingested allergens can elicit the secretion of cytokines (IL-1β, IL-4, IL-5, IL-6, IL-12, IL-13, IFN-γ, TNF-α) by mast cells (MC) and dendritic cells (DC). These cytokines can reach the brain through the circulation and cross the blood–brain barrier (BBB) or stimulate the brain endothelial cells, to induce a local immune response and cause a dysregulated neuronal homeostasis. In addition, changes in the microbiota can result in high levels of LPS and propionic acid reaching the circulation and acting in the brain, affecting brain function
In this context, a hypothesis relevant to understanding some alterations of mucosal immunity in autism could involve the functional plasticity of peripheral Foxp3+ nTreg cells (Fig. 9.4). Foxp3+ nTreg cells can become into Th1 and Th17 effectors cells, particularly in the gut microenvironment or sites of parasitic infection, following inflammatory signals that modulate the signal pathway and influence the stability of the Foxp3+ nTreg cell phenotype (Ahern et al. 2008; Liu et al. 2010) . Interestingly, it has been shown that the T cell receptor controls Foxp3 expression via the PI3K-Akt-mTor signaling pathway (Sauer et al. 2008) . The mammalian target of rapamycin (mTor) is also involved in synaptic function and plasticity (Hoeffer and Klann 2010) . Therefore, dysfunction in this pathway could be responsible for the aberrant cellular immune response (Mondino and Mueller 2007) and the changes in both neuronal and immune cell survival, both reported in autism. In addition, this reinforces the suggestion that cell survival mechanisms are altered in autism (Fatemi et al. 2001; Engstrom et al. 2003) . Moreover, mutations in the phosphatase and tensin homolog (PTEN), a major upstream regulator of the mTOR pathway, have been found in association with autism (Rodriguez-Escudero et al. 2011) . These findings could provide new directions toward a better understanding of the immune dysfunction in autism, as well as the identification of new therapeutic targets for ASD in the future.
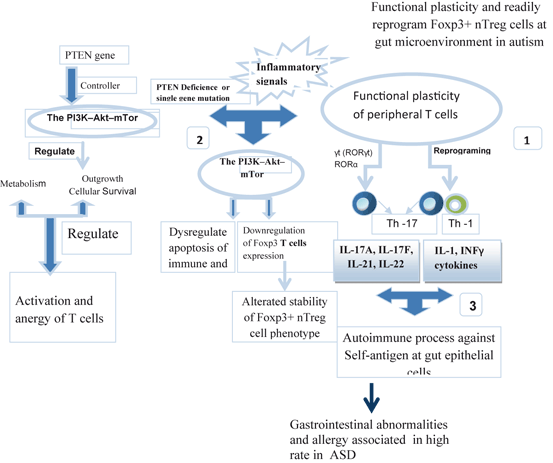
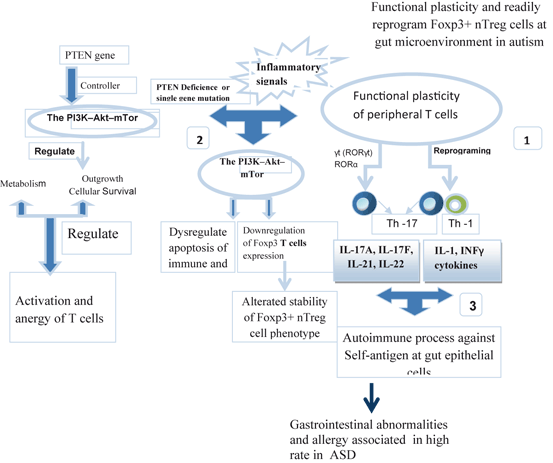
Fig. 9.4
Prominent functional plasticity and readily reprogram Foxp3+ nTreg cells. A hypothesis relevant to understand some alterations of mucosal immunity in autism could involve the functional plasticity of peripheral Foxp3+ nTreg cells. (1) Foxp3+ nTreg cells can convert into Th1 and Th17 effector cells particularly in the gut microenvironment, after an infection or other inflammatory signals. (2) PTEN gene is a controller of mTor pathway . Inflammatory signals and/or gene mutation are also able to modulate mTor pathway influencing the stability of the Foxp3+ nTreg cell phenotype, alterated in high rate in ASD. So, it has been seen that mTor inhibition stabilizes Foxp3 expression by suppression of nTreg cells. (3) Aberrant immune mechanism at the gastrointestinal tract, followed by the influence of ASD-associated cytokines and lymphocytes induce an increased permeability of the blood–brain barrier (BBB). Activated lymphocytes and serum cytokines crossing BBB, bind to brain endothelial cells to induce an immune response into the brain with a dysregulated neuronal homeostasis
Alternatively, co-localization of immunoglobulin and complement components on the epithelial membrane, in both stomach and duodenal specimens, could be indicative of an autoimmune process against self-antigen contained within epithelial cells and an inflammatory degradation that could contribute to disruption of the intestinal barrier function (Torrente et al. 2002; Furlano et al. 2001) .
