Abbreviations
BOLD
blood oxygen level-dependent
CS
cervical spondylosis
CST
corticospinal tract
CSM
cervical spondylosis myelopathy
DTI
diffusion tensor imaging
FA
fractional anisotropy
FC
functional connectivity
GM
gray matter
fMRI
functional magnetic resonance imaging
MD
mean diffusivity
MR
magnetic resonance
MRI
magnetic resonance imaging
M1
primary motor cortex
RSNs
resting-state networks
RS-fMRI
resting-state functional MR imaging
SCI
spinal cord injury
SMC
sensorimotor cortex
SMA
supplementary motor area
S1
primary sensory cortex
SMG
supramarginal gyri
WM
white matter
Introduction
Compression of the cervical spinal cord can be caused by a wide range of causes, but the most common is cervical spondylosis (CS), a chronic degenerative process that affects the vertebral bodies and inter-vertebral discs of the cervical spine ( Table 1 ). CS is frequently found in asymptomatic adults after the fifth decade of life, but may progress to disk herniation, bone spur formation, compression of the spinal cord, and cervical spondylotic myelopathy (CSM) ( ).
Traumatic causes | Atraumatic causes |
---|---|
Vehicular accidents | Degenerative spondylosis |
Falls | Intervertebral disk herniation |
Violence | Metastatic disease |
Sports | Primary spinal cord tumor |
Recreation activities | Spinal epidural abscess |
Spinal epidural hematoma | |
Scoliosis | |
Rheumatoid arthritis | |
Bone diseases |
The compression of the spinal cord by CS is a slow and progressive process caused by the reduction of the spinal canal diameter by dynamic and static factors ( ). In CS, any flexion or extension of the cervical spine causes the intermittent compression of the spinal cord. This progressive compression leads to several changes such as apoptosis of the anterior horn neurons, ischemia, gradual spongy necrosis, demyelination of the white matter (WM), and Wallerian degeneration ( Fig. 1 ; ). Despite it may affect one single segment of the spine, multi-level involvement is very frequent, generally at the lower segments. Contrary to the lumbar spine, which encloses only a small part of the spinal cord, stenosis of the cervical spinal canal can induce a direct compromise of the whole spinal cord and severe neurological deficits.

Characteristically, in CS, the severity of compression and deficits varies greatly across individuals. Some patients experience a benign form of the disease with stable periods lasting from months to years, while others develop CSM with neurological deficits and a considerable deterioration over time ( ; ). If CSM fully develops, the symptoms are progressive and in a stepwise manner ( ), with a wide range of clinical manifestations including weakening or sensory loss in one or more limbs, gait unsteadiness, fine motor deficits, and non-specific neck and shoulder pain with or without radiculopathy.
Diagnosis of CSM is based on physical examination and clinical imaging, usually with Magnetic Resonance Imaging (MRI). MRI allows to evaluate the vertebral discs, ligaments, subarachnoid space, spinal cord, and quantify the spinal stenosis. Still, it lacks sensitivity to provide information about the integrity of the spinal cord, presents a poor correlation with neurological and functional impairment, and does not provide reliable prognostic information to predict surgical outcomes ( ). Recent advances in MR techniques have started to overcome these limitations by providing invaluable information about CSM pathology, with several studies reporting that prolonged cervical spine compression affects not only local structures but also distally from the site of injury. For instance, numerous brain changes, such as cortical and subcortical atrophy, functional reorganization of the sensorimotor cortex (SMC), and connectivity impairment, have been reported ( ). These observations have had a great impact on the understanding of CSM, framing the disease as multi-factorial and complex, with its pathophysiology still being poorly understood. In this sense, a clear limitation to advancing in the field of CSM pathology has been the lack of reliable animal models.
Understanding the sequence of structural and functional changes of CSM at the brain level, and defining their effects on the clinical outcome is of crucial importance to better understand the disease, to prevent its fatal consequences, and to develop the best evidence-based rehabilitation therapies. In the next sections, we will summarize the current knowledge on the effects of prolonged spinal cord compression on the brain ( Figs. 2–5 ).




