List of abbreviations
AGEs
advanced glycation end products
AD
Alzheimer’s disease
ACR
acrylamide
AKT
protein kinase B
APP
amyloid precursor protein
AQP4
astrocyte expressing aquaporin-4
ARE
antioxidant response element
α7nAChR
alpha-7 nicotinic acetylcholine receptor
BBB
blood-brain barrier
Bax
Bcl-2-associated X protein
Bcl-2
B-cell lymphoma 2
BDNF
brain-derived neurotrophic factor
CAT
catalase
COX-2
cyclooxygenase-2
EGCG
(−)-epigallocatechin-3-gallate
eNOS
endothelial nitric oxide synthase
FASN
fatty acid synthase
FGF2
fibroblast growth factor 2
GFAP
glial fibrillary acidic protein
GDNF
glial cell line-derived neurotrophic factor
GSH – Px
glutathione peroxidase
GSK-3
glycogen synthase kinase-3
HMGB1
high-mobility group box 1 protein
IRI
ischemia-reperfusion injury
iNOS
inducible nitric oxide synthase
IL-1β
interleukin-1 beta
IL-4
interleukin-4
IL-6
interleukin-6
67LR
67 kDa laminin receptor
LPS
lipopolysaccharide
MDA
malodialdehyde
MDI
motor deficit index
MMP-9
matrix metalloproinase-9
MIP-1α
macrophage inflammatory protein 1-alpha
MPO
myeloperoxidase
NF-κB
nuclear factor Kappa B
NGF
nerve growth factor
Nrf2
nuclear erythroid 2-related factor 2
NF-L
neurofilament triplet L
NADPH-d
nicotinamide adenine dinucleotide phosphate-diaphorase
NO
nitric oxide
NOS
neuronal nitric oxide synthase
nNOS
neuronal nitric oxide synthase
NT3
neurotrophin-3
NGFR-p75
nerve growth factor receptor p75
PARP
poly(ADP-ribose) polymerase
PD
Parkinson’s disease
PKC
protein kinase C
PI3K
phosphatidylinositol 3-kinase
ROS
reactive oxygen species
RhoA
ras homologue gene family member A
SCI
spinal cord injury
SOD
superoxide dismutase
TNFα
tumor necrosis factor alpha
TUNEL
terminal deoxynucleotidyl transferase dUTP nick end labeling
TLR4
Toll-like receptor 4
Trk-B
tropomyosin receptor kinase B
Trk-C
tropomyosin receptor kinase C
VEGF
vascular endothelial growth factor
Introduction
Over the past decades, a large number of polyphenolic compounds with neuroprotective effects have been described. One of the main sources of these molecules is green tea, the most widely consumed beverage next to water. The chemical composition of green tea contains a number of bioactive components, mainly including polyphenols, caffeine, and amino acids ( ). Green tea polyphenols, generally known as catechins which make up 30% of the dry weight of green tea leaves, are the main bioactive constituents of green tea with a wide variety of beneficial health effects ( ). The main catechins in green tea include (+)-catechin, (−)-epicatechin, (+)-gallocatechin, (−)-epigallocatechin, (+)-catechin gallate, (−)-epicatechin gallate, (+)-gallocatechin gallate, and (−)-epigallocatechin-3-gallate (EGCG) ( ). EGCG, chemically (2 R ,3 R )-5,7-dihydroxy-2-(3,4,5trihydroxyphenyl)chroman-3-yl 3,4,5-trihydroxybenzoate ( Fig. 1 ), is the most abundant composition of the tea catechins, account for 65% of the total catechin content and is thought to be responsible for the majority of biological activity of green tea extracts ( ). In terms of bioavailability, EGCG is predominantly absorbed in the intestine and presented more than 77% in a free form in plasma as well as its metabolites excreted in urine after oral administration ( ; ; ). The half-life of EGCG in a purified form is around 3 h ( ). Meanwhile, it is well documented that EGCG is able to cross the blood-brain barrier (BBB) and can reach to nervous tissue even at a very low concentration, the first requirement of a dietary compound to apply neuroprotective effects ( ; ). Several experimental studies have shown that EGCG can afford neuroprotection against brain ( ) and spinal cord ( ) injuries, neurodegenerative diseases ( ), and peripheral nerve injuries ( ). These beneficial effects have been mainly attributed to free radical scavenging or anti-oxidant, anti-inflammatory, and anti-apoptotic properties of EGCG ( ; ; ). In this regard, it is well known that green tea catechins due to the hydroxyl groups on the B- and D-rings of the galloylated catechins can bind to the free radicals and neutralized those ( ). On the other hand, scavenging effects of EGCG lead to attenuation of nuclear factor kappa B (NF-κB) activity ( ), which regulates genes involved in inflammatory processes such as tumor necrosis factor alpha (TNFα), cyclooxygenase-2 (COX-2), and interleukin-1 beta (IL-1β), beside modulation of nitric oxide synthase isoforms ( ). Also, catechins have proven to modulate apoptosis at various points in the sequence and messengers, and so regulate the mitochondrial membrane permeabilization ( ; ).

