CLINICAL CASE | Middle Cerebral Artery Occlusion, Right Side Paralysis, and Global Aphasia
A 57-year-old male was brought to the emergency room after being discovered by his wife to be unable to move his right arm or leg. On testing, his right upper limb strength was 0/5 and the lower limb, 1/5. The left limbs had normal strength and spontaneous movements. In addition, there was drooping of the right side of the lower face. Pinch of the nail beds—a mildly noxious stimulus that elicits a withdrawal—revealed withdrawal of the left arm but no response for the right arm. The patient was able to look to the left but not the right; there were no saccadic (rapid, conjugate) eye movements to the right. The patient was unable to speak and only followed simple commands.
Figure 3–1A shows a horizontal MRI. The large white territory corresponds to the infarcted region on the left side of the cerebral hemisphere. Figure 3–18B is a magnetic resonance angiogram (MRA), showing the distribution of arteries with flowing blood. Note the asymmetry in the MRA, with an absence of middle cerebral artery perfusion on the left side.
Answer the following questions based on your readings of the case report and this chapter.
1. The patient’s lesion is large. Occlusion of which cerebral artery produced the lesion, and what was the contribution of its deep and superficial branches?
2. Damage to what single key structure could produce the major limb and facial motor signs?
Key neurological signs and corresponding damaged brain structures Paralyzed right arm and legThe corticospinal tract, which is key to controlling the contralateral arm and leg, descends subcortically and then travels in the posterior limb of the internal capsule (see Figure 2–17). This subcortical white matter and the more dorsal parts of the posterior limb of the internal capsule are supplied by deep branches of the middle cerebral artery. The infarction also would have destroyed part of the lateral precentral gyrus, where the corticospinal tract to the arm segments of the spinal cord originates. This is supplied by superficial branches of the middle cerebral artery. By contrast, the infarction spares the leg area of motor cortex (see Figure 10–8). Whereas the descending axons are destroyed when they are in the internal capsule, as we shall see in later chapters sparing of the cortex may help during neurorehabilitation.
Right lower facial droopThe corticobulbar tract controls facial muscles. This is the component of the descending cortical motor pathway that controls cranial motor nuclei in the brain stem. (Bulb is an archaic term to describe the lower brain stem.) The tract travels subcortically from lateral part of the precentral gyrus (face area of motor cortex) to the genu and posterior limb of the internal capsule, rostral to the corticospinal tract axons. The subcortical white matter and dorsal parts of the internal capsule are largely supplied by the deep branches of the middle cerebral artery. The face-controlling area of motor cortex is supplied by superficial branches of the middle cerebral artery. Interestingly, control of the lower face—like that of the arms and legs—is strictly contralateral, but upper facial muscle control is bilateral. Damage to the corticobulbar tract on one side thus eliminates control of lower face on the opposite side, resulting in paralysis or major weakness. Upper facial muscles, because they receive control by both sides of the brain, are functional after a unilateral corticobulbar tract lesion.
Absence of limb withdrawal to noxious stimulationDamage to the internal capsule can destroy the ascending thalamocortical projection, carrying somatic sensory information to the postcentral gyrus. This results in the loss of somatic sensation. However, in the case of our patient, there were no spontaneous right arm movements and there was no upper limb strength. Therefore, it is unlikely that he would have been able to move the limb had noxious stimulation been felt.
Absence of eye movement to the rightThe infarction damaged the cortical regions, and their descending control pathways, for saccadic eye movements. These are the rapid, darting, eye movements we use to shift our gaze from one object of interest to another.
Inability to speak and understand languageThe cortical centers controlling speech are in the left hemisphere in most right-handed individuals. These areas are Wernicke’s area in the superior temporal gyrus, for sensory processing in speech, and Broca’s area in the inferior frontal lobule, for producing speech. Both areas are supplied by superficial branches of the middle cerebral artery. Their axonal interconnections are also supplied, in large part, by the middle cerebral artery. In the absence of these structures, there is loss of language, both spoken and understanding.