Cervical spine compression causes brain atrophy
There is increasing evidence supporting that spinal cord injury (SCI) may cause destructive cortical changes ranging from atrophy of projecting neurons to cell death ( ; ). In animal models, after SCI, the disruption of the motor efferents and sensory afferents results in atrophic changes of the neural sensorimotor systems ( ). Indeed, several studies have demonstrated that SCI can induce cortical atrophy on the supraspinal network, whose degree correlates with the spinal cord atrophy ( ) and the functional status ( ).
There are still a few works exploring the effect of prolonged spinal cord compression by CS in the brain. However, the results are consistent reporting cortical atrophy in the primary SMC, with additional volume changes at the thalami, specifically at the pulvinar nuclei ( ; ). Furthermore, supplementary brain areas related to the execution of complex movements (such as gait, grasp, and fine hand-motor coordination) are also atrophied ( ; ). Metabolic studies using MR-spectroscopy on CSM patients have also reported neuronal damage and impaired metabolism on the SMC that is not recovered or may worsen after surgery in spite of clinical improvement ( ; ).
In a recent study regarding chronic CS, a consistent pattern of brain changes associated with neurological function and neck pain was observed ( ). Specifically, cortical thinning in the superior frontal regions, anterior cingulate, insula, and precuneus were present. Furthermore, atrophy in the putamen, BA3a, and primary motor regions when worsening neurological symptoms was reported. Taking into account the role of BA3a as an afferent receiver of proprioception ( ), the observed atrophy in this study may explain the deficits in proprioception (gait and hand function) reported in CSM patients ( ).
CS patients may also present brain changes to chronic pain in the same fashion as other chronic pain conditions. Specifically, a cortical thinning within the insula and anterior cingulate cortex has been reported ( ). Finally, a faster rate of cortical thinning and atrophy compared to age-matched healthy subjects in CS patients has been observed ( ).
The significance of these findings is that despite CS has been traditionally considered as a benign disease typically present in the old-age population, it may, if not properly monitored, harm the brain inducing progressive atrophy. In this sense, the CS-related brain atrophy may become a potential obstacle to clinical recovery after surgery, even if significant repair is achieved at the site of compression.
Cervical spine compression causes neural plasticity to preserve function
One of the most fascinating characteristics of the central nervous system is its ability to self-repair, reorganize after injury, and compensate for functional loss. This mechanism, known as neural plasticity (or functional reorganization), is present in numerous brain conditions ( ) and has also been observed in SCI. The exact mechanism is not fully understood but may occur through synaptic modifications of pre-existing connections and/or by the development of new circuitries ( ).
Several studies have shown that SCI, although it does not directly involve cortical neurons, may alter the functional activation pattern of the SMC. These observations have been achieved with different physiological and imaging techniques such as functional MRI (fMRI). fMRI is a non-invasive imaging modality that allows to detect changes in brain activation over a period of time while a specific task is being performed. It can be used to localize areas of brain activation secondary to several cognitive and motor tasks, allowing to map the cortical representation of neural activation ( ).
In CSM patients, fMRI studies have shown the existence of significant changes in the cortical activation pattern during sensorimotor tasks. These changes consist of the expansion of the cortical representation for the affected limb by the inclusion of adjacent motor territories ( ). Recruitment of supplementary areas outside the SMC including globus pallidus , left thalamus, caudate nucleus, and both cerebellum hemispheres have been reported. Additionally, decreased activation in SMA and both SMC, as well as increased activation of both cerebellum hemispheres, has been observed ( ). Interestingly, the displacement and activation of the sensorimotor areas in CSM appeared in the vicinity of the areas with gray matter (GM) atrophy, suggesting that the displacement and recruitment of new cortical areas may be mediated by adaptive changes to GM atrophy ( ). This functional reorganization is closely associated with the severity of the SCI ( ), helping to maintain a residual motor function, and contributing to its restoration if the disease progression is halted ( ). Indeed, these adaptive changes may also explain why some CSM patients with significant SCI are able to perform motor activities with apparently minor neurological deficits.
