Vascular Malformations
Main Text
Preamble
Brain vascular malformations, a.k.a. cerebrovascular malformations, are a heterogeneous group of disorders that exhibit a broad spectrum of biologic behaviors. Some cerebrovascular malformations (e.g., capillary malformations) are almost always clinically silent and found incidentally on imaging studies. Others, such as arteriovenous malformations and cavernous angiomas, may hemorrhage unexpectedly and without warning.
In this chapter, we begin with an overview of cerebrovascular malformations (CVMs), starting with a discussion of terminology, etiology, and classification. CVMs are grouped according to whether or not they exhibit arteriovenous shunting, and then each type is discussed individually.
Terminology
Two major groups of vascular anomalies in the central nervous system are recognized: Vascular malformations and hemangiomas. All CVMs—the entities considered in this chapter—are nonneoplastic lesions. CVMs are sometimes referred to as angiomas.
In contrast, “hemangiomas” are benign neoplastic vascular lesions that can be isolated, solitary or multiple, or occur as part of a PIK3CA-related overgrowth syndrome. In the most recent WHO classification of CNS tumors, hemangiomas are grouped with other soft tissue tumors and designated as mesenchymal, nonmeningothelial CNS tumors. Hemangiomas are discussed in Chapter 28.
Classification
CVMs have been traditionally classified by histopathology into four major types: (1) Arteriovenous malformations (AVMs), (2) venous angiomas (developmental venous anomalies), (3) capillary telangiectasias (sometimes simply termed “telangiectasia” or “telangiectasis”), and (4) cavernous malformations.
Many interventional neuroradiologists and neurosurgeons group CVMs by function, not histopathology. In this functional classification, CVMs are divided into two basic categories: (1) CVMs that display arteriovenous shunting and (2) CVMs without arteriovenous shunting (Table 7-1). The former are potentially amenable to endovascular intervention; the latter are either treated surgically or left alone.
Cerebrovascular Malformations With Arteriovenous Shunting
Arteriovenous Malformation
Terminology
A brain AVM (BAVM) is a tightly packed tangle of serpiginous, thin-walled vessels without an intervening capillary bed.
Etiology
Genetics
Most BAVMs are solitary and nonsyndromic. Recent evidence suggests that germline and somatic mutations are crucial for AVM development and that aberrant angiogenesis might be the key mechanism. Somatic KRAS mutations activating the MAPK-ERK signaling pathway in brain endothelial cells have recently been identified as playing a key role in BAVM development.
Multiple BAVMs are almost always syndromic. Less than 5% of all BAVMs occur in autosomal dominant inherited genetic syndromes, such as hereditary hemorrhagic telangiectasia (HHT) or capillary malformation-AVM syndrome. Inherited vascular neurocutaneous syndromes are discussed in detail in Chapter 44.
Pathology
Location
Over 85% of AVMs are supratentorial, located in the cerebral hemispheres (7-1). Only 15% are found in the posterior fossa.
Size
AVMs vary from tiny lesions to giant malformations that can occupy an entire cerebral lobe or hemisphere. Most are intermediate in size with a nidus ranging from 2-6 cm in diameter. Both the feeding arteries and draining veins are usually enlarged.
“Micro”-AVMs have a nidus ≤ 1 cm; feeding arteries and draining veins are usually normal in size. Micro-AVMs are typically associated with HHT.
Gross Pathology
AVMs appear as a focal, compact, ovoid or wedge-shaped mass of malformed arteries and veins of varying caliber and thickness that connect through a nidus instead of a normal capillary bed (7-2). Their broadest surface is at or near the cortex with the apex pointing toward the ventricles (7-1). Dilated draining veins are often found on the cortical surface overlying an AVM.
The brain surrounding an AVM often appears abnormal due to ischemic “steal” and tissue rarefaction. Hemorrhagic residua, such as gliosis and secondary ischemic changes, are common, as are siderotic changes in the overlying pia. Occasionally, an AVM presents with large, obliterating hemorrhage, often secondary to rupture of an intranidal aneurysm or sudden, catastrophic thrombosis of outlet draining veins.
Microscopic Features
There are no capillaries within an AVM nidus. Parenchyma within the nidus itself is minimal and gliotic. The nidus contains dysplastic, hyalinized, thin-walled vessels with “arterialized” veins, varying amounts of laminated thrombus, dystrophic calcifications, and hemorrhagic residua.
AVM: ETIOLOGY AND PATHOLOGY
Etiology
• Congenital defect
Abnormal vascular development
Dysregulated angiogenesis
• Genetics
Sporadic BAVMs have somatic activating KRAS mutations
Syndromic BAVMs (e.g., HHT) have known specific mutations
Pathology
• Gross pathology
Ovoid or wedge-shaped with broad base toward cortex
3 components: Feeding arteries, nidus (center), draining veins
• Microscopic features
Dysplastic thin-walled vessels
Ectatic “arterialized” veins
Only nonfunctional gliotic brain in nidus
Clinical Issues
Epidemiology
Almost all AVMs are sporadic and solitary. With very rare exceptions (“de novo” AVMs), most are considered congenital lesions. Sporadic (nonsyndromic) AVMs are found in 0.15% of the general population.
Presentation
Peak presentation occurs between 20-40 years of age, although 25% of patients harboring an AVM become symptomatic by 15 years of age. There is no sex predilection.