Gastrointestinal pathology in ASD has been also evidenced by a higher number of helper and cytotoxic T lymphocyte infiltration and CD19-positive B cells at duodenum, ileum, and colon in biopsies of children with ASD (Ashwood et al. 2003; Torrente et al. 2002) , compared to histologically noninflamed controls, been more enhanced at terminal ileum and colon level (Ashwood et al. 2003; Torrente et al. 2002; Furlano et al. 2001; de Theije et al. 2011) . Thus, some pathways of intestinal inflammation may be susceptible to establish a neuroimmune interaction linked to this disturbance in support of this hypothesis in autism. Dietary antigens and bacteria, as well as their products, can become a target for immune regulation at the gastrointestinal tract and then influence the nervous system activity. So, ingested antigens entering the gut mucosa can access the Peyer’s patch or can be taken up by antigen presenting cell (APCs) that come to the Peyer’s patch or mesenteric lymph node, and there they interact with naïve T lymphocytes to initiate an adaptive immune response. In the context of chronic inflammation, memory T and B cells are activated, damaging the epithelial cell layer, releasing cytokines, and thereby increasing the intestinal permeability and causing a gastrointestinal inflammation similar to what has been found in autism (de Magistris et al. 2010; D’Eufemia et al. 1996) . In addition, it has been demonstrated that dietary derived antigen peptides are able to bind to the lymphocyte receptors and tissue enzymes CD69 and CD26, respectively, resulting in autoimmune reaction in children with autism (Vojdani et al. 2003; Siniscalco and Antonucci 2013) .
In this respect, mast cell–neuron interactions occurring in the gastrointestinal tract might influence behavior via mast cells or other immune cells, which are able to trigger enteric neurons to convey information through afferent pathways in vagal and spinal nerves to the CNS (Adams et al. 2011) .
Another pathway to reinforce the neuroimmune interaction surrounding the intestinal inflammation in autism is related to serotonergic neurotransmission. Serotonin is both a neurotransmitter and a mediator of inflammation, implicated early in the pathogenesis of autism (Schain and Freedman 1961) . Hyperserotonemia in one third of the patients with ASD (Hanley et al. 1977) and a decrease in the capacity of 5-HT synthesis in the brain of these children (Chugani et al. 1999) have been demonstrated in autism (Anderson et al. 1987; Minderaa et al. 1987; Hoekstra et al. 2010) . ASD-related hyperserotonemia was proposed to be from genetic (Holt et al. 2010; Sousa et al. 2010; Coutinho et al. 2007) , gastrointestinal (Anderson et al. 1987; Hoekstra et al. 2010; Holt et al. 2010; Hoekstra and Wheelwright 2009; Nijmeijer et al. 2010), or immune origin (Jyonouchi et al. 2005; Burgess et al. 2006; Warren et al. 1986) .
For example, an increased utilization of dietary tryptophan by the gut argue less tryptophan available for passage through the BBB with a reduced 5-HT levels in the brain leading to the mood and cognitive dysfunctions reported in ASD (Cappiello et al. 1996; McDougle et al. 1996) . Nevertheless, more research is required to establish from this analysis a therapeutic target in ASD, either by providing dietary tryptophan or by pharmaceutical treatments based on selective serotonin reuptake inhibitors.
Finally, during gastrointestinal inflammation, the immune cells produce cytokines and chemokines in the gut, which are released into the intravascular compartment to pass all organs, including the brain (Banks 2012; Erickson et al. 2012) . Therefore, gastrointestinal inflammation may influence the brain homeostasis and module characteristic behavior pattern in these patients.