Comparison of pre-operative and post-operative studies has shown that surgical decompression of the cervical spine leads to cortical reorganization, probably by the recovery of conduction in the preserved axons at the spinal cord ( ). After surgical decompression, the altered SMC recruitment pattern gradually disappears ( ), and everything progresses toward an activation pattern similar to the observed in healthy controls ( ). There is an increased activation in the contralateral primary motor cortex (M1) concomitant with the improvement of the motor function and the extent of spinal cord expansion ( ; ). Also, a gradual disappearance of the ipsilateral primary SMC activation in addition to a progressive increase in the contralateral SMC and SMA is observed ( ). This normalization continues over time and can be enhanced by activity-dependent mechanisms related to task practice and learning.
In summary, according to recent studies, CSM patients present compensatory mechanisms that consist of an increase and expansion of the cortical representation of the motor areas, especially those devoted to the hands. After the surgery, there is a tendency to normalization of the sensorimotor representation. However, the reported results are not completely consistent. This is probably because CSM patients form a heterogeneous group with different levels of impairment, different degrees of SCI, and different natural histories. In addition, there is no uniformity in the timing of imaging after the surgery. Still, the reported observations provide direct evidence of neuronal plasticity in the SMC of CSM patients that may play a main role in the post-operative motor functional recovery.
Cervical spine compression changes the functional connectivity of the brain
Few studies have analyzed the alterations of the whole-brain network in CSM. These studies have been performed with resting-state functional MR imaging (RS-fMRI), a new technique that allows evaluating the functional relationship between brain areas, and enables the visualization of brain functional connectivity (FC) between spatially separated brain regions. RS-fMRI is a robust method that uses the endogenous brain activity detectable with blood oxygen level-dependent (BOLD) to identify areas that are interacting at rest. With RS-fMRI spontaneous low-frequency BOLD-fluctuations in neural activity (known as amplitude of low-frequency fluctuation) are detected, allowing to establish the different anatomically correlated functional networks ( ). As a result, the resting-state networks (RSNs) maps are obtained which represent topographies of functionally connected regions across the brain, helping to detect functionally relevant changes after injury to the central nervous system, if present.
In CSM, RS-fMRI studies have reported that compression of the cervical spinal cord alters the connectivity pattern of the sensorimotor and visual cortices of the brain. Specifically, an overall increase in the FC in the sensorimotor cortex and supplementary areas (precuneus, superior frontal gyrus, and anterior cingulate), and between the cerebellum and the pre-and post-central gyri has been reported. This increased FC correlates with the worsening of the neurological symptoms, suggesting that the altered FC between the SMC and supplementary areas might represent compensatory mechanisms for accomplishing motor tasks ( ).
In a recent study, increased brain activity in the primary SMC and decreased brain activity in visual cortices and both supramarginal gyri (SMG) were observed. Characteristically after surgical decompression, the brain activity of the sensorimotor and visual cortices normalized ( ). In another study by Chen et al., the authors only reported decreased neural activity in the primary visual cortex of CSM patients and an increased FC between the visual cortex and the posterior cingulate lobe ( ). These observations suggested that the visual cortices were impaired, which accounted for the blurred vision observed in the patients of the study. Interestingly, the visual impairment also improved after surgical decompression. Considering that the cingulate gyri are related to the dorsal attention network, that in turn is involved in the control of visual attention ( ), the authors concluded that the enhanced FC in the visual attention network was an adaptive mechanism to compensate for the diminished activity observed on the primary visual cortex.
In another study, increased FC between the left thalamus, the visual system (bilateral lingual gyrus, cuneus), and the right cerebellum posterior lobe, that correlated with the sensory scores ( ), were found. Moreover, decreased FC between the sensory and motor cortices was observed. The authors concluded that the increased FC in the visual-related network acted as a compensatory mechanism to the decreased somatosensory transmission information detected in the patients. Likewise, increased FC between the brainstem and visual cortex in both posterior parietal cortices and its association with neurological impairment and neck disability has been described in CSM ( ).
