Fig. 24.1
Recruitment of EPCs to injured spinal cord. (a–d) Bone marrow (BM)-derived EPCs were serially identified in the spinal cord of wild-type mice with SCI following BM transplantation from Tie2/lacZ transgenic mice. Representative immunohistochemistry for β-gal (red) at days 3 (a), 7 (b), 14 (c), and 28 (d) after injury. Nuclei were counterstained with DAPI (blue). (e–h) Immunohistochemical staining for CD31 (green) and β-gal (red) at days 3 (e), 7 (f), 14 (g), and 28 (h) after injury reveals that β-gal positive cells are also labeled with CD31 (e). The β-gal positive cells are scattered at day 3, but are distributed along CD31 positive vessels at day 7 or later. Scale bars: left panel of (a–d), 300 μm; right panel of (a–d), 50 μm, and (e–h), 50 μm
24.2.3 Administration of Exogenous EPCs for Repairing Injured Spinal Cord
To clarify the contribution of EPC recruitment to histological changes in the injured spinal cord, cultured EPCs labeled with DiI-ac-LDL or phosphate buffered saline (PBS) were administered systemically just after SCI. DiI positive cells indicating administered EPCs were observed around the injury site at day 3. Immature reactive astrocytes co-labeled with GFAP and nestin were increased by the administration of EPCs at day 3 after injury. CD31+ blood vessels were also increased by the administration of EPCs at day 3 after injury. These findings suggest that systemically administered EPCs are recruited into the injured spinal cord and enhance neovascularization and astrogliosis following SCI.
24.3 EPCs Enhance Astrogliosis Through Notch Signaling
Although the exact molecular mechanisms underlying the interaction between NSCs and vascular cells still remain elusive, the previous study showed that endothelial cells stimulated the self-renewal of NSCs and enhanced NSC-derived neurogenesis through Notch signaling [11]. We therefore hypothesized that a Notch signaling mediated interaction between NSCs and EPCs might promote the activation of endogenous NSCs and subsequently lead to the enhanced repair of the injured spinal cord. In this study, we used conditional Jagged1 (Jag1) knockout mice (loxP/loxP, Mx-Cre). EPCs were cultured from bone marrow mononuclear cells isolated from Jag1+/+ as well as Jag1−/− mice. EPCs (1 × 106 cells in 200 μL of PBS) derived from Jag1+/+ mice or Jag1−/− mice were injected to mouse models of SCI intravenously immediately after injury (Jag1+/+ EPC group, Jag1−/− EPC group), as well as 200 μL of PBS (PBS group) [12].
24.3.1 Notch Ligands in Cultured EPCs
The levels of expression of Notch ligands, including Jag1 and Delta-like 1 (Dll1), in cultured EPCs were confirmed by quantitative real-time PCR. Jag1−/− EPCs lack expression of Jag1, whereas no significant difference in the expression of Dll1 could be detected between Jag1−/− and corresponding wild-type EPCs.
24.3.2 Functional Recovery After Spinal Cord Injury
The recovery of hindlimb function was assessed using the basso mouse scale (BMS) [13]. The BMS score in the Jag1+/+ EPC group was significantly higher than in the PBS and the Jag1−/− EPC group at days 14 or later. Although the average score in the Jag1−/− EPC group was slightly higher than in the PBS group at days 14 or later after SCI, there was no significant difference in the BMS score between these two groups. To assess functionality and recovery of descending pathways from the forebrain to the hindlimb motor neuron pool, motor evoked potentials (MEPs) were monitored in the hamstring muscles. At 6 weeks after SCI, the amplitudes of MEPs in the PBS group, Jag1+/+ EPC group, and Jag1−/− EPC group were 13.33 ± 5.16 %, 24.31 ± 7.31 %, and 13.73 ± 5.27 % of the pre-injury values, respectively. The amplitude in the Jag1+/+ EPC group was significantly higher than in the PBS and the Jag1−/− EPC group. However, there was no significant difference in the amplitudes between the PBS and the Jag1−/− EPC group. These findings suggest that the transplantation of Jag1+/+ EPCs but not Jag1−/− EPCs promoted the functional recovery after SCI.
