Brain tumors are a leading cause of mortality and morbidity in the United States. Malignant brain tumors occur in approximately 80,000 adults. Furthermore, the average 5-year survival rate for malignant brain tumors across all ages and races is approximately 30% and has remained relatively static over the past few decades, showing the need for continued research and progress in brain tumor therapy. Improved techniques in molecular biology have expanded understanding of tumor genetics and permitted viral engineering and the anticancer therapeutic use of viruses as directly cytotoxic agents and as gene vectors. Preclinical models have shown promising antitumor effects, and generation of clinical grade vectors is feasible. In parallel to these developments, better understanding of antitumor immunity has been accompanied by progress in cancer immunotherapy, the goal of which is to stimulate host rejection of a growing tumor. This article reviews the intersection between the use of viral therapy and immunotherapy in the treatment of malignant gliomas. Each approach shows great promise on its own and in combined or integrated forms.
Brain tumors are a leading cause of mortality and morbidity in the United States. Malignant brain tumors occur in approximately 80,000 adults. Furthermore, the average 5-year survival rate for malignant brain tumors across all ages and races is approximately 30% and has remained relatively static over the past few decades, showing the need for continued research and progress in brain tumor therapy. Improved techniques in molecular biology have allowed expansion in understanding of tumor genetics and have permitted viral engineering and the anticancer therapeutic use of viruses as directly cytotoxic agents and as gene vectors. Preclinical models have shown promising antitumor effects, and generation of clinical grade vectors is feasible. In parallel to these developments, better understanding of antitumor immunity has been accompanied by progress in cancer immunotherapy, the goal of which is to stimulate host rejection of a growing tumor. This article reviews the intersection between the use of viral therapy and immunotherapy in the treatment of malignant gliomas. Each approach shows great promise on its own and in combined or integrated forms.
This article initially reviews the fundamental concepts of viral therapy for brain tumors and traces how these have developed. Next, how viruses, wild-type and oncolytic, interact with the immune system is examined, followed by how immunomodulation, both positively and negatively, can augment antitumor effects of viruses. The final sections briefly review how the immune-modifying capacity of an oncolytic virus can be used to enhance a standard immunotherapy vaccine approach.
Viral therapy for brain tumors
Viral therapy for brain tumors can be considered in two main categories: (1) the use of replication-defective viruses, which do not multiply or propagate progeny at the site of inoculation, and (2) the use of replication-selective viruses, which divide in tumor cells and lyse them (oncolysis), and whose progeny infect and kill neighboring cells, continuing the cycle. Although these approaches have yielded promising data in preclinical studies, more recent work, particularly in combination with immunotherapy, has focused on the use of oncolytic (replicating) viruses. The therapeutic effect of replication-defective viral vectors is not produced through direct killing, but rather through the expression of transgenes.
The types of genes inserted and expressed can be divided into five categories based on mechanism of purpose :
- 1.
Correction of genetic defects in cancer, such as replacement of wild-type p53 in glioma, which often contains p53 mutations.
- 2.
Expression of antiangiogenic gene products, such as decoy vascular endothelial growth factor receptors.
- 3.
Immune-modifying genes, particularly cytokines, designed to stimulate the immune system.
- 4.
Drug resistance–modifying genes that help prevent the development of resistance to commonly used chemotherapeutics.
- 5.
Prodrug-activating enzymes that enhance chemotherapeutic targeting of tumors.
Challenges in treating malignant brain tumors with replication-defective viruses have included delivering the agent to a sufficient number of cells within a tumor and in achieving adequate viral titers. For example, retrovirus was initially a commonly used vector in cancer gene therapy models because it infects actively mitotic cells (eg, tumor cells and tumor-associated vascular endothelium).
To increase the intratumoral retroviral load, vector-producing cells (VPCs), typically fibroblasts, have been infected with retrovirus and implanted intratumorally, with the goal of generating higher and effective titers in the tumor microenvironment. However, initial studies showed very little spread of the virus through the tumor, with activity limited to a small zone at the injection site. More recent advances, particularly with alternative vectors such as adenovirus and lentivirus, have streamlined this approach, but significant limitations in transduction efficiency remain. Contributing factors include a lack of expression on many tumors of the adenovirus receptor (eg, Coxsackie adenovirus receptor [CAR]) and the natural host immune response against the vectors, as is discussed later.