In this chapter, we have reviewed the neuroprotective effects of EGCG and its molecular mechanisms responsible for the neuroprotection following spinal cord injury (SCI). Meanwhile, we also compared the neuroprotective effects of EGCG in SCI to other neurological disorders.
In vivo studies
SCI is a complex multifactorial process caused first by ischemia or mechanical trauma and then by various mechanisms of secondary injury ( ). The neurological outcome of SCI depends on the extent of secondary cellular, molecular, and biochemical cascades such as oxygen free radical-induced lipid peroxidation, inflammatory reaction, and apoptosis ( ; ; ). In recent years, much attention has been paid to secondary injuries, as they appear to be prone to therapeutic interventions that may include the use of natural anti-oxidants, anti-inflammatory, and anti-apoptotic agents ( ) such as EGCG ( Table 1 ). In this regard, our previous studies have shown that intraperitoneal injection of EGCG (50 mg/kg, i.p., immediately and 1 h after SCI) can protect spinal cord tissue and improve behavioral function after traumatic and ischemia-reperfusion injury (IRI) in rats ( ; ; ). In regard to mechanisms of neuroprotective effects of EGCG, our results revealed that malodialdehyde (MDA) levels as an index of tissue lipid peroxidation, an important pathologic event in post-traumatic neuronal degeneration, were significantly decreased in traumatized spinal cord tissue after EGCG treatment ( ). It is well documented that EGCG due to hydroxyl groups can bind to the free radicals and neutralize those, while can indirectly increase the body’s endogenous antioxidants ( ). We detected immunohistochemically anti-apoptotic properties of EGCG with decreasing pro-apoptotic protein Bax and increasing anti-apoptotic protein Bcl-2 staining in EGCG-treated rats, which was correlated with terminal deoxynucleotidyl transferase dUTP nick end labeling (TUNEL) staining method ( ). Also, our other study showed that EGCG treatment (50 mg/kg, i.p., immediately and 1 h after SCI) attenuated pro-inflammatory cytokines such as TNFα and IL-1β in traumatized spinal cord tissue ( ). In line with the anti-inflammatory properties of EGCG, documented that EGCG treatment (100 mg/kg, between 24 and 72 h after SCI) decreased spinal tissue edema through down-regulation of astrocyte expressing aquaporin-4 (AQP4), which plays a critical role in the transport of water from blood/CSF to spinal cord parenchyma, and through down-regulation of glial fibrillary acidic protein (GFAP) as a specific marker of astrocytes. Also, another study found that attenuated NF-κB pathway is a pivotal anti-inflammatory affect of EGCG (intra-spinal injection of 50 mg/kg EGCG immediately and then weekly for up to 28 days) after SCI ( ). Meanwhile, this study showed a significant increase in the gene expression of fibroblast growth factor 2 (FGF2) and vascular endothelial growth factor (VEGF) after SCI. documented that EGCG (100 mg/kg, i.p., immediately after SCI) can protect secondary SCI by potential mechanism of regulating p38MAPK\NF -κB\AQP4 signaling pathway and thus reduce edema after SCI in rats. Also, another study documented that intrathecal administration of EGCG (10 or 20 mg/kg immediately after SCI) can significantly improve locomotors recovery, which may be related to the inhibition of Bcl-2-associated X protein (Bax) and to the up-regulation of brain-derived neurotrophic factor (BDNF) and glial cell line-derived neurotrophic factor (GDNF) ( ). Studies have shown that EGCG has beneficial effects not only on the tissue destruction but also on behavioral and functional outcome, so that intravenous infusion of EGCG (20 mg/kg/hour for continuous 36 h) in acutely (initiated within 4 h post-trauma 0 time) and chronically (initiated after 12 months of SCI onset) spinal cord injured rats improved significantly motor and sensory functions measured using Basso-Beattie-Bresnehan behavioral score test, Louisville forced swim test, and pain behavior assessment tests ( ). One of the common complications of SCI is neuropathic pain. About the effect of EGCG on neuropathic pain, documented that short time EGCG treatment (30 mg/kg i.p., 30 min after and daily during the first week post-SCI) reduced thermal