Video-EEG Monitoring and Epilepsy Surgery
Vibhangini S. Wasade
Jules E. C. Constantinou
Long-term video-EEG monitoring with scalp electrodes is a well-established tool that permits time-locked correlation of paroxysmal clinical events with electrographic data. Video-EEG monitoring enables the accurate classification of seizures for optimal choice of medical therapy and, in those with medically intractable focal epilepsy, assists in the localization of the epileptogenic focus for possible surgical resection. Video-EEG monitoring is also helpful in monitoring the response to treatment of epilepsy. Seizure frequency can be documented in epilepsies with clinical manifestations that are fleeting or not always readily apparent, for example, in epilepsies characterized by typical and atypical absence seizures. Epileptiform activity in sleep can be monitored in the epileptic encephalopathies such as Landau-Kleffner syndrome and epilepsy with status epilepticus in sleep (ESES). Video-EEG monitoring also permits the distinction between epileptic and nonepileptic episodes, whether physiologic or psychogenic.
Approximately 30% of the epilepsies are medically intractable. According to the International League Against Epilepsy (ILAE), drug-resistant epilepsy is defined as failure of adequate trials of two appropriately chosen and tolerated antiseizure drugs (ASDs) at therapeutic doses to produce sustained seizure freedom.1 In the epilepsy clinic-based study of Kwan and Brodie, only 11% of patients with newonset seizures became seizure free with subsequent changes to the ASD regimen after a failed treatment response to the first ASD, if the initial treatment failure was not because of unacceptable side effects or an idiosyncratic response.2 In another clinic-based study of the efficacy of eight major new ASDs in patients with chronic epilepsy for at least 5 years, seizure-free rates of only 14% and 15% were noted after a second and third change to the ASD regimen. Although 28% of patients did become seizure free with manipulation of ASDs, patients with focal epilepsy were not distinguished from those with idiopathic generalized epilepsy, which is more readily amenable to drug treatment.3 The identification of a lesional etiology is also a major determinant of pharmacoresistance. In a large hospital-based study, only 11% of patients with hippocampal sclerosis and 24% with cerebral dysgenesis remained free of seizures for 1 year.4
In contrast, resective epilepsy surgery is a viable treatment option in those with intractable epilepsy. Epilepsy surgery results in seizure freedom in 70%-80% of those with temporal lobe epilepsy (TLE) and in 30%-50% of those with extratemporal epilepsy. Only two randomized controlled studies have compared the efficacy of surgical and medical treatment of mesial TLE. In the first, 58% of patients in the surgical arm became free of disabling seizures, as opposed to only 8% in the medical arm. There was a significant improvement in quality of life (QOL) scores in the surgical group, with no surgical mortality and infrequent morbidity.5 The subsequent Early Randomized Surgical Epilepsy Trial (ERSET) also demonstrated superiority of early surgical treatment in those with newly intractable TLE characterized by disabling seizures for no more than 2 years.6
Epilepsy surgery is underutilized. Between 100,000 and 200,000 individuals might benefit from epilepsy surgery in the United States. However, only 6200 cases of epilepsy surgery were reported between 2000 and 2013, according to the Centers for Medicare and Medicaid Services.7 Despite the clear evidence of the benefit of epilepsy surgery, there has been no increase in the utilization of epilepsy surgery during the 2000s.8 The lag time between onset of the epilepsy and surgery often spans decades.9 Medical intractability should be appreciated and epilepsy surgery considered earlier to minimize the psychosocial consequences of this chronic disorder.
This chapter will provide an overview of long-term video-EEG monitoring with scalp electrodes, with special reference to the evaluation for epilepsy surgery. Characterizing the clinical semiology of habitual seizures and the interictal and ictal electrographic patterns is the cornerstone of the evaluation. Magnetic resonance imaging (MRI), ictal-interictal single photon emission computed tomography (SPECT), positron emission tomography (PET) scan, neuropsychological data, Wada testing, and magnetoencephalography (see Chapter 20) provide important
corroborative information for defining the epileptogenic zone (EZ). The EZ is defined as the brain region from which seizures originate, or conversely, the region whose removal is necessary and sufficient to abolish seizures. It is important to determine the relationship of the EZ to the functional or eloquent cortex, so that excisional surgery can be carried out successfully without causing neurologic compromise.
corroborative information for defining the epileptogenic zone (EZ). The EZ is defined as the brain region from which seizures originate, or conversely, the region whose removal is necessary and sufficient to abolish seizures. It is important to determine the relationship of the EZ to the functional or eloquent cortex, so that excisional surgery can be carried out successfully without causing neurologic compromise.
REVIEW
13.1: What are the indications for epilepsy monitoring unit (EMU) evaluation?
View Answer
13.1: EMU evaluation is indicated (1) to classify seizures for optimal choice of medical therapy; (2) to localize the epileptogenic focus for possible surgical resection in those with medically intractable focal epilepsy; (3) to monitor the response to treatment of epilepsy; (4) to document seizure frequency in epilepsies with clinical manifestations that are fleeting or not always readily apparent, such as absence seizures; (5) to monitor epileptiform activity in sleep in the epileptic encephalopathies such as Landau-Kleffner syndrome, acquired epileptic aphasia, or epilepsy with status epilepticus in sleep, and (6) to distinguish between epileptic and nonepileptic episodes.