In contrast, oncolytic viruses infect and kill tumor cells, selectively allowing spread of viral progeny into adjacent tumor cells. In 1991, Martuza and colleagues showed that a genetically engineered herpes simplex virus type 1(HSV-1) effectively and safely treated xenografted glioma tumors in mice. Since then, several oncolytic viruses have been studied and used in preclinical models and patients who have gliomas. These viruses are modified to replicate selectively in tumor cells, conferring specificity and safety.
Although their principal effect may be through direct oncolysis, oncolytic viruses may be designed to simultaneously deliver gene products that enhance antitumor activity. The most commonly used oncolytic virus backbones have been adenovirus and HSV-1. Clinical trials in malignant glioma have also been performed with reovirus.
Human adenoviruses belong to a family of nonenveloped DNA viruses, of which serotypes Ad5 and Ad2 have been most commonly studied and used in oncolytic therapy. Adenoviruses bind to cells through a fiber protein and penton base, which mediate cell-binding by way of specific receptors on cells, depending on the serotype. A common receptor for many oncolytic adenoviruses is CAR, which is expressed on human glioma, thereby allowing efficient transduction of these tumor cells. Onyx-015 is an early-developed oncolytic adenoviral vector. Onyx-015 carries a mutation in the E1B gene, the 55kD product of which inactivates normal p53 function in cells, thus resulting in a replication-selective oncolytic virus. Initial studies of this mutated virus suggested that it can replicate selectively in tumor cells deficient in p53 function, which is a common feature in many tumors, including gliomas. Further investigation has shown that Onyx-015 tumor selectivity may actually be conferred through tumor substitution of another E1B function; that is, late viral mRNA export.
Delta-24 adenovirus is a well-developed vector currently in clinical trials for glioma ( http://clinicaltrials.gov/ct2/show/NCT00805376 , accessed June 3, 2009). Delta-24 adenovirus has a 24-bp deletion in the viral gene E1A . The E1A gene product normally stabilizes the Rb protein so that E2F (a transcription factor) is released from E2F–Rb complexes and is free to activate the E2 promoter of the adenovirus, and several other cell-cycle genes.
In cells with normal Rb function, the viral gene E1A allows wild-type adenoviral infection and replication. Normal cells are, however, resistant to infection by Delta-24 adenovirus. The E1A mutation prevents binding of Rb and the subsequent transcription of viral genes by way of E2F in normal cells. In tumor cells that are defective in Rb and its upstream tumor suppressor, p16, Delta-24 is able to replicate, which is the basis for its tumor cell selectivity. Enhancements related to this basic premise have been made to increase selectivity for glioma cells.
HSV-1 is a particularly well-developed asset as an oncolytic virus against brain tumors. HSV-1 is an enveloped, double-stranded DNA virus with several advantages for use in gene and oncolytic therapy: (1) it is a large genome suitable for insertion of foreign genes; (2) its tropism for neural cells; (3) it has a safety mechanism in its sensitivity to agents such as ganciclovir; (4) high titers can be generated; and (5) it does not integrate into the host genome, so it is unlikely to be oncogenic.
The first engineered HSV-1 oncolytic virus had a mutation in the viral thymidine kinase (TK) gene, and showed killing of glioma cells in vitro and in in vivo models of glioma. Tumor specificity of this mutant is achieved through the virus’ dependence for replication on up-regulated human TK, present in rapidly dividing tumor cells. Viral TK is the substrate for the antiviral efficacy of nucleoside analogs, such as ganciclovir, which interrupts DNA synthesis when incorporated as ganciclovir triphosphate.