hyperalgesia and gliosis via ras homologue gene family member A (RhoA) and fatty acid synthase (FASN) pathway. In this regard, it is mentioned that some biological properties of EGCG are attributed to its inhibitory action on FASN ( ). In another study, the synergistic effects of curcumin and EGCG in an animal model of acute SCI were investigated ( ). Results of this study revealed that combination of curcumin and EGCG reduced glial scar formation, increased axonal sprouting, and changed the amount of macrophage inflammatory protein 1-alpha (MIP-1α), IL-1β, interleukin-4 (IL-4), and interleukin-6 (IL-6). Recently, our laboratory assessed the neuroprotective effects of EGCG on spinal cord IRI in rats ( ). The level of MDA was significantly reduced in EGCG-treated rats. Attenuated caspase-3 ( Fig. 2 ), TNFα ( Fig. 3 ), and inducible nitric oxide synthase (iNOS) expression could be significantly detected in the EGCG-treated rats. Also, EGCG reduced the extent of degeneration of the spinal cord neurons, in addition to a significant reduction of motor deficit index (MDI). Overall, the behavioral, biochemical, and histopathological evidences demonstrated that pre- (50 mg/kg, i.p., before IRI) and post-ischemic (50 mg/kg, i.p., after IRI) treatment with EGCG had protective effects against spinal cord IRI in rats.
Model of injury | Treatment schedule | Finding | Possible mechanism | Author | Year |
---|---|---|---|---|---|
Weight-drop method | 50 mg/kg, intraperitoneal, immediately and 1 h after spinal cord injury | Decreased bcl-2-associated X protein and increased b-cell lymphoma 2 expression | Anti-apoptotic activity | Khalatbary et al. | 2010 |
Weight-drop method | 50 mg/kg, intraperitoneal, immediately and 1 h after spinal cord injury | Decreased tissue lipid peroxide level | Anti-oxidant activity | Khalatbary and Ahmadvand | 2010 |
Weight-drop method | 50 mg/kg, intraperitoneal, immediately and 1 h after spinal cord injury | Attenuated tumor necrosis factor alpha, interleukin-1 beta, Nitrotyrosine, inducible nitric oxide synthase, cyclooxygenase-2 and poly(ADP-ribose) polymerase expression, and decreased myeloperoxidase activity | Anti-inflammatory activity | Khalatbary and Ahmadvand | 2011 |
Vascular clip method | 100 mg/kg, between 24 and 72 h after spinal cord injury | Down-regulated astrocyte expressing aquaporin-4 and glial fibrillary acidic protein | Anti-edema effect | Ge et al. | 2013 |
Vascular clip method | 100 mg/kg, intraperitoneal, immediately after spinal cord injury | Reduced the releasing of tumor necrosis factor alpha and interleukin-1 beta, decreased the water content | Regulating p38MAPK\NF -κB\AQP4 signaling pathway | Tao and Zhu | 2013 |
Weight-drop method | Intrathecal administration of 10 or 20 mg/kg, immediately after spinal cord injury | Improved locomotor function | Up-regulation of brain-derived neurotrophic factor and glial cell line-derived neurotrophic factor | Tian et al. | 2013 |
Weight-drop method | 20 mg/kg/hour for continuous 36 h, intravenous, in acutely (initiated within 4 h post-trauma 0 time) and chronically (initiated after 12 months of spinal cord injury onset | Improved motor and sensory functions | Reduced lesion size area | Renno et al. | 2014 |
Weight-drop method | 30 mg/kg, intraperitoneal, 30 min after and daily during the first week post-spinal cord injury | Reduced thermal hyperalgesia and gliosis | Via RhoA and FASN pathway | Álvarez-Pérez et al. | 2016 |
Balloon compression method | Intra-spinal injection of 50 mg/kg, immediately and then weekly for up to 28 days | Attenuated nuclear Factor Kappa B pathway and increased gene expression of fibroblast growth factor 2 and vascular endothelial growth factor | Enhanced neuroregeneration | Urdzikova et al. | 2017 |
Weight-drop method | 50-mg/kg, intraperitoneal, before and after ischemia-reperfusion injury | Decreased malodialdehyde, attenuated caspase-3, tumor necrosis factor alpha, and inducible nitric oxide synthase, reduction of motor deficit index | Anti-inflammatory and anti-apoptotic activity | Ahadi et al. | 2019 |


In vitro studies
A number of in vitro studies regarding EGCG’s neuroprotection and its underlying molecular mechanisms in neural cells are starting to accumulate which summarized in Table 2 .