While the altered FC in the SMC is in line with previous fMRI literature during sensory and motor tasks, the observation of increased FC in the visual cortex is surprising as CS is not a primary visual disorder. In this sense, Wang et al. propose that the observed increased FC in the visual cortex may be secondary to the need for more visual input to optimize body movements, mechanics, and balance ( ). This observation is in line with the work of Chen et al., where the authors suggest a compensatory compensation for the insufficient input from the proprioceptive system ( ).
In summary, brain plasticity is present in CSM patients to overcome functional deficits. The main changes are long-term FC reorganizations in the sensorimotor regions and areas assisting in the regulation of movement and sensory perception. This altered connectivity between various sub-networks may have prognostic potential and/or therapeutic implications in CS patients.
Cervical spine compression also damages the brain WM
Progressive chronic compression of the spinal cord also results in many pathological changes in the WM that are detectable using diffusion tensor imaging (DTI). DTI is an MRI technique that allows the evaluation of micro-structural changes in the WM by measuring the motility and orientation (anisotropy) of water molecules in tissues. DTI evaluates several parameters, the most important are: (1) mean diffusivity (MD), and (2) fractional anisotropy (FA). FA is a scalar value between 0 and 1 that evaluates the preferred direction of which diffusion occurs in the central nervous system and is the most sensitive parameter to detect SCI ( ). FA is largely attributed to the transverse axonal barriers in WM (i.e., myelin sheath, axon membrane) restricting the water diffusion to a preferred longitudinal orientation. Reduced FA values indicate a disturbance in the directional orientation of nerve fibers and are associated with reduced myelin integrity ( ).
In CSM, most of the DTI studies have been centered on the local changes at the spinal cord, reporting differences in diffusion characteristics of the water molecules at the site of compression, that are associated with increased neurological dysfunction ( ; ; ). Specifically, decreased FA values have been found in stenotic segments ( ) in the lateral and posterior column of WM suggesting significant myelin damage ( ). Likewise, decreased FA values and increased MD values have been found in CS patients in the early stages of the disease even in cases without visible myelopathy in the MRI examination ( ); suggesting that DTI presents higher sensitivity in myelopathy detection compared to conventional MRI.
In the brain, current studies in SCI have mostly analyzed the effect of traumatic etiology. In these reports the results show WM damage in supraspinal areas along the corticospinal tracts in the medulla, pons, midbrain, posterior limbs of the internal capsule ( ; ), and both centrum semiovale ( ). Furthermore, the CST damage correlates with the brain functional reorganization, the lower cross-sectional spinal cord area, and the upper limb ability ( ). Characteristically, this WM damage starts early after the traumatic SCI, with a pronounced and progressive decrease of the spinal cord area concomitant with a reduction in the cranial CST and GM volume at the SMC ( ). These changes are assumed to be induced by retrograde degeneration along the CST axons that over time results in shrinkage of the soma of corticospinal projecting neurons. Overall, the studies in traumatic SCI suggest widespread changes in WM micro-structure in the CST and in areas adjacent to the SMC that in turn associate with the clinical state of the patients.
Although there are few reports on CS, similar results have been found suggesting that CS causes anterograde and retrograde degeneration of the spinal WM tracts, resulting in axonal loss and demyelination that may extend cranially to cerebral regions. For instance, micro-structural changes in the ventral horns above the level of injury that correlated with diffusivity changes in the CST have been observed ( ). Similarly, Wen et al. observed WM damage at the region of the CST that correlated with the myelopathy severity in CSM patients ( ). Thus, both studies suggest a direct association between neurodegeneration affecting the motor system at the spinal cord, the CST integrity, and clinical impairment.