Headache with parenchymal hemorrhage is the most common presentation, occurring in ~ 1/2 of all patients. Seizure and focal neurologic deficits are the initial symptoms in 25% each. Small micro-AVMs in patients with vascular neurocutaneous syndromes, such as HHT, are often asymptomatic and only discovered when screening imaging studies are performed.
Natural History
A growing body of gene expression and polymorphism-based research studies support the involvement of localized inflammation in BAVM disease and rupture.
The annual hemorrhage risk is ~ 3%, but, depending on the clinical and anatomic features of the AVM, the risk may be as low as 1% per year (in patients whose initial presentation was nonhemorrhagic) or as high as 33% in hemorrhagic lesions with deep brain or brainstem location and exclusively deep venous drainage. Other features associated with bleeding include feeding artery aneurysm and venous outflow restriction.
Several grading systems have been devised to characterize AVMs and estimate the risks of surgery. The most widely used is the Spetzler-Martin scale. Here, AVMs are graded on a scale from 1-5 based on the sum of “scores” calculated from lesion size, location (eloquent vs. noneloquent brain), and venous drainage pattern (superficial vs. deep).
AVM: CLINICAL ISSUES
Demographics
• Peak age: 20-40 years (mean: 33 years)
• 25% symptomatic by 15 years
Presentation
• Headache with intracranial hemorrhage (ICH) in 50-60%
• Seizure in 25%, neurologic deficit in 25%
Natural History
• Overall annual ICH risk is 3% but wide variation
Treatment Options
Embolization, surgery, stereotactic radiosurgery, or a combination of treatments are all current options in treating ruptured (i.e., hemorrhagic) BAVMs. Stereotactic radiosurgery is an option in patients with small unruptured AVMs (< 3 cm) &/or low Spetzler-Martin grades.
SPETZLER-MARTIN AVM GRADING SCALE
Size
• Small (< 3 cm) = 1
• Medium (3-6 cm) = 2
• Large (≥ 6 cm) = 3
Location
• In noneloquent brain = 0
• In eloquent brain = 1
Venous Drainage
• Superficial veins only = 0
• Deep veins (e.g., internal cerebral veins) = 1
Imaging
The imaging diagnosis of an uncomplicated AVM is relatively straightforward. However, the presence of hemorrhage or thrombosis can complicate its appearance. Acute hemorrhage may obliterate any typical findings of an AVM. Residua of previous hemorrhagic episodes, such as dystrophic calcification, gliosis, and blood in different stages of degradation, may also complicate its appearance.
General Features
AVMs are complex networks of abnormal vascular channels consisting of three distinct components: (1) Feeding arteries, (2) a central nidus, and (3) draining veins (7-1).
CT Findings
AVMs generally resemble a bag of worms formed by a tightly packed tangle of vessels with little or no mass effect on adjacent brain. NECT scans typically show numerous well-delineated, slightly hyperdense serpentine vessels (7-3). Calcification is common. Enhancement of all three AVM components (feeding arteries, nidus, draining veins) is typically intense and uniform on CECT scans.
CTA is commonly included as part of the initial evaluation in patients who present with nontraumatic “spontaneous” intracranial hemorrhage (sICH) and may be helpful in delineating the feeding arteries and draining veins of an underlying AVM (7-4).
MR Findings
The typical appearance of a BAVM is a tightly packed mass or a “honeycomb” of “flow voids” on both T1(7-5A)and T2(7-5B)scans. Any brain parenchyma within an AVM is typically minimal, gliotic, and hyperintense on T2WI and FLAIR (7-5C).
Contrast enhancement of AVMs is variable, depending on flow rate and direction. Draining veins typically enhance strongly and uniformly (7-5D).
Hemorrhagic residua are common. T2* sequences often show foci of “blooming” both within and around AVMs as well as siderosis in the adjacent pia.
Angiography
The pial feeding arteries that supply an AVM are often enlarged and tortuous (7-5E). A flow-induced “pedicle” aneurysm is seen in 10-15% of cases.
The nidus, the core of the AVM, is a tightly packed tangle of abnormal arteries and veins without an intervening capillary bed (7-6). Up to 50% contain at least one aneurysmally dilated vessel (“intranidal aneurysm”).
As there is no intervening capillary bed, direct arteriovenous shunting within the nidus occurs. Draining veins typically opacify in the mid to late arterial phase (“early draining” veins) (7-5F). Veins draining an AVM may become so prominent that they form varices and even exert local mass effect on the adjacent cortex. Stenosis of one or more “outlet” draining veins may elevate intranidal pressure and contribute to AVM hemorrhage.
Approximately 25% of superficially located, large, or diffuse AVMs have some transdural arterial contributions, so thorough evaluation of the dural vasculature should also be part of the complete angiographic delineation of AVM arterial supply.
AVM: IMAGING
NECT
• Slightly hyperdense “bag of worms”
• Tightly packed
• Little/no mass effect
CECT
• Strong serpentine enhancement
MR
• “Honeycomb” of “flow voids”
• No normal brain inside
Differential Diagnosis
• Highly vascular neoplasm (e.g., glioblastoma multiforme)
• Cerebral proliferative angiopathy
Differential Diagnosis
Occasionally, a highly vascular neoplasm, such as glioblastoma (GBM), displays such striking neoangiogenesis that it can mimic an AVM. Most GBMs contain significant amounts of neoplasm interposed between the enlarged vessels. Sometimes, densely calcified neoplasms, such as oligodendroglioma, can mimic the “flow voids” of an AVM on MR.