Model of induction | Cell type | Finding | Possible mechanism | Author | Year |
---|---|---|---|---|---|
Apoptosis | PC12 | Inhibited apoptosis | Modulation of cytochrome c and caspases, activation of phosphatidylinositol 3-kinase and survival-promoting pathways, inhibition of glycogen synthase kinase-3 | Nie et al., Koh et al., Mandel et al., Jung et al., Hou et al., Strividhya and Kalaisali | 2002a, 2002b, 2003, 2003, 2007a, 2007b, 2008, 2013 |
Apoptosis | SH-SY5Y | Inhibited apoptosis | Inhibition of reactive oxygen species production and caspase activity, activation of protein kinase C | Levites et al., Jeong et al., Avramovich-Tirosh et al., Tia and Truong | 2002, 2004, 2007, 2010 |
Apoptosis | N18D3 | Attenuated apoptosis | Regulation of phosphatidylinositol 3-kinase and modulation of pro- and anti-apoptotic genes | Zhang et al. | 2014 |
Apoptosis | Primary neuron cells | Attenuated apoptosis | Inhibition of Bcl-2-associated X protein and cytochrome c translocation and autophagic pathways | Lee et al. | 2015 |
Apoptosis | SH-SY5Y | Reduced apoptosis | Inhibiting endoplasmic reticulum stress-mediated apoptosis | Du et al. | 2018 |
Microglial activation | SH-SY5Y | Inhibited LPS-activated microglial secretion of nitric oxide and tumor necrosis factor alpha | Down-regulation of inducible nitric oxide synthase and tumor necrosis factor alpha expression | Lee et al. | 2004 |
Oxidative stress | Neuronal cells culture | Up-regulated superoxide dismutase and catalase levels, decreased malodialdehyde and carbonyl levels, and advanced glycation end products formation | Antioxidative properties and interfering with AGEs-RAGE interaction mediated pathways | Lee and Lee | 2007 |
Oxidative stress | PC12 | Decreased reactive oxygen species production | via the SIRT1/PGC-1α signaling pathway | Ye et al. | 2012 |
Oxidative stress | PC12 | Inhibited the cytotoxicity of acrylamide and increased the cell viability | Without providing possible mechanisms | Esmaeelpanah et al. | 2018 |
Inflammation | SH-SY5Y and ARPE-19 | Anti-inflammatory effects | Suppression of tumor necrosis factor alpha signaling via the nuclear Factor Kappa B pathway | Li et al., Fu and Koo | 2004, 2006 |
Neurogenesis | PC12 | Potentiated nerve growth factor- and brain derived neurotrophic factor-induced neuritogenesis | Involvement of sub-lethal levels of reactive oxygen species or dependent on 67 LR and H2O2 | Gundimeda et al. | 2010, 2014 |
In regard to anti-apoptotic effects of EGCG, some studies indicated that EGCG led to significant inhibition of apoptosis in PC12 cells by scavenging reactive oxygen species (ROS), modulating cytochrome c and caspases, activation of phosphoinositide 3-kinase (PI3K), activation of survival-promoting pathways, and inhibition of glycogen synthase kinase-3 (GSK-3) ( ; ; ; ; ; ; ; ). Also, studies showed that EGCG has a protective effect on cell death in SH-SY5Y (or SHSY-5Y) cell line through inhibiting ROS production and caspase activity along with activation of protein kinase C (PKC) ( ; ; ; ). EGCG protects N18D3 neural cells from quinolinic acid-induced apoptosis by regulation of phosphatidyl inositol 3-kinase (PI3K) and modulation of pro- and anti-apoptotic genes due to decreased intra-cellular calcium levels and nitric oxide (NO) production ( ). Results of a study showed that EGCG protects the neuronal cells against human prion protein-induced damage through inhibiting Bax and cytochrome c translocation and autophagic pathways in primary neuron cells ( ). Recently, it was documented that EGCG reduced amyloid beta-induced neurotoxicity via inhibiting endoplasmic reticulum stress-mediated apoptosis in SH-SY5Y cells in a dose-dependent manner which is contributed to the down-regulation of cleaved-caspase-3 and -12 ( ).