13.2: What are the commonly performed tests used as presurgical evaluation for epilepsy surgery in intractable partial epilepsy?
View Answer
13.2: The commonly performed tests used as presurgical evaluation for epilepsy surgery in intractable focal epilepsy include EMU evaluation, MRI brain, ictal-interictal SPECT or PET scan, neuropsychological evaluation and intracarotid amobarbital procedure (IAP), or Wada test.
THE EPILEPSY MONITORING UNIT
Cameras and EEG Equipment in Epilepsy Monitoring Unit
Guidelines regarding the requirements for long-term monitoring in epilepsy are provided by the ILAE and American Clinical Neurophysiology Society (ACNS).10,11
Digital technology has largely replaced analog equipment for the acquisition, storage, and retrieval of video and EEG. The digital recording system should be able to store at least 24 hours of video and 32-64 channels of EEG (30 gigabytes of information). Systems including up to 128 channels are now widely available for use with intracranial recordings. A server or other efficient data storage method is essential to manage the high volume of data.
Each patient’s suite in the epilepsy monitoring unit (EMU) is fitted with cameras, camera mounts, and audio systems for recording digital video. A camera with pan-and-tilt mechanisms remote-controlled from a central monitoring station allows staff to keep patients within view, and a remote zoom assists in maintaining focus and obtaining close-up images of subtle behaviors. Event markers or push button alarms are available in the patients’ rooms and in the monitoring station to call EMU staff for significant clinical episodes and to mark the record for subsequent review of that episode. Relevant clinical data can be annotated into the recording by an observer. EEG data is transmitted by flexible cable from the electrodes to the EEG amplifier, which performs analog to digital conversion of the acquired electrical signals.
Time synchronization of video and EEG signals is enabled by time-date generators in the computer software to facilitate accurate temporal correlation of clinical and electrophysiologic phenomena. Digitized EEG and video images can be displayed together on a single screen. Acquisition stations and review stations for data analysis are often separate. Software systems maintain fidelity of the EEG data between acquisition and review and enable the reviewer to change the montage and gain as needed during review of the EEG. Careful filtering may be necessary because of the movement and muscle artifact that frequently obscures the ictal electrographic trace. The reviewer should always be aware that high-frequency filtering to reduce muscle artifact also blunts epileptic spikes and may shift phase relationships between waveforms.
Automated digital spike and seizure detection systems, incorporated in the EEG software, use mathematical algorithms to identify abnormal electrical activity without changing the raw data. Putative spikes and seizures are automatically captured and stored for subsequent clinical review and verification. Although specificity is low because artifacts cause frequent false positives, digital spike and seizure detection has high sensitivity, on the order of 80%-90%, and is very useful as a timesaving measure for reviewing long-term video-EEG studies.12
Personnel/Setup
The National Association of Epilepsy Centers (NAEC) recommends that EMUs should have qualified EEG technologists and medical staff at all times.
Trained EEG technologists apply electrodes upon admission to the EMU and check electrodes impedances and the integrity of the recording system at least daily (Fig. 13.1). The EEG technologist should be able to identify clinical seizures as well as interictal and ictal electrographic patterns. Prescreening of data accumulated in the preceding 24 hours by the technologists facilitates daily physician review. Injection of radiotracer for ictal SPECT at seizure onset may also fall within the domain of the EEG technologist, but requires formal training in the safe handling of nuclear isotopes, and licensing rules vary from one state or country to another.
Nursing and other ancillary staff should also interact with patients during and after behavioral events to evaluate changes in responsiveness, language, and motor functions, which may provide valuable information about the seizure phenomenology. EMU staff should remember the mantra, “Say something, do something, remember something,” as a prompt to test verbal and behavioral responsiveness and memory. A standardized procedure for testing patients during and after seizures in the EMU has been proposed by a joint task force of the ILAE.13
Safety in the EMU
Patients in the EMU are at risks for seizure emergencies, injuries, and adverse events, underscoring the need for safety protocols.14 Because long-term video-EEG monitoring equipment is connected to an electrical power supply and makes direct contact with the patient, attention should be given to electrical safety. A brief review of these issues can be found in Chapter 3.
![]() FIGURE 13.1. Electrode impedance check being performed by a technologist in the epilepsy monitoring unit (EMU). |
Appropriate precautions should be taken to avoid seizure-related injuries and falls in the EMU and to recognize and treat seizures and other medical emergencies quickly. A risk assessment should be performed upon admission to the EMU. Choice of furnishings and flooring materials in the patient’s suite may minimize the risk of injury. Caretaker supervision can minimize risk when the patient is out of bed or in the bathroom. Padded bed rails may help prevent patient injury during a seizure. Posey or other forms of physical restraints may be necessary for patients who become confused, combative, or agitated peri-ictally or interictally or in patients with intracranial electrodes. Oxygen and suction should be readily available for postictal care and intravenous access maintained throughout the admission. Cribs and pediatric resuscitation equipment are necessary when children and infants are monitored.