FIGURE 3–1
Neuroradiological imaging after stroke. A. Diffusion-weighted image (DWI) showing a large left middle cerebral artery infarction. The white region corresponds to the infarcted territory of the middle cerebral artery. B. Magnetic resonance angiogram (MRA) showing a complete lack of perfusion of the left middle cerebral artery. This demonstrates occlusion at its proximal portion. (Reproduced with permission from Ropper AH et al. Adams & Victor’s Principles of Neurology, 9th ed. AccessMedicine; 2009, Fig. 34–3.)

Brain vasculature disorders constitute a major class of nervous system disease. The principal source of nourishment for the central nervous system is glucose, and because neither glucose nor oxygen is stored in appreciable amounts, when the blood supply of the central nervous system is interrupted, even briefly, brain functions become severely disrupted.
Much of what is known about the arterial supply to the central nervous system derives from three approaches. First, classical studies in normal postmortem tissue used colored dye injected into a blood vessel to identify the areas it supplies. Second, in postmortem tissue or on radiological examination, the portion of the central nervous system supplied by a particular artery can be inferred by observing the extent of damage that occurred after the artery became occluded. Third, radiological techniques, such as cerebral angiography and magnetic resonance angiography, make it possible to view the arterial and venous circulation in the living brain (see Box 3–1). These important clinical tools also permit localization of a vascular obstruction or other pathology.
Box 3–1 Radiological Imaging of Cerebral Vasculature
Cerebral vessels can be observed in vivo using cerebral angiography. First, radiopaque material is injected into either the anterior or the posterior arterial system. Then a series of skull x-ray images are taken in rapid repetition as the material circulates. Images obtained while the radiopaque material is within cerebral arteries are called angiograms or arteriograms. Images can also be obtained later, after the radiopaque substance has reached the cerebral veins or the dural sinuses (venograms). The entire course of the internal carotid artery is shown in cerebral angiograms in Figure 3–9. Images can be obtained from different angles with respect to the cranium. Two views are common—from the front (frontal projection, Figure 3–9A) and from the side (lateral projection, Figure 3–9B). The lateral view shows the C-shape of the anterior cerebral artery (and its branches). The medial-to-lateral course of the middle cerebral artery is revealed in the frontal view.
The rostrocaudal course of the middle cerebral artery, from the point at which it enters the lateral sulcus to the point at which it emerges and distributes over the lateral surface of the cerebral cortex, is revealed in the lateral view (Figure 3–9B). The middle cerebral artery forms loops at the dorsal junction of the insular cortex and the opercular surface of the frontal and parietal lobes (see Figure 3–8). These loops serve as radiological landmarks that aid in estimating the position of the brain in relation to the skull. Figure 3–10 shows the posterior circulation viewed from a lateral perspective. Figure 3–11 shows the two vertebral arteries joining to form the basilar artery and the subsequent bifurcation of the basilar artery into the two posterior cerebral arteries.
Cerebral angiography involves intravascular injection of radiopaque material. The process of injecting this material, and the material itself, can produce neurological complications; therefore, its use is not without risk. Magnetic resonance imaging has also been applied to the study of brain vasculature because it can detect motion of water molecules. This application, termed magnetic resonance angiography (MRA), selectively images blood in motion. The MRA scan in Figure 3–12 is a dorsoventral reconstruction (ie, as if looking up from the bottom). The posterior communicating artery is present only on the left side. This patient does not have a complete circle of Willis. The entire cerebral circulation can be reconstructed from the locations of cerebral arteries or veins at multiple levels.
As discussed in previous chapters, brain vasculature is closely related to the ventricular system and the watery fluid contained within it, the cerebrospinal fluid. This is because most cerebrospinal fluid is produced by active secretion of ions from blood plasma by the choroid plexus. Moreover, to maintain a constant brain volume, cerebrospinal fluid is returned to the blood through valves between the subarachnoid space and the dural sinuses.