In regard to microglial activation, a study indicated that EGCG exerts significant protection against microglial activation-induced neuronal injury through the down-regulation of iNOS and TNFα expression ( ).
In regard to antioxidant properties of EGCG, in vitro study revealed that EGCG treatment decreased MDA level, up-regulated superoxide dismutase (SOD) and catalase (CAT) levels against advanced glycation end products (AGEs)-induced injury in neuronal cell culture ( ). Also, it was documented that EGCG decreased markedly ROS production via the SIRT1/PGC-1α signaling pathway in 1methyl4-phenyl-pyridine (MMP +)-induced PC12 cells damage ( ). Evaluation of EGCG’s effects on acrylamide-induced cytotoxicity in PC12 cells showed that EGCG inhibited the cytotoxicity of acrylamide and increased the cell viability ( ).
In regard to anti-inflammatory properties of EGCG, in vitro studies also revealed that EGCG treatment potently inhibited lipopolysaccharide (LPS)-activated microglial secretion of TNFα and significantly exerted anti-inflammatory effects partly as a suppressor of TNFα signaling via the NF-κB pathway in SH-SY5Y ( ) and RPE cells ( ).
It is well known that anti-oxidants can also affect the stem cells differentiation and potency. In this regard, using the PC12 cell model showed for the first time that low concentration of EGCG and unfractionated green tea polyphenols potentiate nerve growth factor (NGF)-induced and BDNF-induced neuritogenesis likely through induction sub-lethal levels of ROS or dependent on 67 kDa laminin receptor (67LR) and H 2 O 2 ( ; ).
Applications to other areas of neuroscience
It is well known that EGCG has neuroprotective effects in another neurological disorder such as brain injury, nerve injury, and neurodegenerative diseases ( Table 3 ).
Model of injury | Treatment schedule | Finding | Possible mechanism | Author | Year |
---|---|---|---|---|---|
Brain injury | 50 mg/kg, intraperitoneal, immediately after ischemia | Deoxidizing peroxynitrate/peroxynitrite without affecting blood flow | Attenuation of NO − 3 /NO − 2 | Nagai et al. | 2002 |
Brain injury | 25 mg and 50 mg/kg, intraperitoneal, immediately after ischemia | Attenuated malodialdehyde level and oxidized/total glutathione ratio | Antioxidant effects | Choi et al. | 2004 |
Brain injury | 50 mg/kg, intraperitoneal, immediately after ischemia | Reduced neuronal damage | Reduction of matrix metalloproinase-9 activity | Park et al. | 2010 |