Cerebral proliferative angiopathy (see below) is a large, diffuse vascular malformation that has innumerable small feeding vessels, no definable nidus, and normal brain interposed between the proliferating vascular channels.
Cerebral Proliferative Angiopathy
Terminology
Cerebral proliferative angiopathy (CPA), formerly considered a variant of AVM known as “holohemispheric giant cerebral AVM” or “diffuse AVM,” is now recognized as a separate entity with distinct imaging features and natural history unlike classic AVMs.
Pathology
CPA is characterized by diffuse abnormal vessels with intermingled brain parenchyma. Feeding arteries are numerous, and draining veins are prominent. In contrast to classic AVMs, there is no nidus and there are no high-flow arteriovenous shunts present. Intralesional hemorrhage is uncommon.
Clinical Issues
While CPA is a progressive disorder, its natural history is quite different from a classic AVM. CPA typically presents with seizure (45%) or headache (40%). Only 15-15% present with neurologic deficits or TIAs.
Mean age at diagnosis is 22 years with a 2:1 female predominance.
Long-term prognosis in CPA is poor. Unlike classic AVMs, CPA is characterized by ongoing angiogenesis and progressive hypervascular shunting. Over time, CPA may demonstrate progressive lesion enlargement with extension into previously uninvolved normal brain parenchyma.
Imaging
CPA is seen on MR as a large (usually > 6 cm) diffusely dispersed network of innumerable dilated vascular spaces intermingled with normal brain parenchyma (7-7). Dense enhancement following contrast administration is typical. Reflecting the hypervascularity, pCT and pMR may demonstrate increased cerebral blood flow (CBF) and cerebral blood volume (CBV), although the brain parenchyma itself is typically hypoperfused. SPECT and PET may demonstrate hypometabolism within the lesion.
DSA and CTA show numerous small feeding arteries, usually with no dominant arterial supply. Recruitment of dural and even transosseous feeders is common. There is no discernible nidus (7-7D), and flow-related aneurysms on feeding vessels are absent. A dense, prolonged vascular “staining” of the affected parenchyma is common. In contrast to AVMs, veins draining CPA are only moderately enlarged relative to the large extent of the abnormality. In further contrast to AVMs, hemorrhage is rare.
Differential Diagnosis
The major differential diagnosis is a large, so-called “diffuse” cerebral AVM. It is crucial to differentiate the two entities because there is lack of dominant feeding arteries and normal functioning brain within a CPA. Unlike AVM, hemorrhage is a rare occurrence in CPA.
Moyamoya disease is an idiopathic progressive occlusive (not proliferative) angiopathy characterized by stenosis of the distal (supraclinoid) internal carotid artery (ICA) with development of an abnormal “telangiectatic” vascular network at the base of the brain.
Dural Arteriovenous Fistula
Dural arteriovenous fistula (dAVF) is the second major type of CVM that exhibits arteriovenous shunting. Much less common than AVMs, dAVFs exhibit a spectrum of biologic behavior that ranges from relatively benign to catastrophic ICH.
A special type of dAVF, carotid-cavernous fistula (CCF), has its own classification schema, distinctive clinical findings, and unique imaging features that differ from dAVFs elsewhere. CCF is discussed separately in this chapter.
Terminology
Intracranial dAVFs are abnormal direct vascular communications between arteries that supply the dura and one or more draining cortical veins or dural venous sinuses. An intervening capillary network is absent.
Etiology
Unlike parenchymal AVMs, adult dAVFs are usually acquired (not congenital). Although the precise etiology is controversial, upregulated angiogenesis in the wall of a thrombosed dural venous sinus with concomitant venous hypertension is the most commonly cited mechanism.
Pathology
Location
Most dAVFs are found in the posterior fossa and skull base. Between 1/3 and 1/2 occur at the transverse/sigmoid sinus junction. Less common sites are the cavernous sinus (CS) and superior petrosal sinus. dAVFs involving the superior sagittal sinus are rare.
Size and Number
dAVFs account for 10-15% of all intracranial vascular malformations with arteriovenous shunting. Most are solitary. Size varies from tiny single vessel shunts to massive complex lesions with multiple feeders and arteriovenous shunts in the sinus wall.
Gross Pathology
Multiple enlarged dural feeders converge in the wall of a thrombosed dural venous sinus (7-8). A network of innumerable microfistulas connects these vessels directly to arterialized draining veins. These crack-like vessels may form a focal mass within the occluded sinus (7-9).
Clinical Issues
Presentation
Most dAVFs occur in adults. The peak age is 40-60 years, roughly 20 years older than the peak age for AVMs.
Clinical presentation varies with location and venous drainage pattern. Uncomplicated dAVFs in the transverse/sigmoid sinus region typically present with either bruit &/or tinnitus. dAVFs in the CS cause pulsatile proptosis, chemosis, retroorbital pain, bruit, and ophthalmoplegia.
“Malignant” dAVFs [lesions with cortical venous drainage (CVD)] may cause focal neurologic deficits, seizures, and progressive dementia.
Natural History
Prognosis depends on location and venous drainage pattern. Most lesions without CVD follow a benign clinical course. Hemorrhage is rare in such cases (~ 1.5% per year). “Malignant” dAVFs often have an aggressive clinical course with high risk for ICH (risk: ~ 7.5% per year).
