16 Vagus Nerve Stimulation Vagal nerve stimulation was first reported as potentially having efficacy in three out of four seizure patients in 1990,1 and it was approved by the Food and Drug Administration for the treatment of medically refractory epilepsy in 1997. As of November 2006, 45,000 patients have received the VNS Therapy System (Cyberonics*, Inc., Houston, TX) pulse generator attached to silicon-coated leads with contact electrodes on the vagus nerve.2 This chapter reviews the history of vagus nervous system stimulation for the treatment of epilepsy, our limited understanding of its mechanism of action, and the indications, surgical technique, management, and outcomes of vagus nerve stimulation for epilepsy. Seizures are episodes of disturbed brain function that cause changes in attention or behavior due to abnormal electrical excitation in the brain. Seizures as a clinical entity were described in Indian, Chinese, and Mesopotamian literature ~3000 years ago. Hippocrates of Galen subsequently postulated that such activity might be due to an excess of phlegm caused by abnormal brain consistency. Epilepsy, defined as two or more seizures, afflicts nearly 3% of the population at some point in their lifetime, though only one-tenth of this number has epilepsy that is active. The consequences of uncontrolled epilepsy are vast and range from physical injury such as contusions, fractures, lacerations, and burns to psychosocial disorders resulting from depression, social withdrawal, embarrassment, decreased independence, and limited employment opportunities.3 Mortality rates are four to seven times greater than in the general population and sudden death can result directly from seizures, or occur as an indirect consequence of severe depression and suicide.3 Of the 0.3% of the population that are active epileptics, two-thirds are controlled by medical therapy, and one-third are refractory even after 18 months of aggressive therapy with two standard antiepileptic agents.4 A percentage of the medically refractory patients will have seizures arising from a focal area that is not in eloquent cortex and thus are candidates for surgical resection of their seizure focus. The remainder of these active epileptics are possible candidates for alternative surgical therapy, such as stimulation. Electrical stimulation of the nervous system as a mechanism for reduction of epilepsy has been practiced for at least 2000 years. In 76 A.D., the Greek herbalist and physician Pedanius Discorides advocated seizure treatment with the electric torpedo ray (Torpedo nobiliana).5 During the 11th century, the Persian physician Ali Abu Ibn-Sina (Latinized by translators to Avicenna) noted efficacy against epilepsy with placement of an electric catfish on the brow of the afflicted.5 The 18th century clergyman and electrotherapist John Wesley, described a patient with a 7-year history of twice daily drop attack seizures he was able to cure with a single electric shock.6 The invention of the Leyden jar, a protoype capacitor, in 1745 by German Ewald Georg van Kleist further enabled the use of electric shock as a means for treating seizures. The Leyden jar was named after the hometown of its second independent inventor in the Netherlands, Pieter van Musschenbroek.7 Altered brain electricity in epilepsy was hypothesized by the 19th century British physician Robert Bentley Todd, perhaps best known for his eponymous postictal paralysis, and his contemporary, electroscientist Michael Faraday.8 John Hughlings Jackson further popularized belief in the electrical basis of epilepsy beginning in 1873.9 British neurophysiologist Richard Caton invented the electroencephalogram (EEG) in 1875, and German psychiatrist Hans Burger performed the first human EEG via scalp recordings in 1924, ultimately enabling visualization of seizure activity.7 The discovery of EEG served as further proof for the electrical basis of nerve conduction, which was confirmed with the Nobel-winning squid action potential work of neurophysiologists Alan Hodgkin and Andrew Huxley in 1952.10 In 1938, stimulation of the severed proximal end of vagus nerve in cats was found to cause cortical EEG changes by Percival Bailey and Frederic Bremer in Chicago.11 Dell and Olson further demonstrated in 1951 that such stimulation of the proximal end of the severed vagus projected to forebrain, thalamus, midbrain, and cerebellum12,13; these projections were found to be extinguished by a tight ligature on the vagus nerve by Zanchetti et al in 1952.14 The mechanism of action of Pranayama, and other yogas postulated to be efficacious against epilepsy, is