Study guidelines
- 1.
Describe the components of the static labyrinth and its primary role.
- 2.
Describe the components of the dynamic labyrinth and its primary role.
- 3.
Describe the head positioning used and the procedure for performing a warm water caloric test.
Introduction
The vestibulocochlear nerve is primarily composed of the centrally directed axons of bipolar neurons housed in the petrous temporal bone ( Figure 19.1 ). The peripheral processes are applied to neuroepithelial cells in the vestibular labyrinth and cochlea. The nerve enters the brainstem at the junctional region of the pons and medulla oblongata. The functional anatomy of the vestibular division of the nerve is described in this chapter.

Vestibular system
The bony labyrinth of the inner ear is a very dense bony shell containing perilymph , which resembles extracellular fluid in general. The perilymph provides a water jacket for the membranous labyrinth , which encloses the sense organs of balance and of hearing. The sense organs are bathed in endolymph . The endolymph resembles intracellular fluid, being potassium-rich and sodium-poor.
The vestibular labyrinth comprises the utricle , the saccule , and three semicircular ducts ( Figure 19.2 ). The utricle and saccule contain a 3 × 2-mm 2 macula . Each semicircular duct contains an ampulla at one end, and the ampulla houses a crista. (It should be pointed out that clinicians commonly speak of ‘canals’ where ‘ducts’ would be strictly more appropriate.)

The two maculae are the sensory end organs of the static labyrinth , which signals head position. The three cristae are the end organs of the kinetic or dynamic labyrinth , which signals head movement.
The bipolar cells of the vestibular (Scarpa) ganglion occupy the internal acoustic meatus. Their peripheral processes are applied to the five sensory end organs. Their central processes, which constitute the vestibular nerve , cross the subarachnoid space and synapse in the vestibular nuclei previously seen in Figures 17.14 and 17.15 .
Static labyrinth: anatomy and actions
The position and structure of the maculae are shown in Figure 19.3 . The utricular macula is relatively horizontal; the saccular macula is relatively vertical. The cuboidal cells lining the membranous labyrinth become columnar supporting cells in the maculae. Among the supporting cells are so-called hair cells , to which vestibular nerve endings are applied. Some hair cells are almost completely enclosed by large nerve endings, whereas others (phylogenetically older) receive only small contacts. At the cell bases are ribbon synapses , the synaptic vesicles being lined up along synaptic bars. Projecting from the free surface of each hair cell are about 100 stereocilia and, close to the cell margin, a single, long kinocilium . The hair cells discharge continuously, at a resting rate of about 100 Hz.