This chapter initially focuses on arterial supply because of the importance of distributing oxygenated blood to the brain and spinal cord for normal function, followed by venous drainage. The blood-brain barrier, which isolates the intravascular compartment from the extracellular compartment of the central nervous system, is considered next. Finally, cerebrospinal fluid production and circulation within the different components of the ventricular system is examined.
Local regions of the central nervous system receive blood from small sets of penetrating arteries that receive their blood from the major arteries (Table 3–1). Cessation or reduction of the arterial supply to an area results in decreased delivery of oxygenated blood to the tissue, a condition termed ischemia. Decreased blood supply typically occurs when an artery becomes occluded or when systemic blood pressure drops substantially, such as during a heart attack. Occlusion commonly occurs because of an acute blockade, such as from an embolus, or the gradual narrowing of the arterial lumen (stenosis), as in atherosclerosis.
Structure | Level | System | Major artery1 |
---|---|---|---|
Spinal Cord | P | Anterior spinal artery | |
P | Posterior spinal artery | ||
S | Radicular arteries | ||
Medulla | Caudal | P | Anterior spinal artery |
P | Posterior spinal artery | ||
Rostral | P | Vertebral | |
P | Vertebral: PICA | ||
Pons | Caudal and middle | P | Basilar |
P | Basilar: AICA | ||
Rostral | P | Basilar: SCA | |
Cerebellum | Caudal | P | Vertebral: PICA |
Middle | P | Basilar: AICA | |
Rostral | P | Basilar: SCA | |
Midbrain | Caudal | P | Basilar |
(inferior colliculi) | P | Basilar: SCA | |
Rostral | P | Posterior cerebral | |
(superior colliculi) | |||
Diencephalon | |||
Thalamus | A | Posterior communicating | |
P | Posterior cerebral: posterior choroidal | ||
P | Posterior cerebral: thalamogeniculate | ||
P | Posterior cerebral: thalamoperforating | ||
Hypothalamus | A | Anterior cerebral | |
A | Anterior communicating | ||
A | Posterior communicating | ||
P | Posterior cerebral | ||
Subthalamus | A | Anterior choroidal | |
A | Posterior communicating | ||
P | Posterior choroidal | ||
P | Posterior cerebral | ||
Basal Ganglia | |||
Globus pallidus | Superior | A | Middle cerebral: lenticulostriate |
Middle, inferior | A | Anterior choroidal | |
Striatum | Superior | A | Middle cerebral: lenticulostriate |
Inferior | A | Anterior cerebral: lenticulostriate | |
Septal Nuclei | A | Anterior cerebral | |
A | Anterior communicating | ||
A | Anterior choroidal | ||
Amygdala | A | Anterior choroidal | |
Hippocampal | A | Posterior cerebral | |
Formation | |||
Internal Capsule | |||
Anterior limb | Superior | A | Middle cerebral |
Middle | A | Anterior cerebral | |
Inferior | A | Internal capsule | |
Inferior | A | Anterior choroidal | |
Genu | Superior | A | Middle cerebral |
Middle | A | Anterior cerebral | |
Inferior | A | Anterior choroidal; anterior cerebral | |
Posterior limb | Superior | A | Middle cerebral |
Inferior | A | Anterior choroidal | |
Retrolenticular | A | Anterior choroidal | |
Cerebral Cortex | |||
Frontal lobe | A | Anterior cerebral | |
A | Middle cerebral | ||
Parietal lobe | A | Anterior cerebral | |
A | Middle cerebral | ||
Occipital lobe | P | Posterior cerebral | |
Temporal lobe | P | Posterior cerebral |
A brief reduction in blood flow produces transient neurological signs, attributable to lost functions of the oxygen-deprived area. This event is termed a transient ischemic attack (TIA). If ischemia is persistent and is uncorrected for several minutes, it can cause death of the tissue it normally supplies, termed an infarction. This can result in more enduring or even permanent impairments. These events describe an ischemic stroke. Under special circumstances, the local reduction in arterial blood flow may not produce an ischemic stroke and infarction because the tissue receives a redundant supply from another artery. This is termed collateral circulation, which is covered further in the section on the anterior and posterior circulations.
