Fig. 11.1
The target task is to rotate two cork balls as fast as possible in a counterclockwise direction with the left hand. The dexterity of the motor performance of the left hand was examined by counting the number of two-ball rotations during 30 s. After the experiments, one of the experimenters, who was blind to the participants’ status, reviewed the video recording and counted the number of rotations. In addition to behavioral parameters, MEP amplitudes were measured by TMS to assess corticospinal excitability before and after intervention (a). It shows that the number of ball rotations performed by the left hand and the mean MEP amplitude increased significantly after the MVF condition (b). Correlation between M1 plasticity and behavioral improvement were significantly correlated (c)
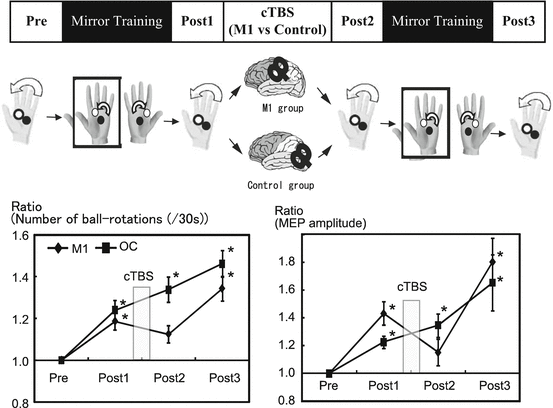
Fig. 11.2
We used cTBS to provide functional interference over the right M1 or occipital area as control to confirm the functional relevance of the M1 in the motor improvement induced by MVF. The cTBS was applied just after MVF intervention. For cTBS, TMS was delivered as 200 bursts of three pulses at 5 Hz given in a continuous train and an intensity of 80 % active motor threshold. The results showed that the number of ball rotations for the cTBS at the M1 creased significantly after the first and second MVF condition (Post1 and Post3), but not after cTBS (Post2). The mean MEP amplitude revealed a similar pattern of behavior, with significant increases at Post1 and Post3 compared with Pre
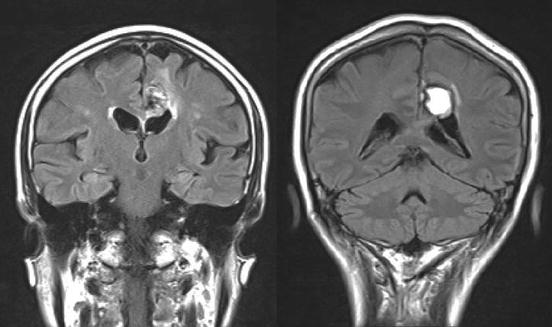
Fig. 11.3
Two patients with corpus callosum lesion participated in our study. These two patients showed impairment in test of cross-localization of finger tips (CLF), which constitute the hallmark of the interhemispheric disconnection syndrome
The potential role of somatosensory stimulation as a means to dynamically modulate the organization of the motor cortex has been postulated during the past years [16, 93–95]. It has been reported that a short period of electrical stimulation is sufficient to trigger changes in the M1 representation in neurologically intact individuals [96–98]. Further exploration has strengthened the understanding that electrical peripheral nerve stimulation is a powerful tool to induce sustained excitability increases as well as rapidly evolving neuroplastic changes of the human motor cortex [99, 100]. Passive movement is also applied as a proprioceptive stimulation in neurorehabilitation. This technique of somatosensory stimulation has been found to generate cortical activity in both sensory and motor cortical areas in humans [101, 102] and/or induce persistent neuroplastic changes of the sensorimotor representation when administered repeatedly.
In rehabilitation field, some new interventions to optimize training strategies after brain lesion have been proposed in the past few years such as constraint-induced movement therapy [103–105], bilateral arm training [106], mirror therapy [70, 71], and robotic-based approached [107–109]. Additionally, other new technical approaches have been suggested to facilitate the beneficial effects of training on motor learning in the setting of rehabilitation such as transcranial magnetic stimulation (TMS) and transcranial direct current stimulation (tDCS). These noninvasive brain stimulation techniques have been used to explore possible causal relations between activity in specific brain areas and particular behaviors [110, 111]. Moreover, knowledge of the relationship of a brain region in a type of behavior was executed by attempts to modulate activity in specific cortical areas with the goal to enhance motor [112, 113] and cognitive [114–116] performance.
References
1.
Doyon J, Benali H (2005) Reorganization and plasticity in the adult brain during learning of motor skills. Curr Opin Neurobiol 15(2):161–167PubMed
2.
Karni A et al (1995) Functional MRI evidence for adult motor cortex plasticity during motor skill learning. Nature 377(6545):155–158PubMed
3.
Fischer S et al (2005) Motor memory consolidation in sleep shapes more effective neuronal representations. J Neurosci 25(49):11248–11255PubMed
4.