24.3.3 Incorporation of Transplanted EPCs
To assess the incorporation of administered EPCs into injured spinal cord, EPCs were labeled with DiI-acetyl LDL prior to administration. DiI+ cells could be observed around the injury site in both Jag1+/+ EPC and Jag1−/− EPC groups; however, no DiI+ cells were detected in the PBS group. No significant difference in the number of incorporated DiI+ cells was detected between these two groups.
24.3.4 Astrogliosis Following Spinal Cord Injury
At day 3 after SCI, reactive astrocytes were stained with antibodies against GFAP and nestin. A large number of reactive astrocytes were observed in the Jag1+/+ EPC group, whereas only few could be detected in the PBS and Jag1−/− EPC group (Fig. 24.2a, b). The mRNA expressions of the Notch effectors Hes1 and Hes5 in the injured spinal cord were assessed by real-time quantitative PCR. Although the expression level of Hes1 in the Jag1+/+ EPC group was slightly higher than in the PBS and Jag1−/− EPC group, no significant difference in Hes1 expression could be detected. In contrast, the expression level of Hes5 in the Jag1+/+ EPC group was significantly higher than in the PBS and the Jag1−/− EPC group, with again no significant difference between the PBS and Jag1−/− EPC group (Fig. 24.2c). At day14 after injury, spinal cord tissues were stained with GFAP for the assessment of astrogliosis. The GFAP negative area in the Jag1+/+ EPC group was significantly smaller than in the PBS and Jag1−/− EPC group, the latter showing again no significant difference.
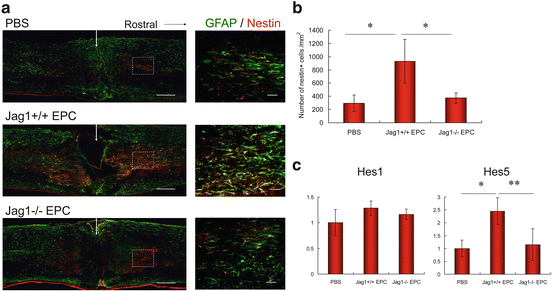
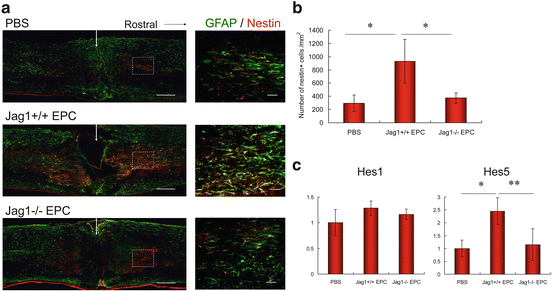
Fig. 24.2
Assessment of astrogliosis. (a) Spinal cord tissues at day 3 after injury immunostained with GFAP (green) and nestin (red). Arrow indicates epicenter of injury site. (b) Number of nestin+ cells at day 3 after injury (n = 6). *P < 0.0005. (c) mRNA expressions of Notch effectors Hes1 and Hes5 in spinal cord tissues at day 3 after injury measured by real-time quantitative PCR (n = 6). Scale bar: (a) large pictures, 300 μm, and small pictures, 50 μm
24.3.5 Neovascularization in the Injured Spinal Cord
Spinal cord sections were immunostained with CD31 at days 3 and 14 after injury in order to assess the degree of neovascularization. At day 3 after SCI, although very little or no angiogenesis was observed at the epicenter zone in all groups, CD31+ vessels were observed in the border zone. In this area, the mean diameter of vessels in the Jag1+/+ EPC group was significantly greater than in the PBS and Jag1−/− EPC group. The number of vessels in the Jag1+/+ and Jag1−/− EPC groups was significantly greater than in the PBS group, whereas no significant difference could be observed between both EPC groups. At day14 after injury, a chaotic vascular architecture with large vessels adjacent to small vessels was observed in the PBS and Jag1−/− EPC group, though no morphological abnormalities of the vessels could be observed for the Jag1+/+ EPC group. These results suggest that both Jag1+/+ EPCs and Jag1−/− EPCs enhanced angiogenesis at day 3 after SCI and that Jag1+/+ EPCs but not Jag1−/− EPCs normalized vascular morphology at day 14 after SCI.