Brain injury | 40 mg/kg, intraperitoneal, once daily for three consecutive days prior to surgery | Reduced neuronal damage | Activation of nuclear erythroid 2-related factor 2 /antioxidant response element signaling | Han et al. | 2014 |
Brain injury | 50 mg/kg, intraperitoneal, immediately after surgery and once daily till day 7 | Promoted angiogenesis | Up-regulation of the nuclear erythroid 2-related factor 2 signaling pathway | Bai et al. | 2017 |
Brain injury | 50 mg/kg, intraperitoneal, immediately after ischemia | Modulated inflammatory responses | Amelioration of tumor necrosis factor alpha, interleukin-1 beta, interleukin-6, nuclear factor kappa B /p65, cyclooxygenase-2, inducible nitric oxide synthase | Zhang et al. | 2015 |
Brain injury | 50 mg/kg, daily for 1 day and 1 h before ischemia | Reduced nitric oxide synthase and inducible nitric oxide synthase , increased endothelial nitric oxide synthase, preserved complex I–V | Complex signal transduction mechanisms | Sutherland et al. | 2005 |
Brain injury | Consuming 0.1% ( w / v ) epigallocatechin-3-gallate drinking water ad libitum before and after traumatic injury | Increased neural stem cells number around the damaged area | Antioxidant and antiapoptotic activities | Itoh et al. | 2011, 2013, 2012 |
Brain injury | 100 mg/kg, intraperitoneal, after traumatic injury | Reduced interleukin-1 beta and tumor necrosis factor alpha mRNA expression, inhibited astrocyte expressing aquaporin-4 and glial fibrillary acidic protein, elevated superoxide dismutase and glutathione peroxidase activities, decreased nicotinamide adenine dinucleotide phosphate oxidase activation | Inhibition of inflammation and oxidative stress | Zhang et al. | 2015 |
Nerve injury | 25 mg/kg, injected daily for 5 days and 2 mg/kg orally daily afterwards | Increased density of retinal ganglion cells | Up-regulation of neurofilament triplet L protein expression | Ahadi et al. | Xie 2010 |
Nerve injury | 25 mg/kg, intraperitoneal or orally, daily for 5 days and 2 mg/kg orally daily afterwards | Enhanced motor neuron survival time | Attenuation of nicotinamide adenine dinucleotide phosphate-diaphorase-d/neuronal nitric oxide synthase | Wei et al. | 2011 |
Nerve injury | 1 mg/kg, intrathecal, once daily from 1 day before to 3 days after chronic constriction injury | Decreased chronic neuropathic pain | Down-regulation of Toll-like receptor 4, nuclear factor kappa B, high-mobility group box 1 protein, tumor necrosis factor alpha and interleukin-1 beta protein expression | Kuang et al. | 2012 |