Seizure emergencies, seizure clusters, or status epilepticus may be anticipated based on diagnosis or prior history. In our institution, an intravenous benzodiazepine is given if the patient experiences a single generalized tonic-clonic (GTC) seizure or more than three focal seizures with impaired awareness (formerly complex partial seizures) over an 8- to 12-hour period. An individualized rapid response plan for the care of status epilepticus is crucial. Twenty-four-hour clinical observation and medical coverage are essential to maximize patient safety.
REVIEW
13.3: Why are the safety measures necessary in EMU? What are the usual procedures to ensure patient safety?
View Answer
13.3: Safety measures are necessary in the EMU to prevent falls or injury during seizures, and appropriate protocols must be in place if seizure cluster or status epilepticus occurs. Safety measures include selecting furnishings to prevent falls, padding the patient’s bedrails, monitoring around the clock and ensuring that the patient is accompanied when out of bed, oxygen and suction availability, and plans to handle seizure or medical emergencies.
Electrodes
The 10-mm (6-mm in pediatric cases) cup-disc scalp electrodes are commonly made of silver or silver chloride (Fig. 13.2). Integrity of the electrode-scalp interface over the extended period of monitoring is maintained by covering the electrode cup and wire tail with collodion-soaked gauze, which firmly glues the electrode in place and is largely impervious to sweating. A 2-mm hole in the center of the cup provides easy access for paste or gel application to enhance electrode conductance. Electrode impedance should be kept at <5000 Ω. Scrupulous electrode maintenance is important, and electrodes should be regelled frequently. The electrode sites should be carefully monitored for the development of skin breakdown, which may limit the duration of video-EEG monitoring.
Scalp electrodes are placed according to the standard international 10-20 electrode placement system. Closer spacing of electrodes across using the 10-10 system is sometimes indicated, especially in the evaluation of epileptogenic foci involving mesial extratemporal regions or sensorimotor cortex. Eye movement, EKG, and other bioelectric channels should also be recorded.
Sphenoidal electrodes, touted to detect epileptiform activity in the anterior basal temporal lobe, are recommended by some in the evaluation of mesial TLE as standard temporal lobe scalp placements may be more sensitive to brain activity across the superior and middle temporal gyri. These flexible wire electrodes are inserted with needle guidance (Fig. 13.2) after sterilization and application of dermal anesthetic cream 2-3 cm anterior to the external auditory canal, about 3-4 cm below the zygomatic arch, through the mandibular notch. The needle is guided upward, backward, and mesially toward the floor of the middle cranial fossa in the
region of the foramen ovale to a depth of 4-5 cm or until the patient reports jaw pain.15 There is a risk of injury to the facial nerve, though this is rare.
region of the foramen ovale to a depth of 4-5 cm or until the patient reports jaw pain.15 There is a risk of injury to the facial nerve, though this is rare.
![]() FIGURE 13.2. Commonly used electrodes in the epilepsy monitoring unit (EMU). 10-mm cup electrodes (A) and sphenoidal electrode (B) within the needle for insertion of the electrode. |
The necessity of sphenoidal electrodes remains contentious. Minisphenoidal electrodes (sphenoidal electrodes advanced only a few millimeters) and zygomatic arch or T1/T2 electrodes may provide equivalent information.16
REVIEW
13.4: What types of electrodes are commonly used in EMU? Describe the procedure for placement of sphenoidal electrodes.
View Answer
13.4: Ten-millimeter cup electrodes are commonly used for scalp electrode placement in the EMU. Sphenoidal electrodes may be used in the evaluation of TLE, although their necessity remains contentious. After skin sterilization and local anesthetic cream, they are inserted 2-3 cm anterior to the external auditory canal, about 3-4 cm below the zygomatic arch, through the mandibular notch and toward the foramen ovale, about 4-5 cm deep.
EMU Evaluation
The patient history obtained on admission is helpful in forming a preliminary impression about the nature of the epilepsy. A history of febrile seizures in childhood, the occurrence of focal aware seizures (formerly simple partial seizures) in isolation, that is, without progression to focal seizure with impaired awareness, may suggest a temporal lobe focus. Evolution to bilateral convulsion occurs rarely. A predisposition for seizures to occur in the drowsy-sleep transition or on awakening, frequent evolution to bilateral convulsion, and seizure clustering may suggest an extratemporal focus. A history of early morning jerks may indicate juvenile myoclonic epilepsy (JME).
Psychogenic nonepileptic seizures (PNES) may be suspected when seizures are prolonged and/or associated with preservation of awareness despite full body movements.
Comorbid issues that frequently accompany epilepsy should also be recognized. Anxiety and depression may affect the ability of the patient to tolerate the long hospital stay. In those with a history of previous psychotic episodes, psychosis may occur with withdrawal of ASDs and induction of seizures. Mental retardation may make it difficult to obtain informed consent.
Patient expectations should be set at the start of the evaluation. The patient and family members should understand that the goal of evaluation is to record enough habitual seizures to make decisions about treatment options for the epilepsy. There is often a lag time, usually days but sometimes weeks, before seizures occur. In one study, the median time to the first diagnostic event, whether epileptic or nonepileptic, was 2 days; 35% required 3 or more days and 7% needed monitoring for more than a week.17 The epilepsy team should be sensitive to the rigors of inpatient monitoring and should preemptively address feelings of anxiety, isolation, and helplessness.