24.3.6 Assessment of Axons
To analyze defined subsets of descending axons, immunohistochemistry for TH (a marker for descending noradrenergic and dopaminergic axons as well as sympathetic fibers) and 5HT (a marker for descending serotonergic axons) was performed at day 42 after SCI [14]. To evaluate the axon growth beyond the injury site, quantitative assessment of axons was performed by measuring the area of immunostained axons in the far rostral region (5 mm rostral to the epicenter), the injury site (the caudal region adjacent to the epicenter), and the far caudal region (5 mm caudal to the epicenter). At the injury site, the area of TH+ and 5HT+ axons in the Jag1+/+ EPC group was significantly larger than in the PBS and Jag1−/− group. In addition, the area of 5HT+ axons in the Jag1−/− EPC group was significantly larger than in the PBS group. In the far caudal region, TH+ and 5HT+ axons were clearly observed in the Jag1+/+ EPC group, whereas these axons were rare in the PBS and Jag1−/− EPC group. The area of TH+ or 5HT+ axons in the Jag1+/+ EPC group was significantly larger than in the PBS and Jag1−/− group.
24.3.7 Transplantation of Notch Ligand-Overexpressing 3T3 Stromal Cells
To clarify the role of ligand-specific Notch activation for the enhancement of astrogliosis following SCI, 3T3 stromal cells transfected with Jag1, Dll1, or empty vector were transplanted directly into the injury site of spinal cords immediately after SCI (Jag1-3T3 group, Dll1-3T3 group, and empty-3T3 group). At day 3 after injury, spinal cord sections were immunostained with GFAP and nestin. In the assessment of reactive astrocytes stained with GFAP and nestin, a large number of reactive astrocytes were observed in the Jag1-3T3 group, though these cells were rare in the empty-3T3 and Dll1-3T3 groups. The number of nestin+ cells in the Jag1-3T3 group was significantly greater than in the empty-3T3 and Dll1-3T3 groups. These findings suggest that Jag1/Notch signaling but not Dll1/Notch signaling enhanced astrogliosis after SCI.
24.4 Transplantation of Human CD133+ Cells
The population of CD133+ cells in human blood, known to be a subpopulation of CD34+ cells, is well characterized as a hematopoietic/endothelial progenitor fraction. CD133+ cells in human blood appear to be suited for clinical application as isolation of these cells is relatively safe and raises only limited ethical concerns. However, the acquisition of a sufficient number of cells remains the most important factor limiting clinical application, as the proportion of CD133+ cells in respect to the overall number of MNCs in human blood is very small [15]. We developed a serum-free culture system for human cord blood-derived CD133+ cells enhancing the cellular function as well as the number of EPCs destined to be used for the revascularization of ischemic organs and tissues [16]. We analyzed and compared ex vivo expanded human cord blood-derived CD133+ cells with freshly isolated CD133+ cells as well as corresponding CD133− control MNCs in respect to their ability to promote spinal cord repair using in vitro assays and cell transplantation into a mouse SCI model [17].