Treatment Options
Endovascular treatment with embolization of arterial feeders with or without coil embolization of the recipient venous pouch/sinus is most common. Surgical resection of the involved dural sinus wall, used either alone or in combination with endovascular treatment, is another option.
Imaging
CT Findings
CT findings vary from none to striking. Parenchymal hemorrhage is uncommon in the absence of CVD. An enlarged dural sinus or draining vein can sometimes be identified on NECT scans. Dilated transcalvarial channels from enlarged transosseous feeding arteries can occasionally be seen on bone CT images in patients with pulsatile tinnitus (7-10A)(7-10B).
Contrast-enhanced scans may demonstrate enlarged feeding arteries and draining veins. The involved dural venous sinus is often thrombosed or stenotic.
MR Findings
A thrombosed dural venous sinus containing vascular-appearing “flow voids” is the most common finding (7-10C)(7-10D). Thrombus is typically isointense with brain on T1 and T2 scans and “blooms” on T2* sequences. Chronically thrombosed, fibrotic sinuses may enhance.
Parenchymal hyperintensity on T2WI and FLAIR indicates venous congestion or ischemia, usually secondary to retrograde CVD.
Angiography
As most dAVFs arise adjacent to the skull base, multiple enlarged dural and transosseous branches arising from the external carotid artery (ECA) are usually present (7-11A) (7-11B). Dural branches may also arise from the ICA (7-11C)and vertebral arteries (7-11D).
Identifying venous drainage is important. dAVFs with normal antegrade venous drainage or minimal reflux into a dural sinus without drainage into cortical veins are considered low-risk lesions. The presence of dural sinus thrombosis, flow reversal with drainage into cortical (leptomeningeal) veins and tortuous engorged pial veins (pseudophlebitic pattern) (7-13)are common in patients with progressive brain disease (7-12).
Angiographic classification of dAVFs helps stratify risk of dAVF rupture and predict clinical course. The Cognard and Borden classifications are the most commonly used systems (7-14). In either classification, the presence of CVD puts a dAVF into a higher grade category with increased risk of parenchymal hemorrhage. Note that dAVFs are dynamic lesions and may spontaneously regress or progress.
COGNARD/BORDEN CLASSIFICATION OF dAVFs
Benign Venous Drainage Patterns
• Borden
I: Drains to dural sinus; no cortical venous reflux (CVR)
• Cognard
I: Drains to dural sinus without reflux; no CVR
IIa: Drains to dural sinus with reflux; no CVR
Aggressive Venous Drainage Patterns
• Borden
II: Drains to dural sinus; CVR present
III: Direct drainage to cortical veins or to isolated dural sinus segment
• Cognard
IIb: Drains to dural sinus, no sinus reflux; CVR present
III: Direct drainage to cortical veins; no venous ectasia
IV: Direct drainage to cortical veins + venous ectasia
V: Spinal perimedullary venous drainage
Carotid-Cavernous Fistula
Terminology
Carotid-cavernous fistulas (CCFs) are a special type of arteriovenous shunt that develops within the CS (7-15). CCFs are divided into two subgroups, direct and indirect fistulas.
“Direct” CCFs are typically high-flow lesions that result from rupture of the cavernous ICA directly into the CS with or without a preexisting ICA aneurysm. “Indirect” CCFs are usually slow-flow, low-pressure lesions that represent an AVF between dural branches of the cavernous ICA and the CS.
Etiology
CCFs are almost always acquired lesions and can be traumatic or nontraumatic. Most direct CCFs are traumatic, usually secondary to central skull base fractures. A single-hole laceration/transection of the cavernous ICA with direct fistulization into the CS is the typical finding. Spontaneous (i.e., nontraumatic) rupture of a preexisting cavernous ICA aneurysm is a less common etiology.
Indirect CCFs are nontraumatic lesions and are thought to be degenerative in origin. In contrast to dAVFs elsewhere, indirect CCFs rarely occur as sequelae of dural sinus thrombosis. Most indirect CCFs are found in the dural wall of the CS and supplied by intracavernous branches of the ICA and deep (maxillary) branches of the ECA.
Clinical Issues
Epidemiology
An indirect CCF is the second most common intracranial dAVF. Direct high-flow CCFs are much less common.
Demographics
Direct CCFs typically occur with trauma and can occur at any age. Indirect CCFs are most frequent in women 40-60 years of age.
Presentation
Direct CCFs may present within hours to days or even weeks following trauma. Bruit, pulsatile exophthalmos, decreasing vision, and headache are typical. Indirect CCFs cause painless proptosis with variable vision changes.
Treatment Options
Direct CCF fistulae are closed with transarterial-transfistula detachable balloon embolization. Indirect CCFs may be treated conservatively or with superselective embolization.
Imaging
CT Findings
NECT scans may demonstrate mild or striking proptosis, a prominent CS with enlarged superior ophthalmic vein (SOV), and enlarged extraocular muscles. “Dirty” fat secondary to edema and venous engorgement may be present.
CECT scans often nicely demonstrate an enlarged SOV and CS. Inferior draining into a prominent pterygoid venous plexus and posterior drainage into an enlarged clival venous plexus are sometimes present.
CTA shows engorgement of the ophthalmic vein and CS (7-16A). Dehiscence of the intracavernous ICA is characteristic for direct CCF.