Family access is important, and in many centers, a couch is provided to allow other family members to stay with the patient. A common room wired to allow concurrent monitoring of several patients may help reduce isolation. Educational and recreation facilities should be provided for children. Social work resources should also be available.
Rate of Drug Withdrawal
Unless the patient is subject to very frequent seizures, ASDs are tapered and often completely withdrawn in order to record habitual seizures. In most centers, the ASD taper is started after patient admission for reasons of safety. On select occasions, for those with very infrequent seizures, withdrawing ASDs a few days before admission can be considered. In those with catamenial seizures, admission may be timed accordingly.
There are no absolute guidelines regarding the rate of ASD withdrawal, and protocols vary from institution to institution. Since abrupt withdrawal may precipitate seizures that are not habitual for the patient or increase the risk of convulsive seizures or status epilepticus, a slow taper in the dose of ASDs is generally recommended. As the majority of patients are on baseline treatment with more than one ASD, it is our practice to withdraw half the daily dose of a single ASD at about 1-2 day intervals. The pace of drug withdrawal is increased for those with infrequent seizures. Phenobarbital and benzodiazepines should be withdrawn with caution. The first recorded focal seizure with impaired awareness often coincides with a subtherapeutic, rather than an undetectable, serum ASD level.
Provocative Techniques
Patients tend to be more sedentary in the EMU and should be encouraged to be as active as possible in order to facilitate seizure onset. A low-set bedside exercise bike, used under supervision, may be helpful.
Hyperventilation and photic stimulation can induce absence and myoclonic seizures in those with primary generalized epilepsies and are most useful in these patients. These activation procedures may also infrequently induce focal seizures with impaired awareness and may also induce spells by suggestion in those with PNES.
Sleep deprivation may also be helpful in provoking seizures. In our unit, complete sleep deprivation every second or third night may be prescribed. In those with predominantly or exclusively sleep-related seizures, consistent sleep deprivation may help shift the sleep-wake cycle so that the patient sleeps and has seizures during the day. This may be essential for ictal SPECT injection and imaging, which in many centers can only be carried out during daytime hours.
In carefully selected patients, a small quantity of alcohol may be prescribed to trigger seizures. The administration of intravenous saline solution to patients with suspected PNES in order to precipitate or abort seizures has been a subject of ethical debate.
How Many Seizures to Record?
The number of seizures that should be recorded to inspire confidence in the clinical diagnosis (epileptic vs nonepileptic vs both) or the localization of seizure onset is individualized according to the questions to be answered for the specific patient.
In a patient with well-defined interictal spikes arising from only one temporal lobe, with corresponding mesial temporal sclerosis (MTS) and concordant clinical and electrographic ictal semiology, it is probably adequate to record only a few representative seizures.
In patients with bilateral and independent interictal temporal lobe spikes, inconsistent ictal onset patterns are more likely. Using statistical analysis, Blum has suggested that recording five concordant seizures excludes the possibility of seizures with conflicting localization with a 95% degree of confidence.18 A more recent analysis has suggested that 7-9 seizures should be recorded when there is a strong probability of multifocal seizures.19 Chronic ambulatory data from patients implanted with a responsive neurostimulation (RNS) device showed that only half of the patients with bilateral TLE revealed bilateral seizures within the 1-2 week period typically used for inpatient EEG monitoring.20
For patients with nonepileptic spells, it is not possible to prove that they do not also have epileptic seizures. Most epileptologists will be satisfied with a representative sample of all of the patient’s seizure semiologies, particularly if the baseline EEG is normal with no epileptiform activity.
REVIEW
13.5: Which provocative techniques may be carried out in EMU for induction of the patient’s habitual seizures?
View Answer
13.5: Provocative techniques that may be carried out in EMU to increase the likelihood of observing the patient’s habitual seizures include sleep deprivation, hyperventilation, photic stimulation, and supervised alcohol consumption in select patients with alcohol as a suspected trigger. The administration of intravenous saline solution to patients with suspected psychogenic nonepileptic seizures in order to precipitate or abort seizures is controversial.
CLASSIFICATION OF SEIZURE TYPES
The division of the focal epilepsies into temporal and extratemporal groups is fundamental to the presurgical evaluation and to the surgical approach.
Clinical Seizure Semiology
Medial or limbic temporal lobe epilepsy (MTLE) should be distinguished from lateral or neocortical temporal lobe epilepsy (NTLE). In some cases, seizure onset
simultaneously implicates both mesial and lateral structures (dual pathology). There is emerging recognition of the entity of temporal “plus” epilepsy. There is a wider epileptogenic network that includes the temporal lobe and nearby brain regions such as the orbitofrontal cortex, insular cortex, frontoparietal operculum, or the temporoparietal-occipital junction. Failure to separate medial and lateral temporal lobe seizures or to recognize the possibility of a wider epileptogenic network may account for persistent seizures in some individuals after standard temporal lobectomy.
simultaneously implicates both mesial and lateral structures (dual pathology). There is emerging recognition of the entity of temporal “plus” epilepsy. There is a wider epileptogenic network that includes the temporal lobe and nearby brain regions such as the orbitofrontal cortex, insular cortex, frontoparietal operculum, or the temporoparietal-occipital junction. Failure to separate medial and lateral temporal lobe seizures or to recognize the possibility of a wider epileptogenic network may account for persistent seizures in some individuals after standard temporal lobectomy.