9.6.2 Gut Microbiome and ASD
A probable factor contributing to the gastrointestinal disturbances in autistic individuals is the observed abnormal composition of gut microbiota (Finegold et al. 2002; Parracho et al. 2005; Adams et al. 2011) . Fecal microflora is qualitatively different in autistic subjects compared with controls and siblings (Finegold et al. 2010; Kang et al. 2013) , and they present an imbalance in beneficial bacteria (Adams et al. 2011) . Specifically, the flora of autistic children is rich in Bacteroidetes and the genus Desulfovibrio (Finegold et al. 2010; Finegold et al. 2012).
How can the microflora of autistic children contribute to their behavioral symptoms? Bacteria can produce a variety of harmful substances (Fig. 9.3). For example, Bacteroidetes produce propionic acid as end product of their metabolism. The intracerebroventricular injection of propionic acid in rats causes a decrease in sociability, an increase in repetitive behaviors and gliosis, and they have been proposed as a rat model of ASD (MacFabe et al. 2011) . The genus Bacteroidetes is highly abundant in the fecal material of autistic children (Finegold et al. 2010; Kang et al. 2013) .
Bacteria of the genus Desulfovibrio can be found in about 50 % of autistic subjects, in some siblings of autistic children, but not in normal controls (Finegold 2011) . Desulfovibrio is a Gram-negative anaerobic vibrio that produces lipopolysaccharide. Serum endotoxin levels are significantly higher in autistic children than in control subjects, and they show an inverse correlation with socialization in patients with severe autism (Emanuele et al. 2010) .
Finally, gut microbiota have an important role in the development and function of both the innate and the adaptive immune systems (Round et al. 2010; Olszak et al. 2012) . So, altered microbiota composition could also argue the immune alterations observed in ASD individuals.
In a small open-label trial with vancomycin (an antibiotic that is not absorbed in the gut), children with regressive autism showed behavioral improvement (Sandler et al. 2000) . However, the use of probiotics or antibiotic in the treatment of autism needs to be further evaluated.
9.7 Cytokines as Mediators for Autism Development
Autoimmune diseases, viral infection, gastrointestinal disturbances, and other proposed etiological and pathophysiological pathways in ASD share a common result: Changes in the levels of cytokines produced either in the periphery and/or within the brain. Hence, cytokines could be considered as the main molecular effectors acting on the brain to alter development and behavior.
A neuroimmune hypothesis on the role of cytokines in autism can be that: (1) proinflammatory cytokines arising from maternal inflammation, infection or allergy during pregnancy, cross the placenta, enter the fetal circulation and pass through the fetal BBB; (2) an excess of proinflammatory cytokines released into the fetal brain cause aberrant neurogenesis, alters synaptogenesis and neuronal function; and (3) altered brain development results in the behavioral symptoms, including reduced sociability, stereotypic and repetitive behavior, hyperactivity, attention deficit, anxiety, and hypersensitivity to environmental stimuli.
After an immune response is elicited, mechanisms are triggered to limit this response either in time or in magnitude. When these mechanisms fail, autoimmune or other pathological results can derive and affect the organism. So, as important to mount an effective immune response is to end it when the stimulus is eliminated. To this purpose, anti-inflammatory cytokines and endocrine factors are a key to limit the immune response, and their failure can lead to long-lasting immune diseases.
The analysis of cytokine profiles in autism pathology reinforces the implication of an immune deregulation in this disorder. The plasma of autistic patients presents increased levels of proinflammatory cytokines such as IL-1β, IL-6, and TNF-α (Ashwood et al. 2011a; Malik et al. 2011) , and reduced levels of anti-inflammatory cytokines such as TGF-β1 (Ashwood et al. 2008a). In addition, peripheral blood mononuclear cells (PBMC) from subjects with ASD show greater lipopolysaccharide-stimulated production of IL-1β (Enstrom et al. 2010b) , TNF-α, and IL-6 (Jyonouchi et al. 2008) than those from controls. Moreover, PBMC from ASD children stimulated with phytohemagglutinin have increased activation of both Th2 and Th1 cytokines (Molloy et al. 2006) . Finally, a multiplex assay for cytokines and chemokines in plasma from individuals with high-functioning ASD and matched controls showed increased plasma concentrations of IL-1β, IL-1RA, IL-5, IL-8, IL-12(p70), IL-13, IL-17, and GRO-α (Suzuki et al. 2011) . On the other hand, Onore and colleagues showed normal levels of IL-17 and decreased IL-23 in autistic children (Onore et al. 2009) . Another study revealed significant differences to IL-1β, IL-12(p70), IL-12(p40), and IL-17 in relationship to electrophysiological events, identified at EEG register and focused at temporal region (Robinson et al. unpublished results).