hypothesized as partially due to respiratory stimulation of the vagus nerve.15 Such respiratory stimulation, also advocated in classes for childbearing women in labor, was allegedly the inspiration for development of the vagal nerve stimulator. The Philadelphia neurophysiologist Jacob Zabara attended such a class with his wife in 197116 and postulated that if mild stimulation of vagal nerve afferents was sufficient to decrease labor pain, then greater stimulation could further suppress the nervous system for other potentially clinical applications. Zabara first applied vagal nerve stimulation to cats to reduce xylazine-induced emesis.17 The second application for his vagal nerve stimulator was to reduce strychnine-induced seizures in dogs.17 Zabara’s experiments were duplicated in other species; vagal nerve stimulation was found to reduce experimentally induced seizures in monkeys18 and rats.19,20 Recognizing the clinical relevance of his work, he partnered with pacemaker designer S. Reese Terry to found Cyberonics, Inc., the manufacturer of vagal nerve stimulators. The first human vagal nerve stimulator was placed by pediatric neurosurgeon William Bell on November 16, 1988. Neurologists J. Kiffin Penry and Christine Dean presented the results from Bell’s patient at a special conference on vagal nerve stimulation at the American Epilepsy Society meeting in Boston in December 1989 where seven other related articles were presented.1 By then a total of 14 patients had been implanted at three centers, and of the nine of these who had been followed for at least 6 weeks, seven had seen a reduction in the number of seizures.1,2 1 Two patients were seizure free at 1 year and there were only minor complications such as temporary hoarseness from the stimulation, and one case of lead breakage. Clinical trials with the vagal nerve stimulator have been performed as crossover trials with the stimulator on or off and as randomized prospective trials with low versus high levels of stimulation.22 Based on the results of clinical trials, the Food and Drug Administration approved the vagal nerve stimulator for the treatment of epilepsy in 1997. Seizures on EEG are represented by abnormal synchronization of neuronal activity. Desynchronization, then, should diminish seizure activity. Vagal nerve stimulation was first hypothesized to work via desynchronization of the EEG.23 Michael Chase at UCLA and colleagues demonstrated in cats that the frequency dependent effect of vagus nerve stimulation on EEG was due to activation of particular fibers at varying frequencies. Vagus stimulation at frequencies above 70 Hz and intensities >3 V were shown to be desynchronizing. If the intensity was dropped to <3 V and only myelinated fibers were stimulated, the EEG would be synchronized. Lower frequencies (20 to 50 Hz) and higher voltages were also shown to be desynchronizing. Desynchronization was shown to be the result of stimulation of vagal fibers that conduct at 1 to 15 m/s.24 Early studies suggested that unlike in animals, vagal nerve stimulation did not affect human EEG regardless of level of alertness.25 On longer follow-up, however, at a mean of 16.8 months after implantation, EEG changes were seen after vagal nerve stimulation with desynchronization dominating over synchronization and diminished interictal spikes.26 In children, a decrease in the number of interictal epileptiform discharges has been seen as early as 3 months after stimulator implantation.27 The vagus nerve, so named for its “wandering” course (Latin), has special visceral efferent fibers that project to the nucleus ambiguus from the pharyngeal and laryngeal muscles. Its general visceral efferent fibers arising from the dorsal motor nucleus innervate the heart, lungs, and viscera. The vagus also has sensory afferent projections, notably from the concha of the ear. The vagus’ visceral afferents travel via the tractus solitarius to the thalamus, amygdale, and fore-brain, and via the medullary reticular formation to other cortical areas28 (reviewed in Rutecki23). The vagus’ diffuse projections mediate several visceral reflexes including the baroreceptive response, coughing, vomiting, and swallowing. Projections to the hypothalamus affect appetite, blood pressure, and volume homeostasis. Projections via the tractus solitarius and medullary reticular formation may be responsible for the antiepileptic impact of vagus nerve stimulation. In rat studies, the hippocampus and striatum are among the epilepsy-mediating areas that have altered metabolism after vagal nerve stimulation.29 Projections to the frontal cortex and cingulate