MR Findings
T1WIs may show a prominent “bulging” CS and SOV as well as “dirty” orbital fat. High-flow CCFs may show too many CS “flow voids” on T2WIs. Strong, uniform enhancement of the CS and SOV is typical. Enlarged, tortuous intracranial veins may occur with high-flow, high-pressure shunts.
Angiography
DSA is required for definitive diagnosis and treatment. Complete delineation of the arterial supply and venous drainage pattern is the goal.
Direct CCFs typically demonstrate rapid flow with very early CS opacification (7-16B). Selective ICA injection with very rapid image acquisition is often necessary to localize the fistula site exactly. A single-hole fistula is usually present, typically between the C4 and C5 ICA segments.
Indirect CCFs often have multiple dural feeders from cavernous branches of the ICA (meningohypophyseal and inferolateral trunks) as well as deep branches of the ECA (middle meningeal and distal maxillary branches).
Differential Diagnosis
The major differential diagnosis with CCFs is CS thrombosis (CST). Both CCF and CST may cause proptosis, intraorbital edema, enlarged extraocular muscles, and the appearance of “dirty” fat. In CST, the CS may appear enlarged, but prominent filling defects are present on T1 C+ MR.
Pial Arteriovenous Fistula
Intracranial pial arteriovenous fistulas (pAVFs), a.k.a. nongalenic pial arteriovenous fistulas, are rare neurovascular malformations. pAVFs account for < 2% of all intracranial AVMs. They can occur in all ages but are most common in children. pAVFs can be congenital or arise from iatrogenic or traumatic injury.
pAVFs usually consist of a single dilated pial artery that connects directly to an enlarged cortical draining vein within a subpial space. No intervening capillary bed or nidus is present, although a dilated venous pouch (“varix”) is common (7-17).
80% of pAVFs are supratentorial. They typically lie on or just within the brain surface under the pia. Supratentorial pAVFs are supplied by the anterior, middle, or posterior cerebral arteries and are usually associated with a venous varix. “Multihole” pAVFs have multiple feeders.
Vein of Galen Aneurysmal Malformation
Different types of vascular malformations share a dilated vein of Galen as a common feature, but only one of these is a true vein of Galen aneurysmal malformation (VGAM). A VGAM is the most common extracardiac cause of high-output cardiac failure in newborns and comprises 30% of pediatric vascular anomalies.
Terminology
VGAM is essentially a direct AVF between deep choroidal arteries and a persistent embryonic precursor of the vein of Galen, the median prosencephalic vein (MPV) of Markowski (7-18).
Etiology
Normally, the developing internal cerebral veins annex drainage of the fetal choroid plexus as the embryonic MPV—the precursor of the vein of Galen—regresses. In a VGAM, a high-flow fistula prevents formation of the definitive vein of Galen. Mutations in Ephrin signaling genes have been identified in nearly 1/3 of VGAMs.
Clinical Issues
Demographics
VGAMs are rare, representing < 1% of all CVMs. Neonatal VGAMs are more common than those presenting in infancy or childhood. There is a definite male predominance (M:F = 2:1).
Presentation
In neonates, high-output congestive heart failure and a loud cranial bruit are typical. Older infants may present with macrocrania and hydrocephalus.
Natural History
Large VGAMs cause cerebral ischemia and dystrophic changes in the fetal brain. Neonates with untreated VGAMs typically die from progressive brain damage and intractable heart failure. Staged arterial embolization, ideally at 4 or 5 months of age, is the preferred treatment.
Imaging
CT Findings
NECT scans show a well-delineated hyperdense mass at the tentorial apex, usually compressing the third ventricle and causing severe obstructive hydrocephalus. Variable encephalomalacia, hemorrhage, &/or dystrophic calcification in the brain parenchyma are often present. CECT scans show strong uniform enhancement (7-19).
MR Findings
Rapid but turbulent flow in the VGAM causes inhomogeneous signal loss and phase artifact. Enlarged arterial feeders are seen as serpentine “flow voids” adjacent to the lesion.
Angiography
Multiple branches from the pericallosal, choroidal, and thalamoperforating arteries drain directly into an enlarged, aneurysmally dilated midline venous sac (7-20). In > 50% of all VGAMs, the straight sinus is hypoplastic or absent, and venous drainage is into a persistent embryonic “falcine sinus” (7-19).
Differential Diagnosis
Typical imaging findings in a neonate with high-output congestive heart failure are virtually pathognomonic of VGAM. A thalamic AVM with deep venous drainage may cause secondary enlargement of the vein of Galen but rarely presents in the neonatal period. A high-flow giant childhood dural AVM (dAVM)may present in infancy and clinically resemble a VGAM. Involvement of the dural venous sinuses rather than the vein of Galen is typical.
Cerebrovascular Malformations Without Arteriovenous Shunting
Developmental Venous Anomaly
Terminology
Developmental venous anomaly (DVA), a.k.a. venous “angioma”or “venous malformation,” is an umbrella-shaped congenital cerebral vascular malformation composed of angiogenically mature venous elements. Dilated, thin-walled venous channels lie within (and are separated by) normal brain parenchyma.
Etiology
The precise etiology of DVAs is unknown. Most investigators believe it is arrested medullary vein development between 8 and 11 gestational weeks that results in DVAs. The underlying genetics involved in DVA development, whether germ-line or somatic, remain to be elucidated.