Altered brain cytokine levels have also been reported in ASD patients. Postmortem studies have shown high levels of TGF-β, IL-6, and MCP-1, as well as activated microglia in the brain of ASD subjects compared to controls (Vargas et al. 2005) . Moreover, increased expression of cytokines has also been reported in cerebrospinal fluid of ASD patients (Chez et al. 2007; Garbett et al. 2008; Li et al. 2009) .
Peripheral cytokines can not only alter immune functions but they can also affect different behaviors (Konsman et al. 2002) . Cytokines can reach the brain directly, or through activation of different communication pathways, including the gut–brain and immune–brain pathways.
During an allergic process, immunoglobulins and neuropeptides (e.g., substance P, NGF, VIP, and neurotensin) activate mastoid cells and basophils to release cytokines, which can in turn trigger enteric neurons. As the mast cell–neurons interaction occurs at the gut level, an allergic reaction at this level might influence behavior following the action of enteric neurons, which are triggered by mast cell to convey information through the vagal and spinal afferent pathways into the CNS. A hypothesis emphasizing the effect of allergy-linked inflammatory mediators in autism underlines that molecules like IL-6 and histamine promote gene transcription of estradiol and retinoic acid. This results in an overexposure of the fetus to retinoic acid and estradiol, which has been described as causing brain abnormalities similar to those observed in ASD, such as cerebellar malformations, abnormalities of dopaminergic system, and cranial nerve abnormalities (Garay et al. 2012) . Moreover, fetal overexposure to estradiol can result in anxiety, hyperactivity and attention deficit, stereotype and repetitive movement, and motor deficits (de Theije et al. 2011) .
For centuries, the structured system of the BBB, the lack of conventional lymphatic drainage, and the low response to alloantigen inoculated in the CNS, were arguments to consider this compartment as a privileged site. Nevertheless, today it is well known that there is no such privilege, but rather the main differences of the immune response in the brain, regarding the periphery, are in the kinetics and the degree of regulation of the different stages of the immune response at this level, which will take place under the functional control of the immune response and influence of cellular mediators acting in a regulated quantitative and temporally balanced sequence. Moreover, numerous cytokines are able to cross the BBB, including IL-1β, IL-6, and TNF-α (McLay et al. 2000; Prat et al. 2001) , whose plasma levels are enhanced in autistic patients (Ashwood et al. 2011b; Malik et al. 2011) . After reaching the brain, specific cytokines can affect brain development and function. For example, TGF-β involved in diverse aspects of development (Ashwood et al. 2008b; Letterio and Roberts 1998) . Levels of TGF-β have been found to be reduced in the plasma of autistic children and adults, in correlation with more severe behavioral scores (Ashwood et al. 2008b), while higher levels of TGF-β were described in postmortem brain and cerebrospinal fluid samples in ASD (Vargas et al. 2005) . This apparent contradiction could result from different roles of this cytokine in the brain and in the periphery, and/or result from different temporal roles of this cytokine during development. Indeed, using an animal model, it was shown that the overexpression of TGF-β1 in the brain can have opposite effects on behavior, dependant on the age of the animals (Depino et al. 2011) .
Macrophage inhibitory factor (MIF), other proinflammatory immune regulator that is constitutively expressed in the brain tissue, has an important influence on neural and endocrine systems (Grigorenko et al. 2008) . Recently, high plasma levels of MIF were observed in ASD individuals with severe behavioral symptoms, and genotyping studies linked MIF to ASD and showed the presence of two polymorphisms in its promoter region (Grigorenko et al. 2008).
In addition to others functions in the immune system, TNF-αα and IL-1β regulate brain processes such as neuron plasticity, long-term potentiation (LTP) and associative learning, and memory (more information in Chap. 7) (Depino et al. 2004) .