Focal seizures of temporal lobe onset are typified by an aura consisting of an epigastric sensation, fear or dreamy state with progression to a period of behavioral arrest, impaired responsiveness with oroalimentary automatisms, and dystonic limb posturing, often in a stereotyped and choreographic sequence (see Video 13.1).
An initial epigastric sensation, fear, or anxiety may help differentiate MTLE, with or without lateral temporal involvement, from pure NTLE. The aura, however, may be absent in bilateral MTLE, possibly due to a transient memory deficit resulting from rapid propagation to the contralateral mesial temporal lobe.21 Visual, auditory, or vestibular hallucinations are correlated with NTLE.
In classic MTLE, consciousness is usually preserved at seizure onset, and loss of interaction typically occurs late (mean delay 43.9 seconds) correlating with secondary propagation to the neocortex.22 On the other hand, loss of awareness at the very beginning of seizure, when it occurs, may be specific for involvement of the lateral temporal cortex either in isolation or as part of a mesial-lateral temporal neocortical network.
Oroalimentary, upper limb and verbal automatisms, such as humming, suggest involvement of a widespread limbic-neocortical temporal network. In pure MTLE, these typically develop during the second half of the seizure. Early onset of these automatisms thus suggests a seizure onset zone involving the lateral temporal cortex, either in isolation or in a mesial-lateral temporal neocortical network (see Video 13.2). In contrast, upper limb tonic posturing and head and eye deviation, whether they occur early or late in the seizure, are not helpful in separating MTLE from NTLE.
Clinical seizure semiology may also be helpful in lateralizing the temporal lobe seizure focus. Preservation of speech during a seizure suggests involvement of the nondominant temporal lobe, while the loss of speech and postictal aphasia suggest involvement of the dominant temporal lobe. Automatisms with retained responsiveness lateralize to the nondominant hemisphere.23
Dystonic posturing of one arm in either flexion or extension (often with a rotational component) when accompanied by automatisms of the other hand is highly suggestive of seizure onset in the hemisphere contralateral to the dystonic arm. Unilateral hand automatisms not accompanied by dystonia of the opposite upper extremity, however, do not reliably predict the side of the seizure onset and are ipsilateral to the seizure focus only in about 60% of cases.24
Head version, a sustained forceful contralateral deviation of the head, occurs late in the course of temporal lobe seizures. When head version occurs immediately before secondary generalization, it has extremely high lateralizing value to the contralateral hemisphere. This forced versive head deviation may be preceded by a less forceful and less well-sustained head turn toward the seizure focus. On the other hand, an extratemporal focus over the contralateral hemisphere should be suspected with early versive head deviation.25
In unilateral TLE, postictal nose wiping performed exclusively with one hand is highly predictive (92%) of seizure onset ipsilateral to the hand used, especially when it occurs repetitively26 and within 60 seconds of seizure end. Ictal or postictal coughing can occur in both temporal- and extratemporal-onset seizures. When consistently present, ictal cough is predictive of temporal lobe onset seizures but does not help with lateralization.
Conflicting lateralizing phenomena during the seizure may suggest that the MTLE is of bilateral onset.27 Postictal unresponsiveness with bilateral onset MTLE seizures often lasts longer than 5 minutes.27
Extratemporal epilepsy can arise from the frontal, parietal, or occipital lobes or the insular cortex. The epileptogenic network may extend across anatomical boundaries.
Frontal lobe (FL) seizures, the most common extratemporal epilepsy, are variable in clinical manifestations. Attempts to correlate clinical seizure semiology with the precise site of origin may be frustrated because FL seizures tend to spread rapidly. It is important to bear in mind that the EZ from which seizure originates may be distant from the “symptomatogenic zone” that generates the clinical semiology. Nevertheless, inferences about the region of epileptogenesis can be drawn from analysis of the clinical phenomenology. Focal clonic seizures, particularly Jacksonian seizures that migrate from one body region to the next, as well as seizures associated with unilateral grimacing, imply capture of the contralateral primary motor cortex. Frontal eye field involvement in the premotor areas results in contralateral head and eye version. Tonic manifestations are generated from widely dispersed areas of the FL, including the premotor cortex and the supplementary motor cortex (SMA), but are often bilateral because of the anatomic representation of these areas of the brain. The “M2e” fencing posture of Ajmone-Marsan and Ralston consists of abduction and external rotation of the one arm with flexion at the elbow, with hand open or clenched, associated with head version and eye deviation so that the patient seems to be looking at the hand. The M2e posture is highly suggestive of seizures beginning or spreading to the SMA contralateral to the posturing arm. Forced vocalization or speech arrest may follow.28 Unilateral eye blinking, which may be associated with frontal and/or temporal seizures, is ipsilateral to the side of seizure onset.29
Classical FL seizures, so-called hypermotor seizures, are associated with frenetic, agitated bilateral body movements and early bilateral motor movements
in the legs and arms (pelvic thrusting, bicycling, and running). Complex gestural automatisms may also occur, and vocalization is often prominent (see Video 13.3). Bizarre behavior may mimic a nonepileptic seizure. Seizures are typically brief with a minimal postictal state. Seizures are sleep-related with a propensity for rapid secondarily generalization. However, the occurrence of hypermotor seizures is not strongly localizing within the FL as dorsolateral frontal, frontopolar, orbitofrontal, and opercular-insular EZs have been documented.30
in the legs and arms (pelvic thrusting, bicycling, and running). Complex gestural automatisms may also occur, and vocalization is often prominent (see Video 13.3). Bizarre behavior may mimic a nonepileptic seizure. Seizures are typically brief with a minimal postictal state. Seizures are sleep-related with a propensity for rapid secondarily generalization. However, the occurrence of hypermotor seizures is not strongly localizing within the FL as dorsolateral frontal, frontopolar, orbitofrontal, and opercular-insular EZs have been documented.30
Ictal pouting with an inverted smile and puckering of the lips in a “chapeau de gendarme” (the semicircular shape of the gendarme hat in the time of Napoleon Bonaparte) implicates mesial frontal regions, especially the anterior cingulate.31 Mirthless laughter and fear with or without a concordant facial expression may occur early.