Hemorrhagic stroke can occur when an artery ruptures, thereby releasing blood into the surrounding tissue. A hemorrhagic stroke not only produces a loss of downstream flow but also can damage brain tissue at the rupture site because of the volume now occupied by the blood outside of the vessel. A common cause of a hemorrhagic stroke is when an aneurysm, or ballooning of an artery due to weakening of the muscular wall, ruptures.
The principal blood supply for the brain comes from two arterial systems that receive blood from different systemic arteries: the anterior circulation, fed by the internal carotid arteries, and the posterior circulation, which receives blood from the vertebral arteries (Figure 3–2, inset; Table 3–1). The vertebral arteries join at the junction of the medulla and pons (or pontomedullary junction) to form the basilar artery, which lies unpaired along the midline (Figure 3–2). The anterior circulation is also called the carotid circulation, and the posterior circulation, the vertebral-basilar circulation. The anterior and posterior circulations are not independent but are connected by networks of arteries on the ventral surface of the diencephalon and midbrain and on the cortical surface (see below).
FIGURE 3–2
Diagram of the ventral surface of the brain stem and cerebral hemispheres, illustrating the key components of the anterior (carotid) circulation and the posterior (vertebral-basilar) circulation. The anterior portion of the temporal lobe of the right hemisphere is removed to illustrate the course of the middle cerebral artery through the lateral (Sylvian) fissure and the penetrating branches (lenticulostriate arteries). The circle of Willis is formed by the anterior communicating artery, the two posterior communicating arteries, and the three cerebral arteries. The inset (bottom) shows the extracranial and cranial courses of the vertebral, basilar, and carotid arteries. Arrows indicate normal direction of blood flow.

Whereas the cerebral hemispheres receive blood from both the anterior and posterior circulations, the brain stem receives blood only from the posterior circulation. The arterial supply for the spinal cord is provided by the systemic circulation—which also supplies muscle, skin, and bones—and, to a lesser degree, by the vertebral arteries. Cerebral and spinal arteries drain into veins. Although spinal veins are part of the general systemic circulation and return blood directly to the heart, most cerebral veins drain first into the dural sinuses, a set of large venous collection channels in the dura mater.
The spinal cord receives blood from two sources. First are the anterior and posterior spinal arteries (Figure 3–2), branches of the vertebral arteries. Second are the radicular arteries, which are branches of segmental vessels, such as the cervical, intercostal, and lumbar arteries. Neither anterior nor posterior spinal arteries typically form a single continuous vessel along the entire length of the ventral or dorsal spinal cord. Rather, each forms a network of communicating channels oriented along the rostrocaudal axis of the spinal cord. The radicular arteries feed into this network along the entire length of the spinal cord.
Although the spinal and radicular arteries supply blood to all spinal cord levels, different spinal cord segments are preferentially supplied by one or the other set of arteries. The cervical spinal cord is supplied by both the vertebral and radicular arteries (in particular, the ascending cervical artery). In contrast, the thoracic, lumbar, and sacral segments are nourished primarily by the radicular arteries (the intercostal and lumbar arteries). When spinal cord segments are supplied by a single artery, they are particularly susceptible to injury after arterial occlusion. In contrast, segments that receive a redundant (or collateral) blood supply tend to fare better following single vessel occlusion. For example, individual rostral thoracic segments are supplied by fewer radicular arteries than are more caudal segments. When a radicular artery that serves the rostral thoracic segments becomes occluded, serious damage is more likely to occur because there is no backup system for perfusion of oxygenated blood. Interruption of the blood supply to critical areas of the spinal cord can produce sensory and motor control impairments similar to those produced by traumatic mechanical injury, such as that resulting from an automobile accident.