HSV-TK is much more efficient than human nucleoside kinases at monophosphorylating ganciclovir, which is subsequently converted by other cellular kinases into the toxic triphosphate form. Ganciclovir, therefore, has specificity against the HSV, which serves as a potential “shut-off” mechanism. Transduction of tumor cells with the HSV-TK gene (either through HSV itself or other vectors such as adenovirus) in conjunction with systemic ganciclovir administration is a potent mechanism for tumor killing, with important immune consequences.
The inability to use nucleoside analogs as a safety mechanism for this first-generation viral TK–mutated vector raised significant safety concerns, and, in fact, neurotoxicity was seen at high doses. In an alternative HSV-1 vector, a mutation in the γ 1 34.5 gene was introduced. The γ 1 34.5 gene and its product, ICP34.5, allow normal HSV to subvert the host’s shut-off response against infection.
Once infected with HSV, a normal cell will activate protein kinase R (PKR), which in turn phosphorylates and inactivates eukaryotic initiation factor-2α (eIF-2α), thereby shutting down protein synthesis in the normal host cell. ICP34.5 restores protein synthesis through activating protein phosphatase-1α which dephosphorylates and restores eIF-2α function. Mutations in this gene, γ 1 34.5 , result in an HSV that cannot replicate in normal cells, which abrogate protein-synthesis machinery. In malignant cells, however, the activation of PKR is less pronounced, likely because of other mutations, and therefore the ICP34.5-mutant HSV can selectively replicate.
A second mutation was added that confers tumor selectivity—the interruption of the gene for the large subunit of ribonucleotide reductase (ICP6). Double-mutant viruses are theoretically safer because the chances of in vivo recombination and restoration of wild-type HSV phenotype are decreased. This second-generation virus, termed G207 , harbors an insertion of the Escherichia Coli lacZ gene into the ICP6 gene, which also allows immunohistochemical detection of the virus in tumor cells. Using the G207 backbone, a third-generation oncolytic HSV-1, G47Δ, was developed through deleting the viral α47 gene. α47 encodes a protein that inhibits transporter associated with antigen presentation (TAP). Wild-type HSV-1 evades immune detection partially through inhibiting TAP in infected cells, thereby interfering with peptide assembly with major histocompatibility complex class I (MHC-I) molecules in the endoplasmic reticulum and preventing antigen presentation.
Recently, bacterial artificial chromosome (BAC)–mediated systems have improved the efficiency of inserting transgenes into the HSV-1 backbones, including with G47Δ, which may have particular relevance for immunotherapy.
Immune compartments, brain tumors, and viruses
Recently, understanding of cancer immunology has advanced dramatically. A model illustrating the dynamic and complex relationship between tumor cells and the immune system is that of cancer immunoediting. In this framework, newly transformed cancer cells are initially subject to immunosurveillance, which may result in their elimination. In response, developing tumor cells may acquire mutations that allow them to avoid being fully eliminated, but they remain quiescent, existing in a tenuous equilibrium state, because the immune response prevents uncontrolled proliferation and spread.
Eventually, this balance is tipped in favor of the malignant cells, because they secrete cytokines or cause proliferation of cellular subsets that result in immunosuppression and ultimately immune escape. For instance, mechanisms of escape may include secretion of soluble natural killer (NK) cell ligands, recruitment and activation of regulatory T cells, and release of immunosuppressive cytokines.
Human immunity can be broadly divided into innate and adaptive arms. Innate immunity is antigen-nonspecific, provides a crucial barrier against many foreign antigens, and stimulates the development of adaptive immunity. Adaptive immunity is specific to certain antigens and includes direct cell killing through specifically activated cytotoxic lymphocytes, and activation of lymphocytes that generate specific antibody responses. Antigen-presenting cells (APCs) link the innate and adaptive immune systems; the most efficient APC in the dendritic cell (DC). Precursors in the bone marrow give rise to immature DCs, which circulate throughout the body, or are resident in tissues, and act as immunologic sensors for foreign antigen. These immature DCs express specific Pattern-Recognition Receptors (PRRs) that are capable of recognizing pathogenic epitopes.