Characteristically, in CS patients, significant WM damage at both CST independently of the affected limb and corpus callosum has been reported. At the corpus callosum, significant damage to the superior and posterior parietal projection fibers, areas related to motor and touch-stimulation ( ) were observed. Tractography results on CS patients also suggested a common pattern of WM damage, being affected the anterior-distal segments, regions directly linked to the motor cortex. The preserved regions at both CST were those arising from S1, which are more posterior and medial than those of M1 and thus less vulnerable to compression. Besides, a direct association between the damage at the CST, the clinical scores, and the evolution after surgery was observed ( ). These observations suggest that CST metrics with DTI may be used as a potential biomarker in the clinical setting to determine the impact of the disease and predict outcomes ( ).
The CST is the largest descending pathway that connects the motor cortex to the brain stem and spinal cord and is mainly responsible for the movement of the distal extremities, particularly fine motor activities of the hand ( ). It is particularly vulnerable to the negative effects of cervical spinal compression suffering early demyelination. Recent reports on SCI both of traumatic and compressive origin suggest that the predominant pathological mechanism on both conditions is the demyelination of the ascending and descending CST fibers, leading to a progressive loss of axonal conductivity ( ), with additional connectivity impairment between the SMC and the brainstem ( ). The impact of the CST damage in the brain cortex has been previously described in other pathologies such as stroke. In these patients, whereas the degree of CST injury strongly predicts the resulting motor impairment ( ; ), the neurological function tends to be preserved by the reorganization and recruitment of new pathways as well as other motor network nodes to optimize the residual neurological function ( ; ). In this sense, it is believed that most of the axotomized CST neurons are able to maintain the sensory-motor circuits through axonal sprouting ( ). However, if CST neurons are damaged, whittling down the population by spinal compression over time may lead to some critical threshold to impairment. This could be the reason why some CSM patients do not present a significant clinical improvement of their symptoms after surgery.
In summary, spinal cord compression leads to significant WM damage mainly at the corticospinal tracts leading to a progressive loss of axonal conductivity that extends cranially to the brain. This reduced connectivity associates with the neurological impairment and may constitute a potential barrier for motor recovery after surgery.
Conclusion and future perspectives
As the population ages, the incidence of CSM will increase, but the knowledge of the effects of CSM in the brain is still sparse. On the other hand, there are no high-evidence studies providing well-founded recommendations for the best treatment modality. Considering that CSM has a potentially devastating outcome more studies are needed to better clarify the evolution of the disease and its consequences. Furthermore, to improve clinical outcomes the strategies should be equally targeted on the recruited adjacent cortex and the primary SMC. In this framework, we propose that increased collaborations among clinicians, experts in neuroimaging techniques, and basic researchers will enhance our ability for a better management of the disease.
Applications to other areas of neuroscience
Cervical spondylosis myelopathy (CSM) is the major cause of disability in the elderly and, despite significant ongoing research, current therapeutic approaches have limited effectiveness and are far from satisfactory ( ). One potential barrier is that current treatments generally assume intact neural motor systems for driving limb movements, but we should consider that this condition can lead to neuronal damage distal to the spinal lesion and cerebral reorganization. Therefore, the brain atrophy and the damage of cortical pathways in CSM could become a potential obstacle for achieving full and effective function recovery, even after successful surgical repair at the site of compression.
Advanced neuroimaging techniques can be applied to detect specific secondary brain damage in CSM. However, brain reorganization is not a static process, but rather very dynamic. Cortical reorganization starts early after spinal cord injury, and its impact over time will depend on a complex balance between spinal recovery and supraspinal reorganization ( ). Thus, it is crucial to better understand how the brain reorganizes after the injury, as well as develop cost-effective therapies to maximize functional recovery. In this framework, therapies designed to enhance brain plasticity could provide functional benefits for these patients both before and following surgery ( ).
The findings of a progressive degenerative pattern in spinal cord compression suggest the need to properly monitor the disease. Advanced Magnetic Resonance techniques may present a main role in non-invasive assessment to better predict the patient’s outcome as well as to identify the patients that will most likely benefit from different therapies and prioritize which patients will precise surgery more urgently.
Mini-dictionary of terms
Cervical spondylosis : Term to define the progressive degenerative changes that affect all the components of the cervical spine (i.e., inter-vertebral discs, facet joints, joints of Luschka, ligamenta flava, and laminae).