Nerve injury | 50 mg/kg, intraperitoneal, following rat sciatic nerve crush injury | Enhanced functional recovery and accelerated nerve regeneration | Down-regulation of apoptosis related genes, up-regulation of survivin gene, and up-regulation of glutathione reductase | Renno et al. | 2017, 2015 |
Nerve injury | 50 mg/kg, intraperitoneal, starting 1 h after sciatic nerve crush injury | Modulated brain-derived neurotrophic factor, glial cell line-derived neurotrophic factor and neurotrophin-3 and their receptors tropomyosin receptor kinase B, tropomyosin receptor kinase C, and nerve growth factor receptor p75 | Up-regulation of neurotrophic factors and their receptors | Renno et al. | 2016 |
Nerve injury | 50 mg/kg, intraperitoneal, 1 h after nerve transaction and followed for 3 days | Reduced neural apoptosis | Modulation of malodialdehyde levels, catalase and superoxide dismutase activities, and Caspase3 and cyclooxygenase-2 expression | Kian et al. | 2019 |
Alzheimer’s disease | 20 mg/kg, intraperitoneal, daily for 60 days | Effective prophylaxis for Alzheimer’s disease | Modulation of amyloid precursor protein cleavage and cerebral amyloidosis | Rezai-Zadeh et al. | 2005 |
Neurodegenerative disease | 2 or 6 mg/day for 4 weeks, intragastrically | Improved degenerative changes of the brain | Modulation of beta-amyloid and amyloid precursor protein | He et al. | 2012 |
Neurodegenerative disease | 2 mg/kg/day for 4 weeks, intragastrically | Ameliorated learning and memory deficits | Modulation of TrkA/p75 NTR signaling and apoptosis | Liu et al. | 2014 |
Parkinson’s disease | No available | Alleviated dopaminergic neuronal injury in Parkinson’s disease | Inhibition of nitric oxide and tumor necrosis factor alpha | Li et al. | 2004 |
Parkinson’s disease | 25 mg/kg, oral administration for 7 days | Neurorescue effects in Parkinson’s disease | Regulation of the iron-export protein ferroportin and reduction of oxidative stress | Xu et al. | 2017 |
Neurodegenerative disease | 300 μg, oral gavage twice daily | Delayed disease onset, induced regeneration of hippocampal axons | Reduction of the inflammatory infiltrates | Herges et al. | 2011 |
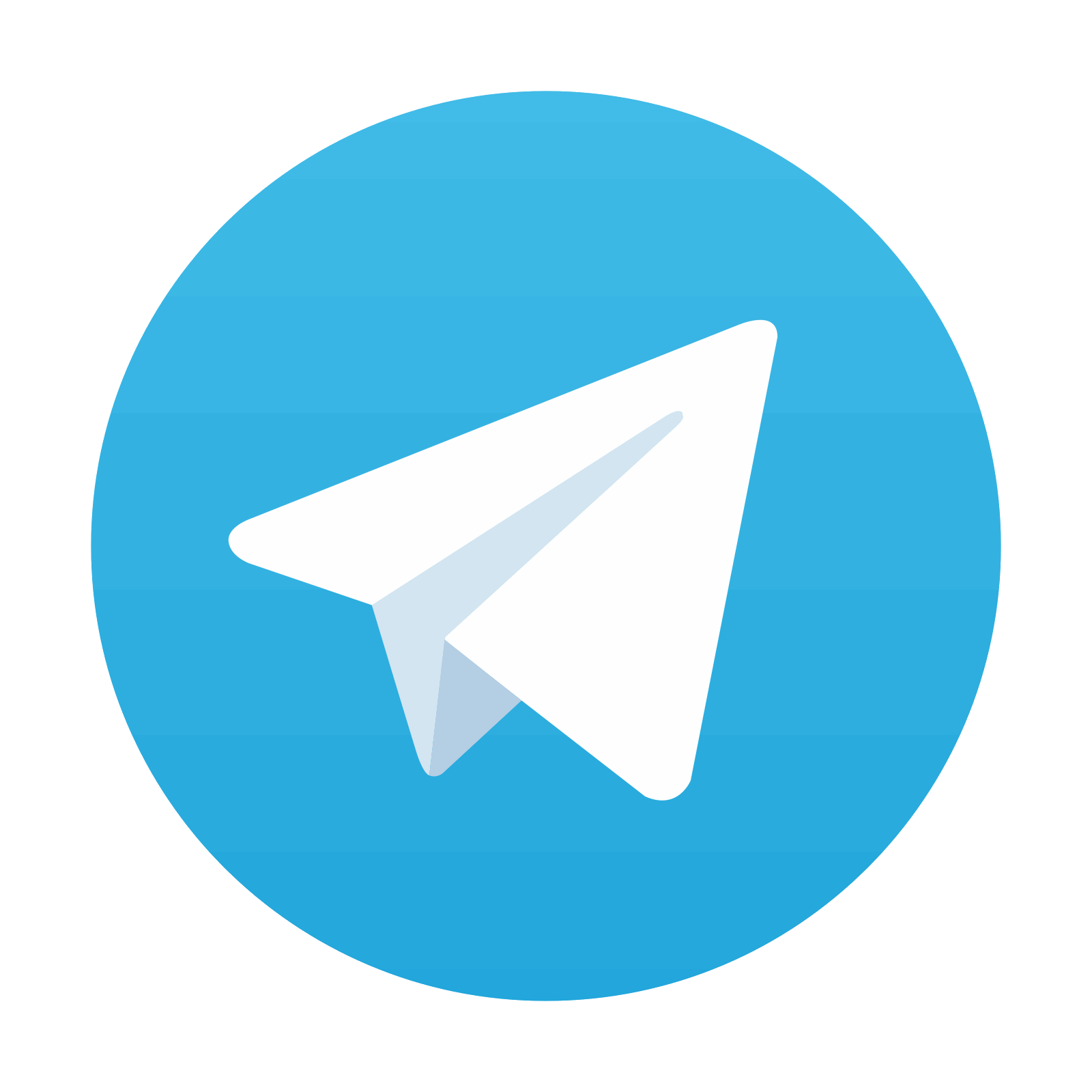
Stay updated, free articles. Join our Telegram channel

Full access? Get Clinical Tree
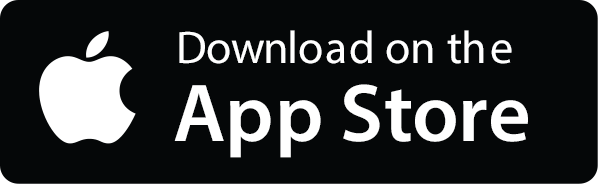
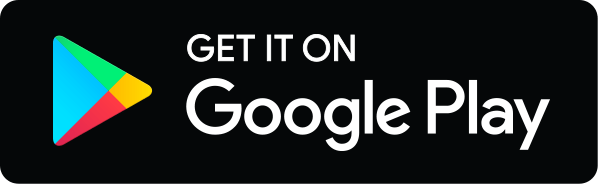