gyrus are highlighted on positron emission studies of patients receiving the vagal nerve stimulator for depression.30 Given the diffuse projections of the vagus nerve and despite understanding some of the properties of stimulation that are most likely to result in EEG desynchronization, we still do not have a clear understanding of the mechanism of vagal nerve stimulation. Decreased excitatory and increased inhibitory neurotransmission31 and changes in cerebral blood flow are observed after both acute and chronic vagal nerve stimulation.32–34 In a rat model, neuronal activity in the locus ceruleus is directly modified by vagal nerve stimulation.35 Chronic stimulation may alter limbic circuitry to reduce epileptogenesis.33 Selim Benbadis and colleagues have generated an algorithm for the management of epilepsy.36 Patients with seizures for 9 months or greater who are refractory to medical treatment by a neurologist should be referred for EEG video monitoring, magnetic resonance imaging (MRI), and confirmation of the diagnosis and type of intractable epilepsy. After this confirmation, patients with nonlocalizable seizures should be considered for vagal nerve stimulation either directly, or after consideration of the ketogenic diet, depending on clinical circumstances. Among patients with localizable lesions resulting in seizures, vagal nerve stimulation should be considered for those who fail surgical resection, or who have bilateral cortical localization.36 Evidence exists to support the use of vagal nerve stimulation earlier rather than later after the onset of epilepsy to maximize its benefit. Epilepsy patients who had tried four or fewer seizure medications or who were implanted with the vagal nerve stimulator within 5 years of onset of the seizure disorder were three times more likely to report no seizures after 3 months of treatment than those who had received the device after failing more medications or waiting longer than 5 years.37 A randomized clinical trial comparing vagal nerve stimulation to standard of care antiepileptic drugs (AEDs) has not been performed. Comparisons in cost accounting for extra hospitalizations due to seizures suggests that the savings of vagal nerve stimulation versus medical management are significant.38–40 An additional advantage of vagal nerve stimulation over medical therapy regards compliance and side-effect profiles. Approximately 75% of patients receiving AEDs discontinue therapy within 5 years,41 whereas only ~20% of vagal nerve stimulation patients discontinue therapy.42 The safety of vagal nerve stimulation for epilepsy at the extremes of age and during pregnancy has not been fully explored. Patients <5 years of age appear to tolerate the stimulation well, and have had excellent reductions in seizures.43 One patient has been reported to have a normal pregnancy outcome after vagal nerve stimulation for depression.44 Vagal nerve stimulator patients appear to tolerate MRI and functional MRI (fMRI) scans without incident; the manufacturer recommends that a transmit and receive head coil be used with a 1.5 T scanner. MRIs have been performed with the stimulator turned on to evaluate the impact of the stimulation.45–51 In one study, the patient had a seizure on the table and diffusion-weighted imaging demonstrated transient ischemia at the focus, which returned to normal at seizure completion.52 Most of the MRI data were obtained on 1.5 T scanners. The technique for vagal nerve stimulation is straightforward for a surgeon experienced in exposure of the cervical carotid artery. We use the technique provided by the device manufacturer, which has minor modifications from the technique originally described by Steven Reid.53 The device components are purchasable as kits from Cyberonics and consist of a titanium-housed pulse generator with a lithium carbon monofluoride battery, and a 43 cm lead wire with two platinum/iridium helical electrodes and a tethering anchor (Figs. 16.1 and 16.2). 54 The electrode and anchor are available in either 2 or 3 mm diameters, and we have always used the smaller of the two; both are associated with a 2-mm lead. There are four models of pulse generator, the most recent of these measures 5.2 x 5.2 x 0.7 cm and uses a single-pin lead. The battery life is estimated at 6 years, but it is affected by stimulation parameters. The VNS lead we currently implant is the model 302–20, which has a single pin for the generator. Earlier generator models were slightly larger and used a double-pin lead. A newer model double-pin pulse generator is still available for replacement in patients with the older lead.