Each of the three divisions of the brain stem and the cerebellum receives its arterial supply from the posterior circulation (Figure 3–3A). In contrast to the spinal arteries, which are located both ventrally and dorsally, arteries supplying most of the brain stem arise from the ventral surface only. Branches emerge from these ventral arteries and either penetrate directly or run around the circumference of the brain stem to supply dorsal brain stem structures and the cerebellum. Three groups of branches arise from the vertebral and basilar arteries: (1) paramedian, (2) short circumferential, and (3) long circumferential (Figure 2–3B). The paramedian branches supply regions close to the midline. The short circumferential branches supply lateral, often wedge-shaped regions, and the long circumferential branches supply the dorsolateral portions of the brain stem and cerebellum.
FIGURE 3–3
A. Arterial circulation of the brain stem is schematically illustrated on a view of the ventral surface of the brain stem. B. Four transverse sections through the brain stem are shown, illustrating the distribution of arterial supply. In the upper medulla (B3), pons (B2), and midbrain (B1), portions of tissue from medial to dorsolateral are supplied by paramedian, short circumferential, and long circumferential branches. The caudal medulla receives its arterial supply from the vertebral and spinal arteries (B4). The dashed lines in A indicate the planes of section in B.

Even though the spinal arteries primarily supply the spinal cord, they also supply a small portion of the caudal medulla. The spinal arteries lie close to the dorsal and ventral midline and nourish the most medial areas (Figure 3–3B4). The more lateral area is served by direct branches of the vertebral arteries, which are equivalent to the more rostral short circumferential branches.
The rest of the medulla is supplied by the vertebral arteries. Small (unnamed) branches that exit from the main arteries supply the medial medulla (ie, paramedian and short circumferential branches). Because these arteries supply axons of the corticospinal tract and the medial lemniscus (see Figure 2–2), when the arteries become occluded, patients develop impairments in voluntary limb movement and mechanosensation. The major laterally emerging (long circumferential) branch from the vertebral artery, the posterior inferior cerebellar artery (PICA), nourishes the most dorsolateral region (Figure 3–3B3). This region of the medulla does not receive blood from any other artery. The absence of a collateral arterial supply makes the posterior inferior cerebellar artery particularly important because occlusion almost always results in significant tissue damage. When this occurs, patients commonly develop characteristic sensory and motor impairments due to destruction of nuclei and tracts in the dorsolateral medulla. Common neurological signs include loss of facial pain sensation and uncoordinated limb movements, both on the side of the occlusion, and loss of limb and trunk pain on the opposite side. An understanding of this complex pattern of sensory and motor loss will be achieved when pain and motor control circuits are described in later chapters.
The two vertebral arteries join to form the basilar artery at the pontomedullary junction (Figure 3–3A), from which paramedian and short circumferential arteries supply the base of the pons, where corticospinal fibers are located. The dorsolateral portion of the caudal pons is supplied by a long circumferential branch of the basilar artery, termed the anterior inferior cerebellar artery (AICA). The region in the pons rostral to that supplied by the anterior inferior cerebellar artery is nourished by the superior cerebellar artery, another long circumferential branch of the basilar artery (Figure 3–3A).