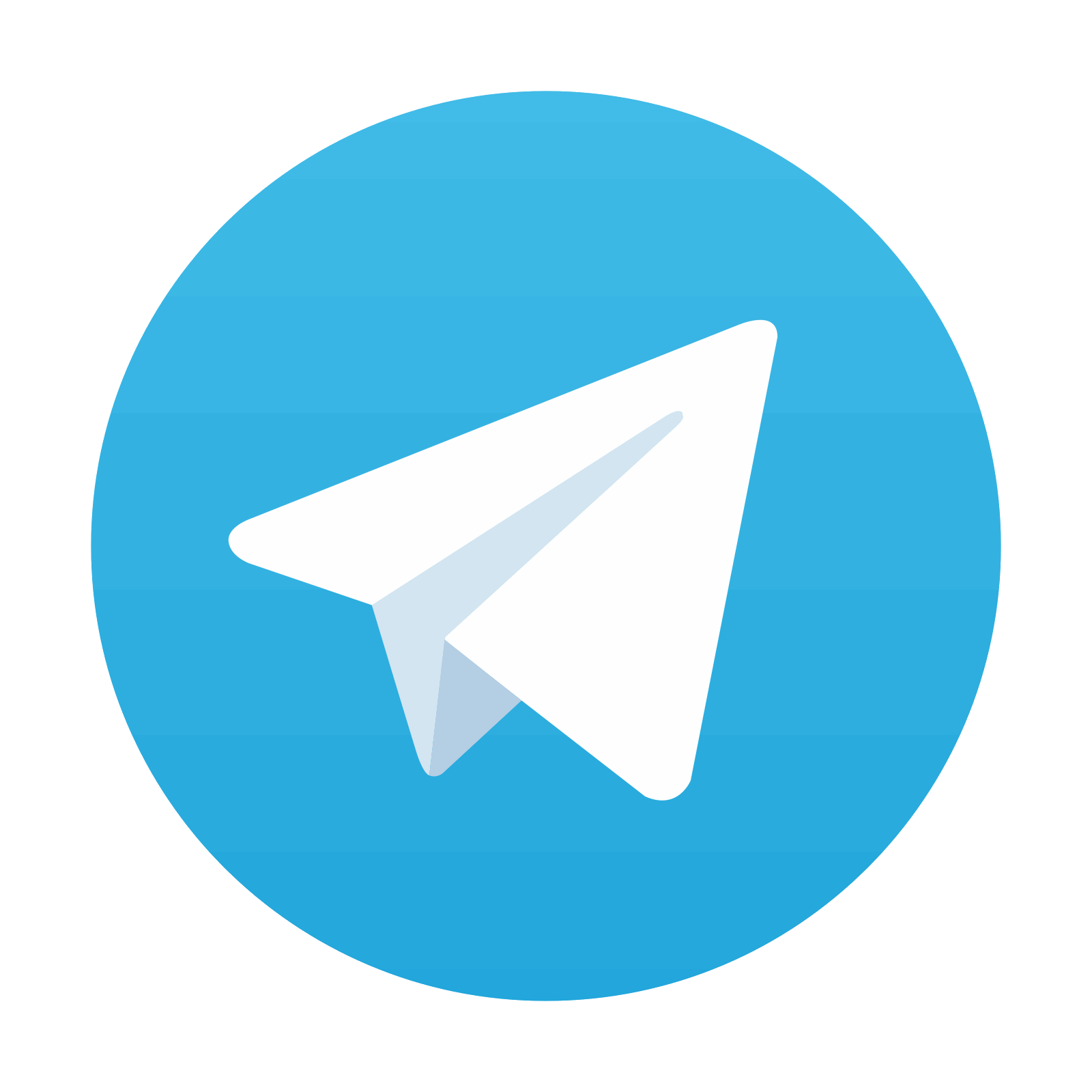
Stay updated, free articles. Join our Telegram channel

Full access? Get Clinical Tree
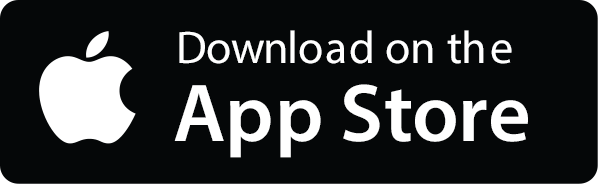
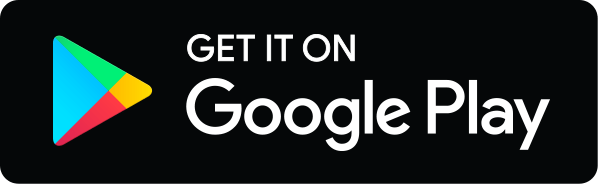