Korman M et al (2003) Multiple shifts in the representation of a motor sequence during the acquisition of skilled performance. Proc Natl Acad Sci U S A 100(21):12492–12497PubMedCentralPubMed
5.
Walker MP et al (2002) Practice with sleep makes perfect: sleep-dependent motor skill learning. Neuron 35(1):205–211PubMed
6.
Muellbacher W et al (2002) Early consolidation in human primary motor cortex. Nature 415(6872):640–644PubMed
7.
Robertson EM, Pascual-Leone A, Miall RC (2004) Current concepts in procedural consolidation. Nat Rev Neurosci 5(7):576–582PubMed
8.
Doyon J et al (2009) Contributions of the basal ganglia and functionally related brain structures to motor learning. Behav Brain Res 199(1):61–75PubMed
9.
Butefisch CM et al (2000) Mechanisms of use-dependent plasticity in the human motor cortex. Proc Natl Acad Sci U S A 97(7):3661–3665PubMedCentralPubMed
10.
Donoghue JP (1995) Plasticity of adult sensorimotor representations. Curr Opin Neurobiol 5(6):749–754PubMed
11.
Jenkins WM et al (1990) Functional reorganization of primary somatosensory cortex in adult owl monkeys after behaviorally controlled tactile stimulation. J Neurophysiol 63(1):82–104PubMed
12.
Wang X et al (1995) Remodelling of hand representation in adult cortex determined by timing of tactile stimulation. Nature 378(6552):71–75PubMed
13.
Nudo RJ et al (1996) Use-dependent alterations of movement representations in primary motor cortex of adult squirrel monkeys. J Neurosci 16(2):785–807PubMed
14.
Donoghue JP, Suner S, Sanes JN (1990) Dynamic organization of primary motor cortex output to target muscles in adult rats. II Rapid reorganization following motor nerve lesions. Exp Brain Res 79(3):492–503PubMed
15.
Sanes JN, Wang J, Donoghue JP (1992) Immediate and delayed changes of rat motor cortical output representation with new forelimb configurations. Cereb Cortex 2(2):141–152PubMed
16.
Brasil-Neto JP et al (1993) Rapid modulation of human cortical motor outputs following ischaemic nerve block. Brain 116(Pt 3):511–525PubMed
17.
Cohen LG et al (1993) Plasticity of cortical motor output organization following deafferentation, cerebral lesions, and skill acquisition. Adv Neurol 63:187–200PubMed
18.
Ziemann U, Corwell B, Cohen LG (1998) Modulation of plasticity in human motor cortex after forearm ischemic nerve block. J Neurosci 18(3):1115–1123PubMed
19.
Jeannerod M, Frak V (1999) Mental imaging of motor activity in humans. Curr Opin Neurobiol 9(6):735–739PubMed
20.
Sharma N, Pomeroy VM, Baron JC (2006) Motor imagery: a backdoor to the motor system after stroke? Stroke 37(7):1941–1952PubMed
21.
Jeannerod M (2001) Neural simulation of action: a unifying mechanism for motor cognition. Neuroimage 14(1 Pt 2):S103–S109PubMed
22.
Gerardin E et al (2000) Partially overlapping neural networks for real and imagined hand movements. Cereb Cortex 10(11):1093–1104PubMed
23.
Lotze M et al (1999) Activation of cortical and cerebellar motor areas during executed and imagined hand movements: an fMRI study. J Cogn Neurosci 11(5):491–501PubMed
24.
Porro CA et al (1996) Primary motor and sensory cortex activation during motor performance and motor imagery: a functional magnetic resonance imaging study. J Neurosci 16(23):7688–7698PubMed
25.
Roth M et al (1996) Possible involvement of primary motor cortex in mentally simulated movement: a functional magnetic resonance imaging study. Neuroreport 7(7):1280–1284PubMed
26.
de Lange FP, Hagoort P, Toni I (2005) Neural topography and content of movement representations. J Cogn Neurosci 17(1):97–112PubMed
27.
Hanakawa T et al (2003) Functional properties of brain areas associated with motor execution and imagery. J Neurophysiol 89(2):989–1002PubMed
28.
Parsons LM et al (1995) Use of implicit motor imagery for visual shape discrimination as revealed by PET. Nature 375(6526):54–58PubMed
29.
Fadiga L et al (1995) Motor facilitation during action observation: a magnetic stimulation study. J Neurophysiol 73(6):2608–2611PubMed
30.
Hari R et al (1998) Activation of human primary motor cortex during action observation: a neuromagnetic study. Proc Natl Acad Sci U S A 95(25):15061–15065PubMedCentralPubMed
31.
Rizzolatti G, Fogassi L, Gallese V (2002) Motor and cognitive functions of the ventral premotor cortex. Curr Opin Neurobiol 12(2):149–154PubMed
32.