Cervical spondylosis myelopathy : Term to define injury to the spinal cord due to severe compression caused by cervical spondylosis.
Corticospinal tract : White matter motor pathway that connects the cerebral cortex with the lower motor neurons and inter-neurons in the spinal cord.
Diffusion tensor imaging : MR technique based on the diffusion of water molecules of the tissues that allows to obtain information about the white matter architecture and integrity.
MR-spectroscopy : MR technique that allows to obtain the chemical composition of tissues, it is used to explore in vivo tissue metabolism and to detect and monitor several pathologies.
Functional magnetic resonance imaging : A technique that allows to obtain the brain activation pattern of a subject while performing a specific task. It is commonly used in the clinical setting to map cortical-activated areas before surgery to preserve their function. It is also widely used in research to study differences in brain activation patterns.
Functional reorganization : Also known as neural plasticity, neuroplasticity, or brain plasticity. The ability of the central nervous system to adapt in response to lesions or changes in the environment. This process could be accomplished through the recruitment of new/different neural networks, changes in the connectivity pattern, and the formation of new synapses.
Resting-state functional magnetic resonance imaging : A technique that allows to obtain the connectivity pattern of functional brain areas at rest that are spatially separated.
Sensorimotor cortex: Brain area located at the pre-central and post-central gyri which contain the primary motor and somatosensory areas of the brain.
Supplementary motor area : Brain area located in the midline cortex, anterior to the primary motor cortex that is responsible for movement control and coordination.
Key facts of spinal cord compression
- •
The most common cause is cervical spondylosis which is frequently found in asymptomatic adults after the fifth decade of life.
- •
Cervical spondylosis may progress to cervical spondylosis myelopathy, a progressive condition that causes incomplete spinal cord injury and associated neurological impairment in a stepwise manner.
- •
At present most of the studies have been focused on the local changes at the cervical spine, showing apoptosis of the anterior horn neurons, gradual spongy necrosis, demyelination of the white matter, and Wallerian degeneration.
- •
Cervical spondylosis may present some similarities with traumatic spinal cord injury.
- •
Cervical spondylosis leads to adaptive changes in the brain to compensate for the functional loss, causing a wide range of clinical manifestations.
Key facts of advanced MRI techniques
- •
Present high sensitivity to detect neural damage secondary to cervical spondylosis.
- •
Allow a better understanding of the consequences of cervical spine compression in the brain.
- •
May be useful for identifying those patients at risk of severe impairment.
- •
May help the non-invasive assessment of cervical spondylosis, to better predict outcomes and to identify the patients that most likely will benefit from different therapies.
Summary points of cervical spine compression
- •
Leads to brain atrophy of the sensorimotor cortex, thalami, supplementary motor areas, and a faster rate of cortical thinning and atrophy related to age.
- •
Causes functional reorganization of the sensorimotor cortex that is more significant for the hand.
- •
Alters the FC of the brain at the sensorimotor cortex and visual cortex to compensate for the functional loss.
- •
Leads to white matter demyelination of corticospinal tracts, which extend cranially to the brain cortex mainly at the primary motor area.
- •
Causes myelin damage at the corpus callosum, mainly at the superior and posterior parietal projection fibers that are related to motor and touch-stimulation.