In the brain, cytokines can also act on neuroglial cells to induce neuroinflammation . Several factors released by astrocytes might be important for the induction and maintenance of the BBB, as manifested by the appearance of endothelial tight junctions in cells unsheathed by astrocytic endfeet. In the healthy CNS, astrocytes and microglia play important roles in neuronal function and homeostasis, as they are both fundamentally involved in cortical organization, neuroaxonal guidance, and synaptic transmission (Bilbo and Schwarz 2009) .
Astrocytes and microglia, produce neurotrophic factors, cytokines and chemokines (Bilbo and Schwarz 2009), regulate the integrity of the BBB (Prat et al. 2001) , and maintain brain homeostasis. Conversely, activated astrocytes and microglia can induce neuronal and synaptic changes, modifying CNS homeostasis, and contributing to neuronal dysfunction during disease. In postmortem brains of autistic patients, enhanced activation of astrocytes and microglia was observed in the subcortical white matter of the midfrontal gyrus, in the anterior cingulate gyrus, in the granular cell layer, in the Purkinje cell layer, and in the white matter of the cerebellum (Pardo et al. 2005; Vargas et al. 2005) . Similar changes have been observed in animal models of autism (Lucchina and Depino 2014; more information in Chap. 8).
9.8 Neurotransmission Disturbed and Immune Dysfunction in ASD
Altered brain development can result in long-term changes in neuronal function, evidenced by altered neurotransmitter production and receptor expression. In addition, an alternative to the hypothesis of cytokines acting on the brain to induce behavioral changes is to propose that changes in brain development can result in persistent alterations of neurons, which can in turn modulate immune function. Differential changes in neurotransmitter systems have been referred in autism, including serotonin (5-HT), dopamine, noradrenaline (norepinephrine), gamma-aminobutryic acid (GABA), glutamate, and neuropeptides suggesting that a dysregulation in neurotransmission could result in altered development. Molecules like oxytocin and vasopressin are involved in immune tolerance, serotonin exerts suppressive or proliferative responses on T cells , and the vasoactive intestinal peptide (VIP) has an immunomodulatory action susceptible to be implicated in ASD.
It has been also reported that cytokines can affect enteric glial cells, although their effects on mood, sleep, nutritional intake, exploratory behavior, and social interactions do not necessarily imply that they cause neuronal damage or death (Bernardo et al. 2004) . Systemic cytokine administration of IFN-α, IL-2, or TNF-α can cause increases in noradrenergic, dopaminergic, and serotonergic metabolism in the hypothalamus, hippocampus, and nuleus accumbens (Mohankumar et al. 1991; Shintani et al. 1993; Merali et al. 1997) .
Neurotransmission at cholinergic synapses is essential for functional aspects of cognition, reward, motor activity and analgesia, and a dysregulation of cholinergic neurotransmission has been associated with autism. Differences in the regional expression of specific nicotinic acetylcholinergic receptor (nAChR) subunits in postmortem adult brains from autistic and non-autistic individuals have been reported (Ray et al. 2005) . A decrease in the density of [3H]epibatidine binding sites within the parietal cortex, cerebellum, and thalamus of autistic brains was correlated to decreases in the levels of α3, α4, and β2 subunits (Martin-Ruiz et al. 2004; Lee et al. 2002) . Although without a total understanding on the impact of this finding in autism pathology, acetylcholine receptor (AChR) expression raises important questions on whether deficits in nAChR neurotransmission identified in at least a subset of autistic children might confer a particularly high sensitivity to the adverse effects of organophosphates and/or neonicotinoid exposure. Indeed, allelic variants of the gene that encodes for a metabolic enzyme for the detoxification of organophosphates have been associated with autism in North America, and the ASD-related variant has lower metabolic activity in vitro (D’Amelio et al. 2005) .