Hanakawa T, Dimyan MA, Hallett M (2008) Motor planning, imagery, and execution in the distributed motor network: a time-course study with functional MRI. Cereb Cortex 18(12):2775–2788PubMedCentralPubMed
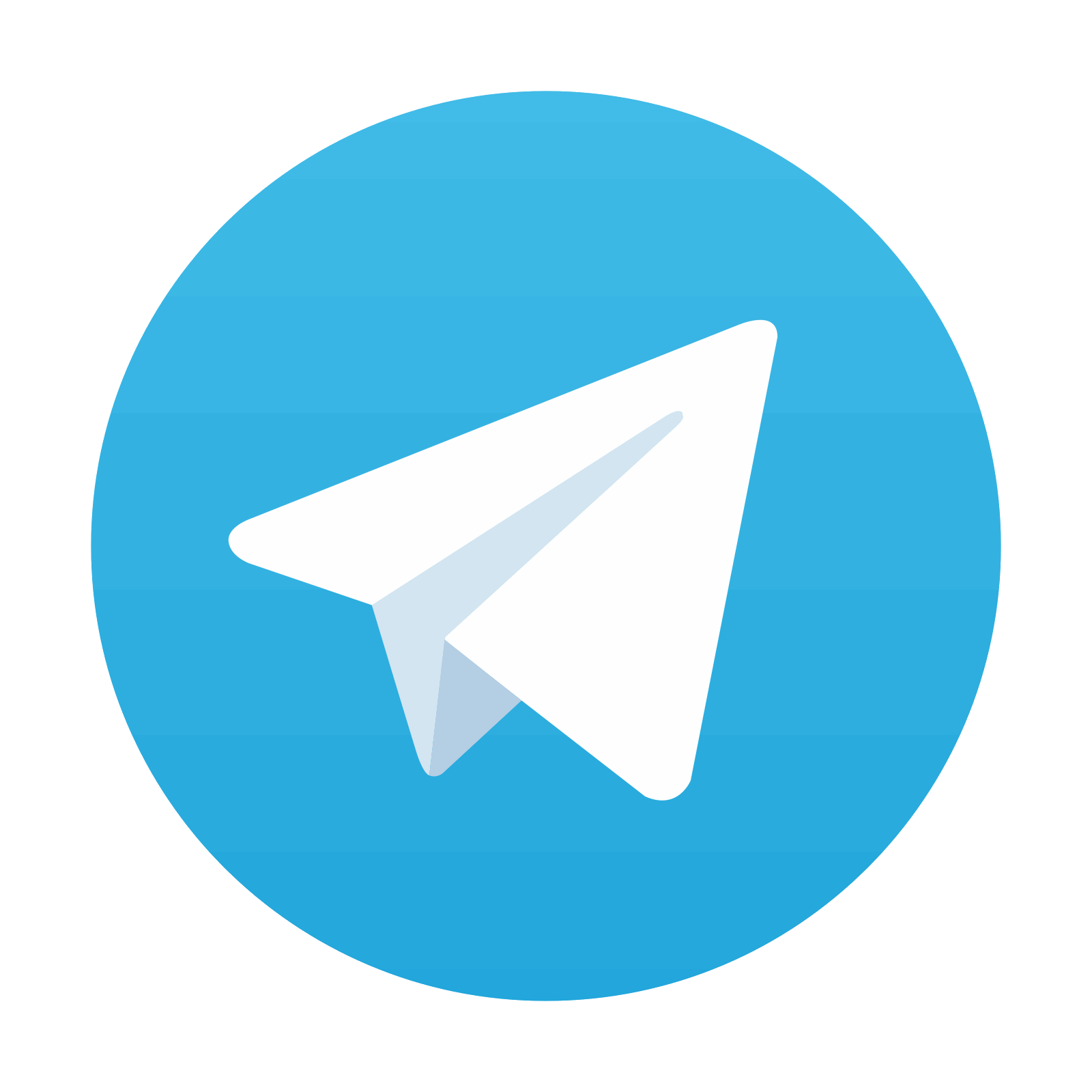
Stay updated, free articles. Join our Telegram channel

Full access? Get Clinical Tree
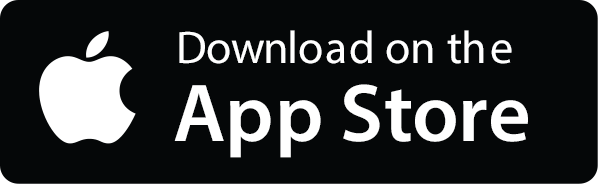
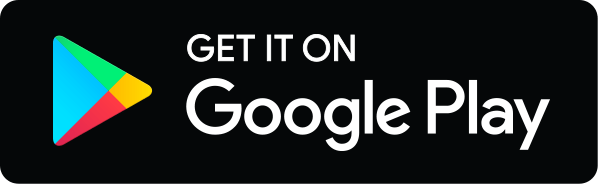