References
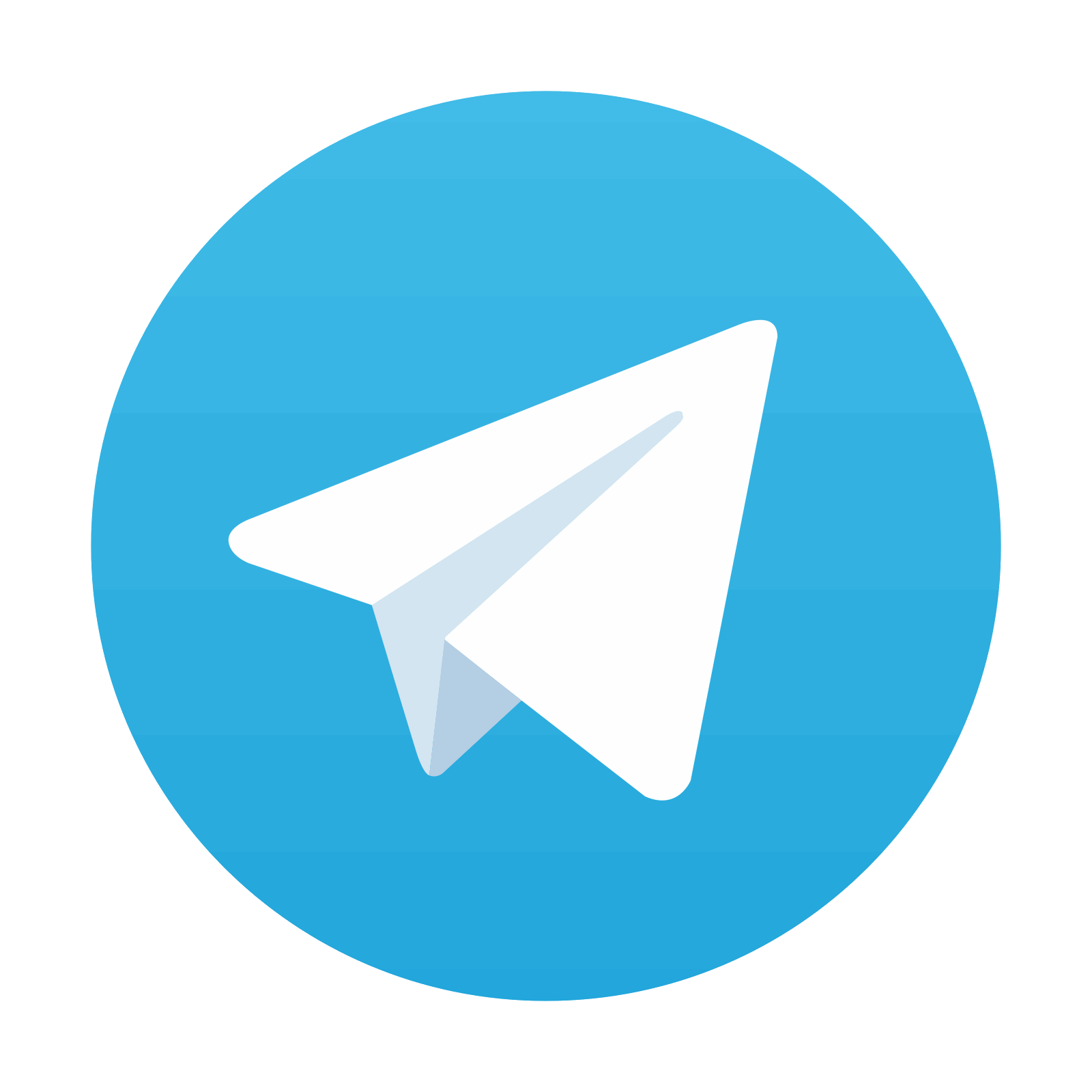
Stay updated, free articles. Join our Telegram channel

Full access? Get Clinical Tree
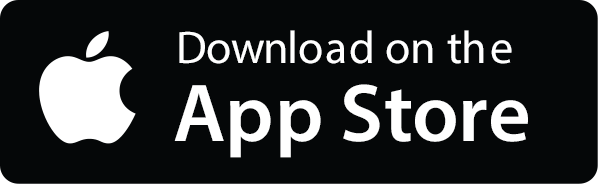
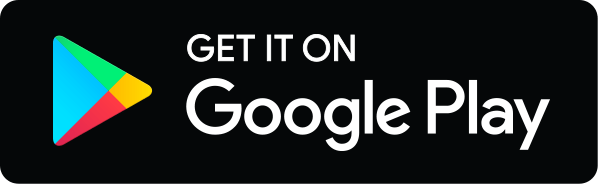