GABA receptors (GABAR) primarily mediate inhibitory responses, but also mediate excitatory transmission (Owens and Kriegstein 2002) . This temporally regulated effect of GABA has been proposed to underlie a key role of this neurotransmitter in conveying information of the environment into a developing brain (Ben-Ari 2002) . A failure in proper regulation of the chloride gradient can result in an imbalance between excitation and inhibition within developing neural circuits, a phenomenon that is proposed to underlie autistic symptoms (Snijders et al. 2013) . Changes in GABA levels have been found in platelets of autistic children (Rolf et al. 1993) . Moreover, the density of GABAR is diminished in the hippocampus of autistic individuals (Blatt et al. 2001) , and both glutamic decarboxylases (GAD) 65 and GAD67 are reduced in the autistic parietal and cerebellar cortex (Fatemi et al. 2002) . Differential levels of specific GABAA and GABAB receptors also suggest a role of GABA in autism (Schmitz et al. 2005; Fatemi et al. 2009a; Fatemi et al. 2009b) .
Central catecholamine imbalance has been reported as a major factor in the behavioral phenotype in autism, and a hypofunction of dopamine (DA) and norepinephrine systems has been also suggested in autism. Attention to a role of DA in autism was drawn after the observation that some DA blockers (i.e., antipsychotics) can treat some aspects of autism (Arnold et al. 2010) . However, the vast majority of studies measuring the levels of DA and its metabolites in the blood or the CSF of autistic individuals, have failed to detect differences with controls as was reviewed by Lam et al. (2006) .
9.9 Current Directions on Immunomodulation in ASD
The concepts of autoimmunity and neuroimmune dysfunction in neurodevelopmental disorders are current important areas of research. However, some precautions are required in interpreting the information at this time of knowledge, since much of the evidences are not rigorous and most are aimed at identifying potential markers, with only preliminary evidences on the mechanisms underlying their involvement in the pathology of ASD.
Many of the experimental data mentioned in our review only suggest or support a primary role of failure of immune functions in the pathogenesis of this particular neuropsychiatric disorder, which does not imply that such a primary role could have been formally proved. We consider that some mechanisms underlying these links could be discovered using the recently developed and validated animal models (see Chap. 8).
This understanding may contribute to designing better protocols of treatment, useful to recover from neuronal dysfunction related to social interaction, verbal and nonverbal communication, as well as the repetitive, stereotypical, and overly restrictive behaviors present in ASD.
In the context of immunomodulatory therapy in ASD, it has been suggested that around 70 % of the children affected undergo complementary and alternative medicinal therapies to treat their symptoms (Levy et al. 2003; Wong and Smith 2006) , including the use of probiotics, anti-infectives, and dietary augmentation (Golnik and Ireland 2009) . The clinical trials aimed at testing therapies that modulate the immune response in children with ASD have addressed mainly the use of steroids, intravenous immunoglobulin G, antibiotics, and vitamin D (Cannell 2008; Gupta 1999; Plioplys 1998; Pradhan et al. 1999; Sandler et al. 2000; Dalakas 1997; Shenoy et al. 2000; Matarazzo 2002; Bradstreet et al. 2007; Buitelaar et al. 1990; Geier and Geier 2005) . Understanding the biological effects of these treatments could probably provide a better background toward more effective conventional and alternative therapies.
9.10 Conclusions
Although it is well known that autism affects primarily the brain function (mainly social functioning and cognition), it is also known that it follows the compromise of other organs and systems. So, published evidences have identified widespread changes in the immune system of children with autism, both at systemic and cellular levels. Brain specimens from autistic subjects exhibit signs of active, ongoing inflammation, as well as alterations in gene pathways associated with immune signaling and immune function. Moreover, many genetic studies have also indicated a link between autism and relevant genes to both the nervous system and the immune system, which can in turn affect function in both systems. Together, these reports suggest that autism may in fact be a systemic disorder with connections to abnormal immune responses in and outside the brain. Consequently, the immune system dysfunction may represent a novel target for treatment. Hence, a better understanding of the involvement of the immune response in autism, and of how early brain development is altered, is crucial for a better and earlier intervention of the disease and has therapeutic implications. In our opinion, this chapter is a modest contribution to these propositions.