The cilia of the maculae are embedded in a gelatinous matrix ( otolithic membrane ) containing protein-bound calcium carbonate crystals called otoconia (‘ear sand’). The cilia ‘move’ as a unit when the otolithic membrane is displaced. (The term ‘otoliths’, when used, refers to the larger, ‘ear stones’ of reptiles.) The otoconia exert ‘gravitational drag’ on the hair cells. Whenever the stereocilia are deflected towards the kinocilium the hair cell is depolarised; deflection in the opposite direction results in hyperpolarisation of the hair cells. Each macula has a central groove ( striola ), and the hair cell orientations have a mirror arrangement in relation to the groove. Polarisation of the hair cells, with respect to the striola, results in the hair cells on one side becoming depolarised and those on the other side becoming hyperpolarised whenever the otolithic membrane is displaced.
The arrangement of the maculae allows them to be responsive to gravitational forces and to ‘communicate’ head position as well as linear acceleration. In response to this signal the vestibular nuclei initiate compensatory movements, with the effect of maintaining the centre of gravity between the feet (in standing) or just in front of the feet (during locomotion), and of keeping the head horizontal. These effects are mediated by the vestibulospinal tracts.
The lateral vestibulospinal tract , seen earlier in sections of medulla oblongata in Chapter 17 , arises from large neurons in the lateral vestibular nucleus (of Deiters) . The fibres descend in the ventral funiculus on the same side of the spinal cord and synapse upon extensor (lower extremity antigravity) motor neurons. Both α and γ motor neurons are excited, and a significant part of the increased muscle tone is exerted by way of the γ loop ( Chapter 16 ). During standing, the tract is tonically active on both sides of the spinal cord. During walking, activity is selective for the quadriceps motor neurons of the leading leg; this commences following heel strike and continues during the stance phase (when the other leg is off the ground). Deiters nucleus is somatotopically organised, and the functionally appropriate neurons are selected by the flocculonodular lobe of the cerebellum. The flocculonodular lobe ( Chapter 25 ) has two-way connections with all four vestibular nuclei.
Antigravity action is triggered mainly from the horizontal macula of the utricle. The vertical macula of the saccule, on the other hand, is maximally activated by a free fall . The shearing effect on the macula produces a powerful extensor thrust in anticipation of a hard landing.
A small, medial vestibulospinal tract arises in the medial and inferior vestibular nuclei ( Figure 17.6 ). It descends bilaterally in the medial longitudinal fasciculus and terminates upon excitatory and inhibitory interneurons in the cervical spinal cord. It operates head-righting reflexes , which serve to keep the head—and the gaze—horizontal when the body is craned forward or to one side. Good examples of head-righting reflexes are to be seen around pool tables and in bowling alleys. An added twist can be provided, if required, by torsion of the eyeballs (up to 10°) within the orbital sockets. This eye-righting reflex is mediated by axons contributed to the ascending medial longitudinal fasciculus from the superior and medial vestibular nucleus to reach nuclei controlling the extraocular muscles. These reflex movements of the eyes will be opposite to the direction of movement perceived by the vestibular system. Evidence derived from unilateral vestibular destruction ( Clinical Panel 19.1 ) indicates that the horizontal position of the eyes in the upright head is the result of a cancelling effect of bilateral tonic activity in these vestibuloocular pathways.
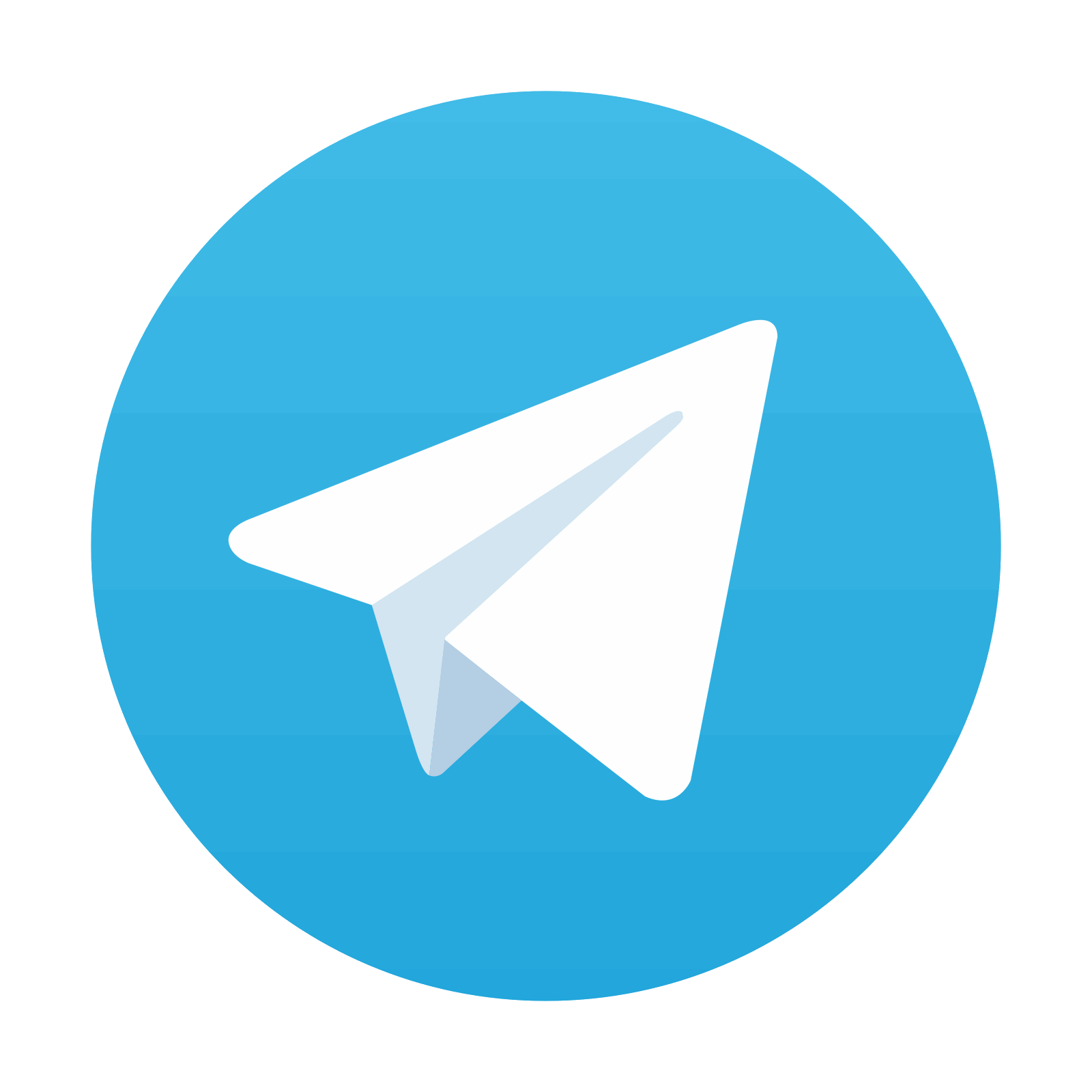
Stay updated, free articles. Join our Telegram channel

Full access? Get Clinical Tree
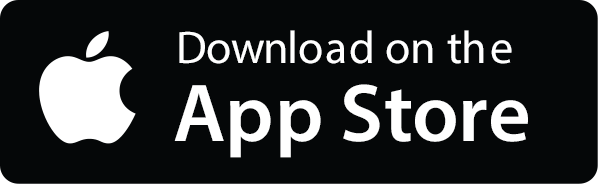
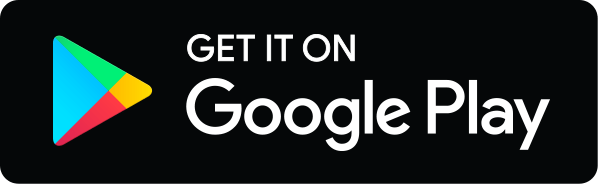