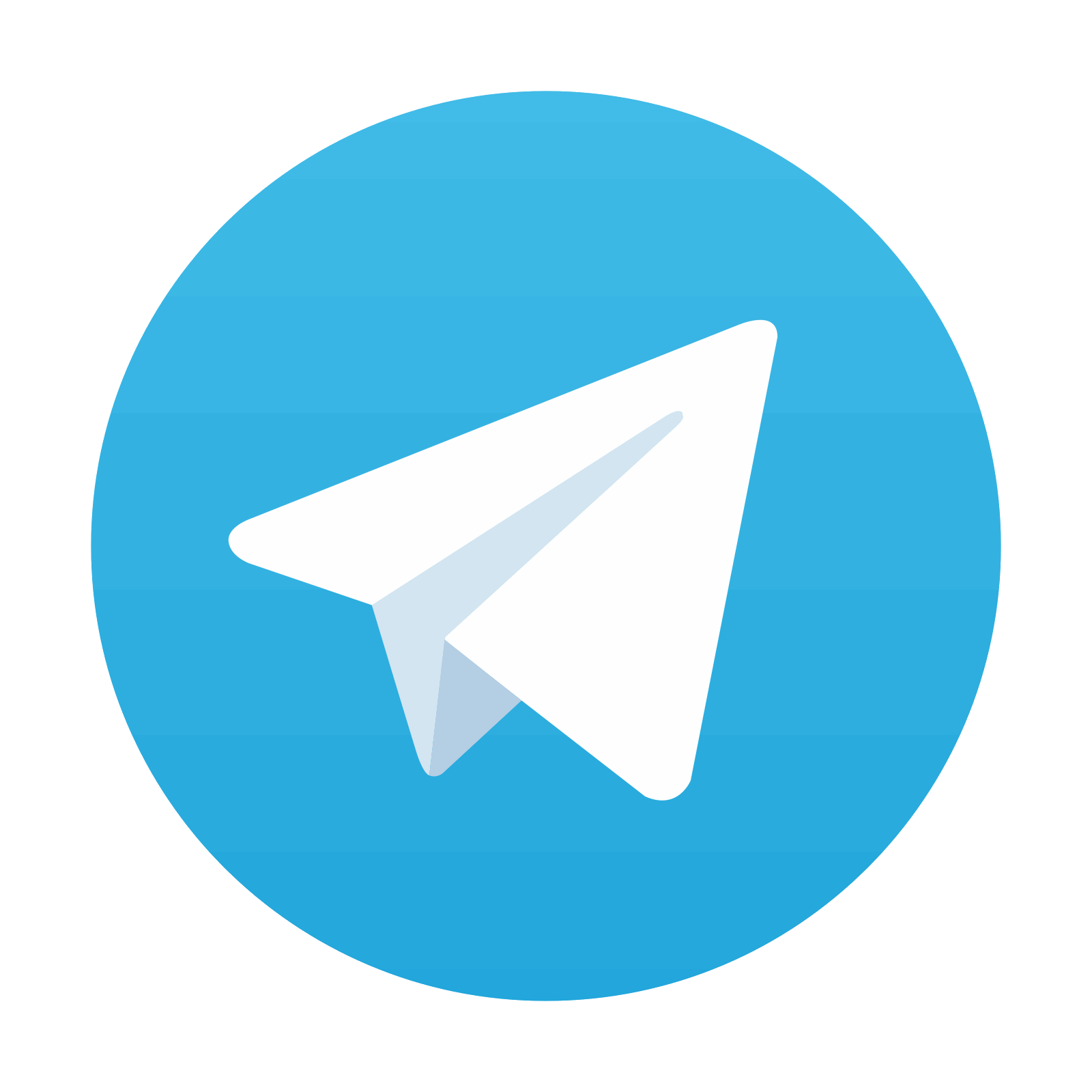
Stay updated, free articles. Join our Telegram channel

Full access? Get Clinical Tree
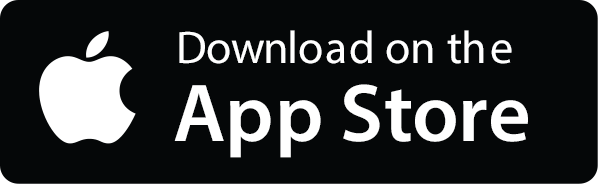
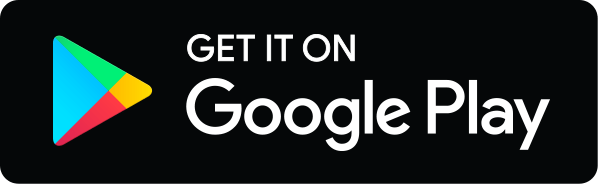