Pathology
Location
Approximately 70% of DVAs are found in the deep white matter, adjacent to the frontal horn of the lateral ventricle (7-21). The second most common location is next to the fourth ventricle (15-30%).
DVA depth is defined as where the medullary venous radicles converge into the collector vein. Three depths are recognized: Juxtacortical, subcortical, and periventricular (7-22). Some larger DVAs can have dual or even triple convergence sites.
Size and Number
DVA size varies from tiny, almost imperceptible lesions to giant venous malformations that involve most of the hemispheric white matter.
Between 6-7% of patients with a DVA have two lesions; multiple DVAs occur in 1%. Multiple DVAs have been reported in blue rubber bleb nevus syndrome (BRBNS, a.k.a. Bean syndrome) and other superficial craniofacial venous and venolymphatic malformations.
Gross Pathology
DVAs consist of two elements: A cluster of variably sized prominent medullary (white matter) veins (the so-called caput medusa) that converges on an enlarged stem or single “collector vein.” A DVA is embedded within and drains grossly normal-appearing brain parenchyma, forming its primary or sole venous drainage pathway (7-23).
Hemorrhage and calcification are uncommon unless the DVA is associated with another vascular malformation or the collecting vein becomes thrombosed. The most common “histologically mixed” CVM is a cavernous-venous malformation found in 10-15% of patients with a DVA.
Microscopic Features
Thin-walled, somewhat dilated venous channels are interspersed in normal-appearing white matter. Varying degrees of focal parenchymal atrophy, white matter gliosis, neuronal degeneration, and demyelination may be present within the venous drainage territory of some DVAs.
Clinical Issues
Demographics
DVA is the most common of all intracranial vascular malformations, accounting for 60% of all CVMs. Estimated prevalence on contrast-enhanced MR scans ranges from 2.5-9.0%.
Presentation
DVAs occur in patients of all ages. At least 98% of isolated DVAs are asymptomatic and discovered incidentally. About 2% initially present with hemorrhage or infarct, often related to presence of a coexisting cavernous malformation or stenosis of the collecting (“outlet”) vein, usually at the entrance to the superior sagittal sinus.
DVAs may also coexist with a sinus pericranii (SP). SP is typically the cutaneous sign of an underlying venous anomaly (7-31). DVAs are also associated with HHT (4% of cases). Other reported associations include malformations of cortical development.
Because multiple sclerosis (MS) lesions evolve predominantly around venous structures, some studies indicate MS may be associated with an increased prevalence of DVAs. Occasionally, demyelinating disease will occur in conjunction with a DVA. MS-associated DVAs are not associated with significantly altered clinical outcomes or disease progression.
DEVELOPMENTAL VENOUS ANOMALY
Terminology
• DVA is a.k.a. venous “angioma”
Pathology
• Solitary > > multiple; small > large
• Enlarged white matter veins interspersed with normal brain
• Usually found adjacent to lateral or fourth ventricle
Clinical Issues
• Epidemiology and demographics
Most common CVM (60%)
Prevalence on T1 C+ MR: 2-9%
All ages, no sex predilection
Natural History
• Usually benign, nonprogressive
• May hemorrhage if mixed with cavernous malformation or collector vein thrombosis
Treatment Options
No treatment is required or recommended for solitary DVAs (they are “leave me alone” lesions). If a DVA is histologically mixed, treatment is determined by the coexisting lesion. Preoperative identification of such mixed malformations is important, as ligating the collector vein or removing its tributaries may result in venous infarction.
Imaging
General Features
DVAs are composed of radially arranged medullary veins that converge on a transcortical or subependymal large collector vein. The classic appearance is that of a Medusa head or upside-down umbrella (7-21).
CT Findings
NECT scans are usually normal unless the collector vein is large (7-27). Unilateral basal ganglia calcification has been reported in the drainage territory of some deep DVAs (7-24A).
CECT scans and CTVs show numerous linear &/or punctate enhancing foci that converge on a well-delineated tubular collector vein (7-24B) (7-24C). In larger DVAs, perfusion CT may show a venous congestion pattern with increased CBV, CBF, and mean transit time (MTT) in the adjacent brain parenchyma.
MR Findings
If the DVA is small, it may be undetectable unless contrast-enhanced scans or susceptibility-weighted sequences are obtained.
T2/FLAIR
Large DVAs can exhibit a “flow void” on T2WI (7-25A). Venous radicles of the caput medusa are often hyperintense on T2/FLAIR. DVA–associated parenchymal abnormalities are common. T2/FLAIR parenchymal abnormalities are present in 10-25% of DVAs (7-28A). The etiology of such associated hyperintensities is unknown but could represent gliosis or hemodynamic stress, such as venous congestion or ischemia, demyelination, or hypomyelination.
T2* (GRE, SWI)
Because flow in the venous radicles of a DVA is typically slow, blood deoxygenates and T2* scans (GRE, SWI) show striking linear hypointensities (7-23).
If a DVA is mixed with a cavernous malformation, blood products in various stages of degradation may be present and “bloom” on T2* sequences (7-30). Discrete hypointense foci on 3T SWI are seen in the majority of cases, especially in DVAs that are associated with parenchymal hyperintensities on FLAIR. Venous congestion in a DVA may result in microhemorrhages or possibly promote the formation of small cavernous malformations.