During secondary generalization, extension of one arm forward from the body with flexion of the other elbow (bringing the hand on that side toward the extended elbow) often occurs during the tonic phase and is known as the “figure of 4” sign. Seizure onset is generally on the side contralateral to the extended arm, although recent literature provides contradictory data.32 Asymmetric cessation of the clonic phase of a secondarily generalized seizure lateralizes seizure onset ipsilateral to the side of the last clonic jerk.33
Complex motor and hypermotor activity is not invariable with FL epilepsy (FLE), and the seizures may consist of a brief period of behavioral arrest and staring, mimicking an absence seizure. Eyelid retraction may occur at the onset.34 Subtle vocalizations, slight turning of the head and the eyes, body rocking, and recollection of losing train of thought are reported. Such “pseudo absences” have been associated with a mesial frontal35 or frontopolar focus. Moreover, oro-bucco-lingual automatisms and unilateral arm dystonia are not specific for temporal lobe seizures and may also occur in FL or other extratemporal seizures, tending to appear later in the sequence as a result of extratemporal to temporal propagation.34
Parietal and occipital lobe epilepsies may also have markedly heterogeneous clinical expression as there may be preferential pathways of propagation into the temporal or frontal lobes. Simple or formed visual hallucinations at onset typify an occipital lobe focus. Eye blinking and head and eye deviation (usually contralateral) may occur at the beginning of the seizure. Parietal seizures often have a somatosensory aura consisting of tingling; numbness or pain, usually contralateral to the seizure focus but sometimes bilateral; or even a disturbance of body image. A well-localized distal sensory aura associated with a sensory march (analogous to the jacksonian march of progressive focal motor seizures in the frontal lobe) is highly characteristic of parietal lobe epilepsy.36 Vestibular hallucinations that are strikingly vertiginous have also been reported.
Insular seizures may mimic temporal, frontal, or parietal seizures depending on pathways of spread. The aura may include somatosensory sensations of tingling, warmth, or electrical feeling involving the limbs, typically in a large area, or the perioral region including the tongue and throat. Painful paresthesias point to ictal onset in the posterior insula. Viscerosensory (abdominal or epigastric sensation, chest tightness, throat tightness, constriction, or choking), visceromotor (gagging, vomiting, and belching), and vegetative and psychic symptoms (breathlessness, panic, or anxiety) may also occur and suggest anterior insula involvement. As the seizures progress, there may be behavioral arrest and oroalimentary and manual automatisms mimicking a temporal lobe seizure or complex hypermotor behavior reflecting FL propagation.37
Interictal Electrographic Patterns
Interictal epileptiform activity in patients with MTS is typically restricted to the anterior temporal derivations (F7-T7/F8-T8) with a maximum at the ipsilateral sphenoidal electrode (Sp1/Sp2)38 (Fig. 13.3A and B). Sharp wave discharges, activated by drowsiness and sleep, may be associated with a “blunted” configuration and at times may occur in brief semirhythmic salvos. Temporal lobe epileptiform discharges should be differentiated from benign variants such as wickets, which typically dissipate in deep sleep, in contrast to epileptiform activity, which may increase in sleep. Focal rhythmic slowing in the delta range across the temporal lobe (TIRDA) may reflect rudiments of spike-and-slow-wave activity confined to the hippocampal regions, for which the spike elements are not well propagated to the scalp.