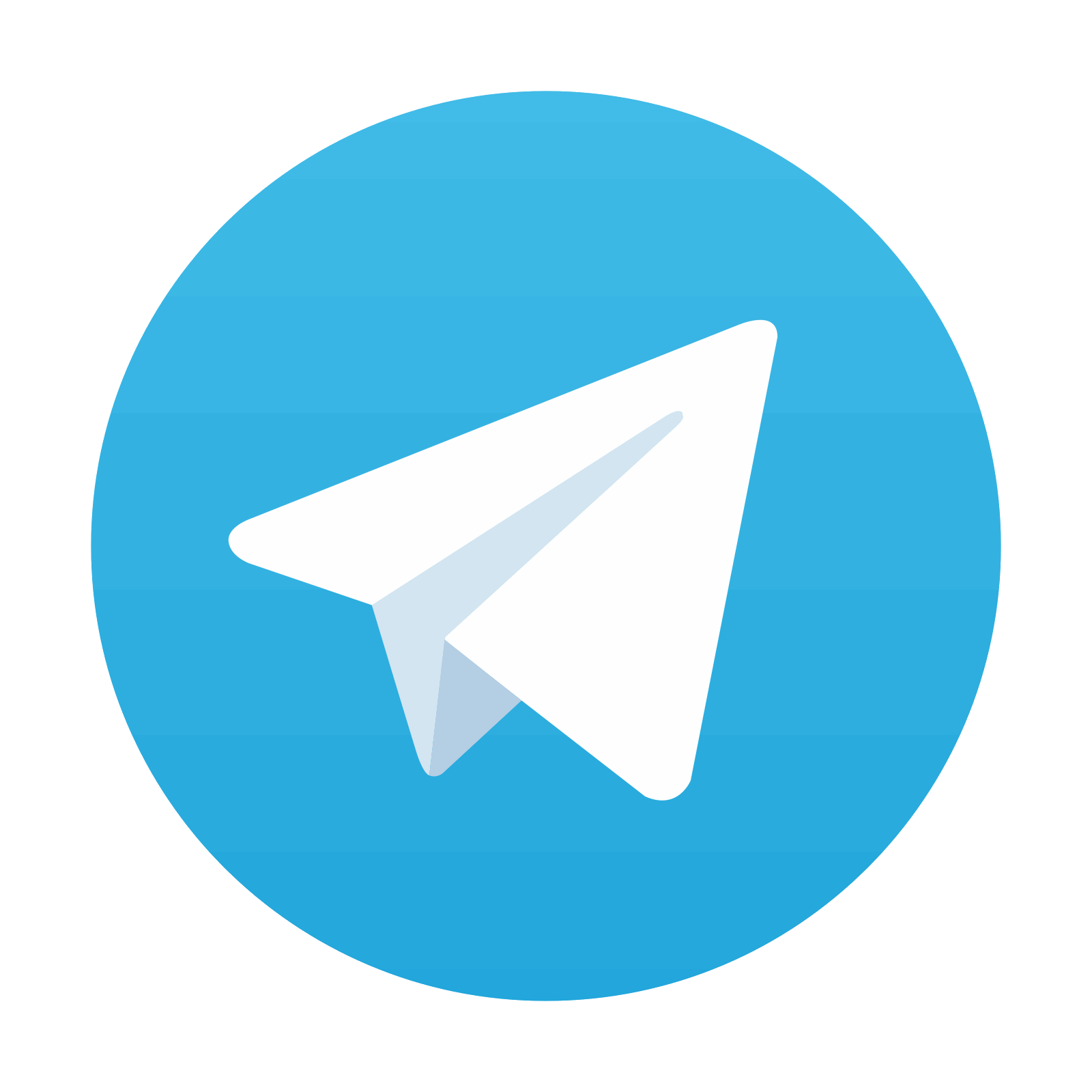
Stay updated, free articles. Join our Telegram channel

Full access? Get Clinical Tree
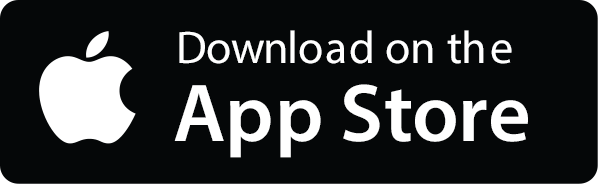
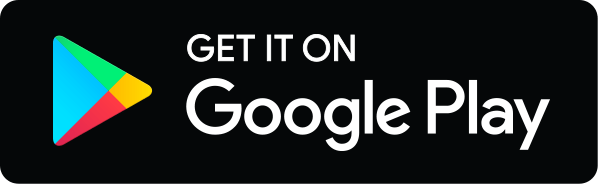