T1 C+
T1 C+ sequences show a stellate collection of linear enhancing structures (7-26B)converging on the transparenchymal or subependymal collector vein (7-25B) (7-25C) (7-28B). The collector vein may show variable high-velocity signal loss (7-26A).
pMR and fMRI
DSC pMR is usually normal in small DVAs. Elevated CBV and CBF with mildly increased MTT are common within larger DVAs. DVAs can strongly resemble neural signal on resting-state fMRI.
Angiography
Except in the rare “arterialized” DVA, the arterial phase is normal. The venous phase shows the typical hair-like collection (“Medusa head”) of dilated medullary veins within the white matter. A faint, prolonged “blush” or capillary “stain” may be present in some cases.
Nuclear Medicine Studies
More than 3/4 of DVAs are associated with hypometabolism in the adjacent brain parenchyma on FDG PET studies, often in the absence of any other structural abnormalities.
Differential Diagnosis
A histologically mixed vascular malformation in which the DVA provides prominent venous drainage is common. The most common combination is a mixed cavernous-venous malformation (7-30). Unusually large (“giant”) capillary telangiectasias often have a dominant central collector vein and may therefore resemble a DVA (7-46). DVAs have also been reported adjacent to foci of cortical dysplasia and in/adjacent to demyelinating lesions (see Digital-Only images 27-30).
DEVELOPMENTAL VENOUS ANOMALY: IMAGING
MR
• T2/FLAIR
Collector vein = flow void on T2WI
Radicles of “Medusa head” hyperintense on FLAIR
10-25% have T2/FLAIR parenchymal hyperintensities
• T2* (GRE/SWI)
Linear hypointensities in radicles, collector vein
“Blooming” foci if mixed with cerebral cavernous malformations
3T SWI may show microbleeds
• T1 C+
“Umbrella” of tubular radicles → collector vein
• pMR
Increased CBV/CBF, increased MTT
DSA
• Classic “Medusa head” in venous phase
Sinus Pericranii
Terminology
Sinus pericranii (SP) is a rare benign venous anomaly that consists of an emissary intradiploic vein that connects an intracranial dural venous sinus with an extracranial venous varix (7-31). The dilated venous pouch hugs the external table of the skull.
Pathology
A bluish sac beneath or just above the periosteum of the calvarium is typical. The dilated, blood-filled sac connects with the intracranial circulation through a well-defined bone defect (7-31). The frontal lobe is the most common site, followed by the parietal and occipital lobes. SPs in the middle and posterior cranial fossae are rare.
SP may be associated with single or multiple intracranial DVAs. Other reported associations include craniosynostosis and dural sinus hypoplasia. SP with multiple DVAs is associated with BRBNS.
Imaging
CT Findings
An SP shows strong uniform enhancement after contrast administration (7-32). The underlying calvarial defect varies in size but is typically well demarcated.
MR Findings
Most SPs are isointense on T1WI and hyperintense to brain on T2WI. “Puddling” of contrast within the SP on T1 C+ is typical unless the lesion is unusually large and flow is rapid. MRV is helpful in delineating both the intra- and extracranial components.
Cerebral Cavernous Malformation
Cerebral cavernous malformations (CCMs) are a distinct type of intracranial vascular malformation characterized by repeated “intralesional” hemorrhages into thin-walled, angiogenically immature, blood-filled locules called caverns. CCMs are discrete, well-marginated lesions that do not contain normal brain parenchyma. Most are surrounded by a complete hemosiderin rim (7-33).
Cavernous malformations exhibit a wide range of dynamic behaviors. They are a relatively common cause of spontaneous nontraumatic ICH in young and middle-aged adults, although they can occur at any age.
Terminology
CCMs are a.k.a. cavernous “angiomas” or “cavernomas.” They are benign malformative vascular hamartomas. CCMs are sometimes erroneously referred to as cavernous hemangiomas. Hemangiomas are benign vascular neoplasms, not malformations.
Etiology
General Concepts
CCMs are angiogenically immature lesions with endothelial proliferation and increased neoangiogenesis. They consist of closely clustered, abnormally dilated and leaky capillaries that occur predominately in the central nervous system.
CCMs can be inherited or acquired. Acquired CCMs are rare and usually associated with prior radiation therapy (XRT). Approximately 3.5% of children who have whole-brain XRT develop multiple CCMs with a mean latency interval of ~ 3 years (3-102 months).
Genetics
CCMs can be solitary and sporadic (80% of cases) or multiple and familial (20%). Familial CCMs are inherited as an autosomal dominant disease with variable penetrance and are more common in Hispanic Americans of Mexican descent.
Three independent genes have been identified in familial CCMs: KRIT1/CCM1, CCM2, and PDCD10/CCM3. All the mutations in these genes cause a loss of function and compromise the protein functions needed for maintaining vascular barrier integrity.
Associated Abnormalities
CCMs are the most common component in mixed vascular malformations. Cavernous-venous and cavernous-capillary are the two most frequent combinations.
Pathology
Location and Size
CCMs can occur anywhere in the CNS and range in size from tiny, near-microscopic lesions to giant malformations that can occupy an entire lobe or most of the cerebral hemisphere.
Gross Pathology
A compact, spongy collection of reddish-purple blood-filled “caverns” devoid of intervening neural elements is typical. Most CCMs are surrounded by a rust-colored rim of gliotic, indurated brain.
Staging, Grading, and Classification
The most commonly used classification of CCMs, the Zabramski classification, is based on imaging appearance, not histologic findings (see next shaded box).