Strictly unilateral interictal spikes, which are concordant with MRI findings of hippocampal atrophy, accurately predict localization of ictal scalp rhythms. In a study of 118 seizures in 24 patients with MTLE with unilateral hippocampal atrophy on MRI, and unitemporal interictal spikes, lateralization was possible in 88.4%-92% of seizures and was always concordant with the side of the interictal spike and of the hippocampal atrophy.39 Unilateral interictal spikes also correctly lateralize seizure onset in 80%-85% of patients with lesional neocortical TLE.40
Temporal lobe spikes with a sphenoidal maximum may also occur in neocortical TLE, however, and may not be useful in distinguishing MTLE from neocortical TLE.40 Pure MTS is less likely when there is posterior temporal propagation, with shifting midtemporal (T3/T4) or posterior temporal (T5/T6) discharges, or widespread extratemporal propagation of the temporal lobe discharges. Dual pathology is also possible, in which both the mesial and neocortical temporal lobe structures are intrinsic to the epileptic network, or there may be a pure neocortical focus that can be either temporal or extratemporal.38
In one-fifth to one-third of people with intractable TLE, interictal epileptiform discharges (IEDs) occur bilaterally. About 70%41,42 are found to have exclusively or predominantly unilateral temporal lobe seizure onset with intracranial monitoring; hence successful temporal lobectomy remains possible.
Absence or paucity of IEDs is a hallmark of frontal lobe epilepsy (FLE), especially in patients with mesial frontal or “Jacksonian” seizures.43 Lateralized interictal spikes usually point to the side of abnormality. The spikes may be limited to the frontal lobe but may also occur in a widespread regional or multilobar distribution. EEG analysis using transverse montages and the Fz, Cz, and Pz electrodes is critical to detect the midline spikes of mesial FLE, which often exhibit a restricted field. Focal spikes may reflect activity in the contralateral frontal lobe resulting in false lateralization, particularly near the frontopolar region. Spikes may also be restricted to the temporal lobe. Generalized spike-wave discharges and secondary bilateral synchrony are characteristic but not specific for mesial FLE (Fig. 13.4). By contrast, bilateral spikes occurring synchronously are rare in TLE.
Ictal Electrographic Patterns
Even though interictal EEG provides important localizing information, the ictal EEG recording is considered critical for the localization of the EZ.
Electrographically, focal seizures of temporal lobe onset commonly begin with background attenuation, which may be localized or diffuse. Abatement of IEDs, repetitive ictal spiking, and uncommonly rhythmic beta patterns are seen less frequently, and more than one pattern may be seen in the same patient. Temporal lobe auras, with or without progression to a seizure with impaired awareness, often show no ictal electrographic changes on scalp EEG.
The electrographic signature of the temporal lobe seizure consists of sustained rhythmic “recruiting” patterns, most often in the theta but occasionally in the alpha or delta frequency range. As the seizure evolves, ictal rhythms merge in a continuum and characteristically build in amplitude as the frequency decreases (Fig. 13.5). If the discharge does not clearly begin on one side, lateralization is still possible when there is a 2:1 ratio of the amplitude of the seizure discharge between sides, using a referential Pz montage. To localize ictal patterns definitively to a particular temporal lobe in a longitudinal bipolar montage, the amplitude of the ictal rhythms across the temporal chain should be more than double that across the ipsilateral parasagittal chain.
This temporal theta-alpha rhythm may occur at ictal onset but more commonly develops later in the evolution of the seizure,44 generally within 20-30 seconds of clinical onset. A delay of the regional temporal rhythm of more than 30 seconds may occur but should suggest the possibility of seizure propagation from the contralateral temporal lobe or from extratemporal regions.
In patients with unilateral interictal discharges, a localized or lateralized ictal theta discharge is identified in 80%-90% of cases.45 Lateralized or localized ictal patterns are observed less frequently in patients with bitemporal independent IEDs. Moreover, bitemporal IEDs make lateralized ictal EEG (recorded with scalp electrodes) less likely to predict the side of surgery, as determined by subsequent intracranial electrode monitoring (IEM).44
Analysis of the evolution of ictal patterns is also important. Switching or flipflopping of temporal ictal recruiting rhythms from one hemisphere to the other, or asynchrony between bitemporal ictal rhythms, often indicates independent bitemporal epileptogenicity and lowers the threshold for IEM. Postictal slowing can be helpful as a lateralizing tool, as it can be more pronounced on the side of ictal onset.
REVIEW
13.6: Describe the EEG hallmarks of temporal lobe epilepsy (TLE).
View Answer
13.6: The electrographic hallmark of the temporal lobe seizure is a sustained rhythmic recruiting pattern, most often in the theta and occasionally in the alpha range. As the seizure evolves, ictal rhythms merge in a continuum and characteristically build in amplitude as the frequency decreases, often with slowing to the delta range.
Analysis of ictal electrographic rhythms is more challenging in the extratemporal epilepsies because of the often-widespread nature of the epileptogenic focus and the propensity to rapid propagation and generalization. Inaccessibility of much of the cortex to surface electrodes is another key issue. Electrode and myogenic artifact resulting from the prominent motor activity and agitation that accompany frontal lobe seizures may obscure the ictal trace. Often, there is no discernible electrographic ictal change, and it is the marked behavioral and clinical stereotypy and the consistency of the time course of the seizure that establish the diagnosis of frontal lobe seizures, rather than the electrographic findings.
When ictal electrographic changes are evident, generalized patterns reflecting secondary bisynchrony are significantly more common in extratemporal epilepsy, particularly in mesial FLE and perhaps also in occipital lobe epilepsy. Generalized ictal patterns should be carefully evaluated for a consistent temporal lead, sometimes of the order of milliseconds, or a consistent voltage predominance favoring one hemisphere, as these findings might suggest a rapidly generalized extratemporal focus.