History
Mechanism of Action
Indications
Operative Technique
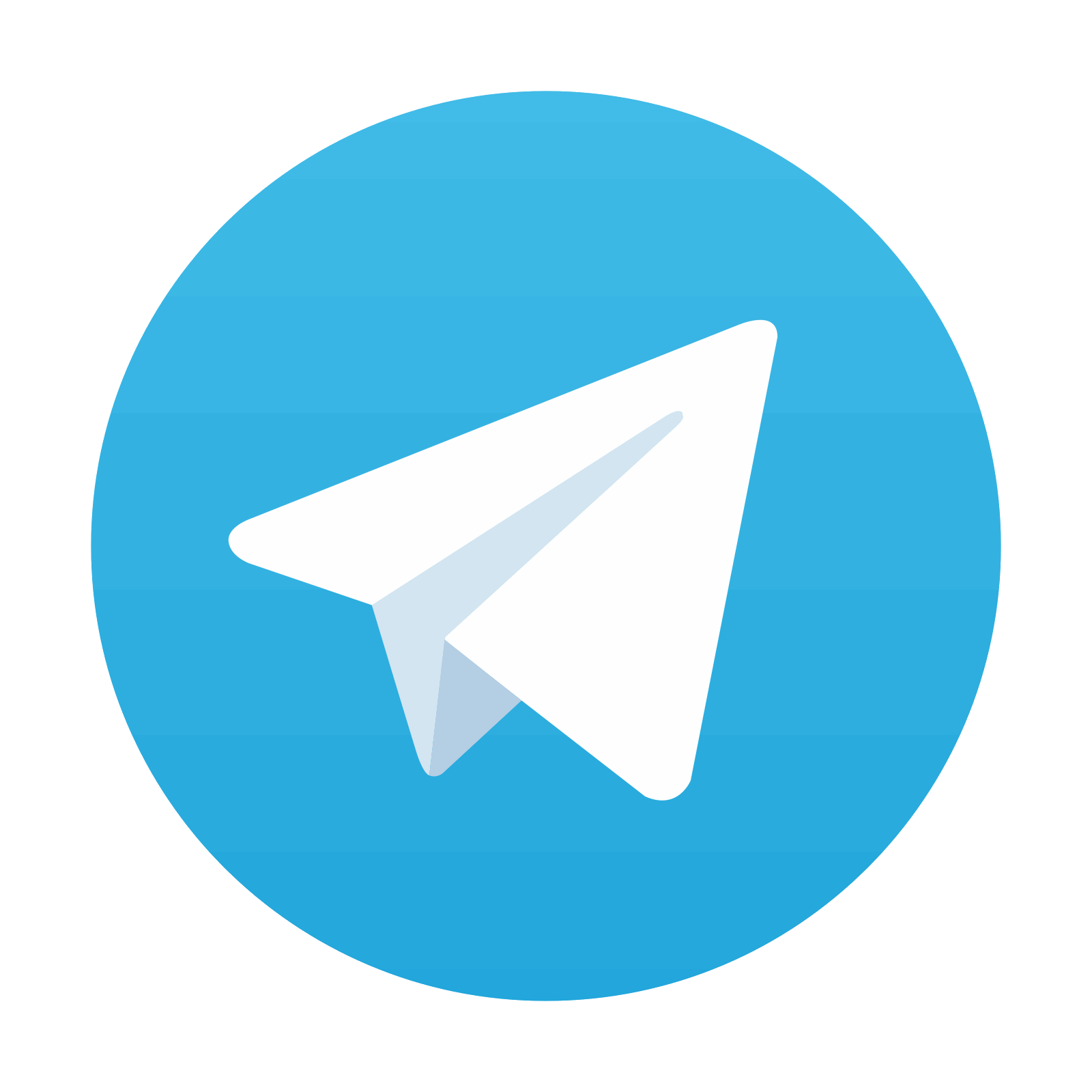
Stay updated, free articles. Join our Telegram channel

Full access? Get Clinical Tree
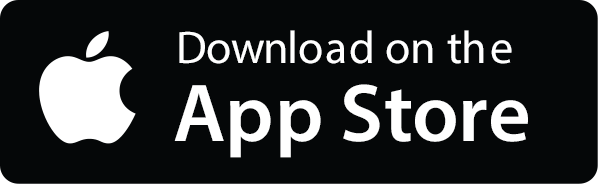
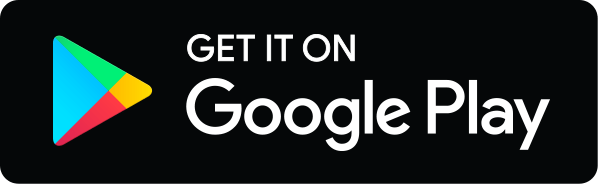