Clinical Issues
Epidemiology
CCMs are the third most common CVM (after DVA and capillary telangiectasia) and are found in ~ 0.5% of the population. 2/3 occur as a solitary, sporadic lesion; ~ 1/3 are multiple.
CEREBRAL CAVERNOUS MALFORMATIONS
Etiology
• KRIT1, CCM2, or PDCD10 mutations in familial CCM
Pathology
• Occur throughout CNS
• Solitary (2/3), multiple (1/3, familial)
• Consist of closely clustered, abnormally dilated leaky capillaries
• Multiple thin-walled blood-filled locules (“caverns”)
• No normal brain inside; hemosiderin rim outside
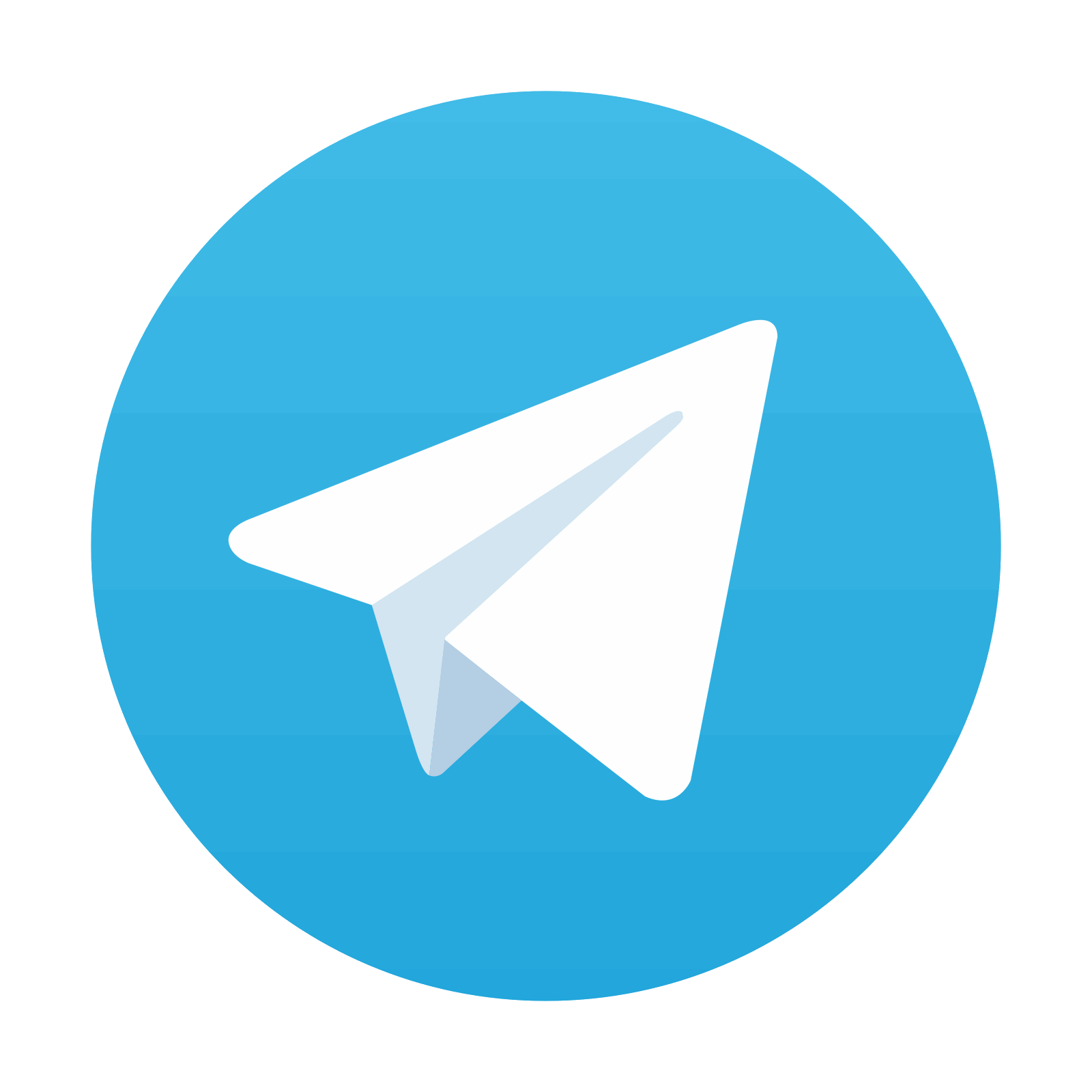
Stay updated, free articles. Join our Telegram channel

Full access? Get Clinical Tree
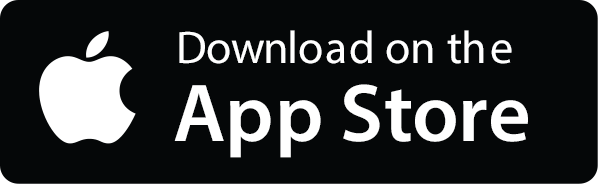
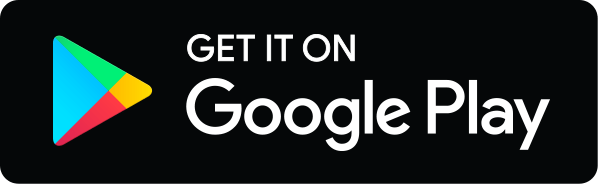