Localizable ictal electrographic changes are evident in less than one-third of cases of extratemporal lobe epilepsy (ETLE).43,46,47 Recent studies, however, have demonstrated discrete or regional ictal EEG changes in 23%-63% of those with
FLE, 70% of those with occipital lobe epilepsy, and 10%-55% of those with parietal lobe epilepsy.48,49
FLE, 70% of those with occipital lobe epilepsy, and 10%-55% of those with parietal lobe epilepsy.48,49
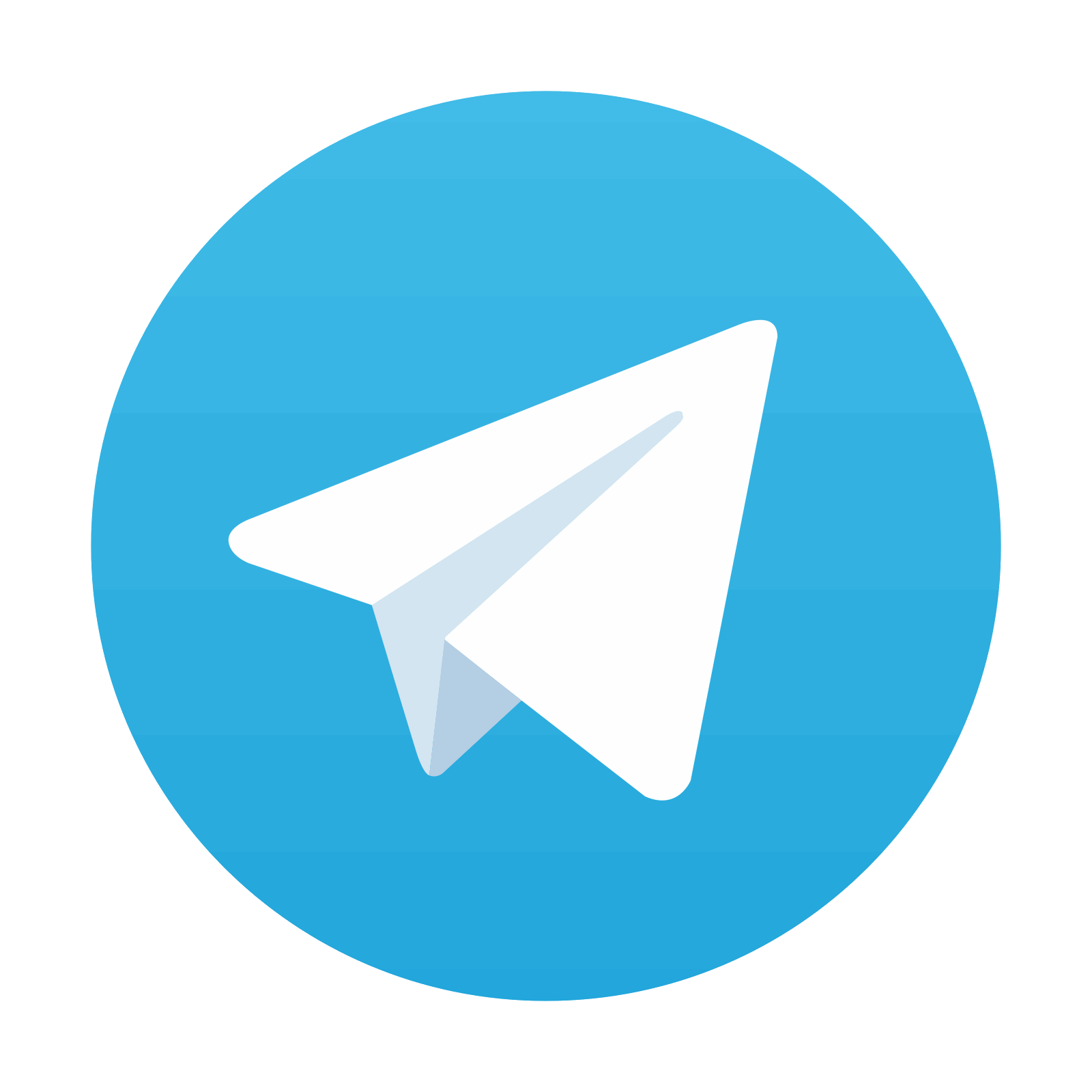
Stay updated, free articles. Join our Telegram channel

Full access? Get Clinical Tree
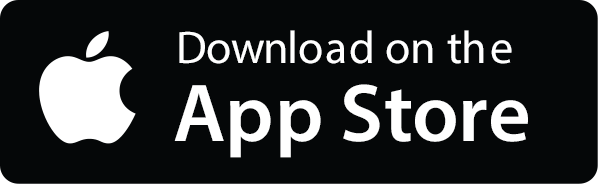
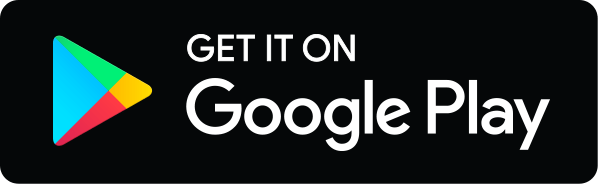