The most well-studied group of PRRs are the toll-like receptors (TLRs), which identify and bind to evolutionarily conserved patterns from microbes, including viruses. For example, TLR9 is expressed on the nuclear membrane of APCs and binds unmethylated CpG motifs in DNA, which are often derived from viral infection. TLRs are also found on NK cells, which are important effectors of the innate immune system that can help inhibit viral replication. Once a TLR-bearing immature DC is activated by an immune danger signal, such as mediators released after viral or bacterial infection, it migrates to lymphoid tissue as it matures and activates effectors of the adaptive immune system, including T cells and B cells.
Efficient antigen presentation requires high expression of MHC class I and II molecules. Stimulation of T lymphocytes by APCs also requires surface expression of costimulatory molecules, such as CD80 and CD86, and ligation of cognate receptors (eg, CD28) on the lymphocyte side of the immune synapse. Activated DCs up-regulate expression of MHC and costimulatory molecules and also secrete immunostimulatory cytokines, such as interleukin (IL)-12. Antigen presentation without concomitant costimulation can actually result in immune anergy.
The expression of immunostimulatory cytokines in the immune environment is critical for activating the relevant cellular entities, and many immunotherapeutic and oncolytic viral approaches drive cytokine overexpression, creating a more stimulatory milieu to shift the immune system toward an antitumor immune response. Although many cytokines and chemokines are involved, some of the most prominent with respect to the response to oncolytic viruses include granulocyte macrophage colony-stimulating factor (GM-CSF), IL-1, IL-2, IL-4, IL-12, IL-17, IL-18, interferon (IFN)-γ, and FMS-like tyrosine kinase 3 ligand (FLT3L).
IFN-γ, FLT3L, and GM-CSF are important mediators for improving tumor cell antigen presentation and DC function. IL-2 and IL-4 activate T cells, whereas IL-12 and IL-18 shift the T-cell response to a type 1 helper T cell (T H 1) rather than a type 2 helper T cell (T H 2) response. Although the array of cytokines involved in the immune response can be complex, this is one of the primary mechanisms through which oncolytic viral therapy and immunotherapy are intertwined. Genetically modifying oncolytic viruses to carry genes for immune-modulating cytokines may enhance stimulation of antitumor immunity. Viral infection itself results in the cellular elaboration of cytokines, some of which are involved in DC maturation and activation.
Although immunostimulatory activity may be productive for strengthening antitumor immunity, the normal immune response to viruses can significantly inhibit viral spread and subsequent tumor cell lysis. Wild-type HSV, for example, activates strong innate and then adaptive antiviral immune responses. Players in the innate immune system, including complement, NK cells, and type 1 IFNs, are activated early in the infection. The complement cascade activates the immune system against oncolytic viruses; complement depletion, in fact, allows better oncolytic viral replication in animal hosts.
Type 1 IFNs play an important role in activating innate immune responses. Although all cell types can express type I IFNs, plasmacytoid DCs, also activated through toll-like receptors, are the heaviest producers of type 1 IFNs. Oncolytic viruses, including HSV-1 and vesicular stomatitis virus (VSV), clearly induce strong type 1 IFN responses. NK cells, stimulated by the postinfectious inflammatory cytokine environment, bind to up-regulated receptors on infected cells and kill them.
Shortly after this innate response to oncolytic viral infection, the adaptive immune system is activated and the developing T H 1 immune response causes activation of CD4+ T lymphocytes and release of IFN-γ and IL-12. T H 1 responses are more likely effective against tumors themselves, although usually the response to viral infection is skewed toward T H 2, with expression of IL-4 and IL-10.
In summary, viral infection causes a strong innate immune response, largely characterized by a type I IFN response, followed by adaptive (T H 1 and T H 2) antiviral responses, which are associated with cell-mediated humoral immunity, and then the virus may be eradicated or driven into latency ( Fig. 1 ).
Immune compartments, brain tumors, and viruses
Recently, understanding of cancer immunology has advanced dramatically. A model illustrating the dynamic and complex relationship between tumor cells and the immune system is that of cancer immunoediting. In this framework, newly transformed cancer cells are initially subject to immunosurveillance, which may result in their elimination. In response, developing tumor cells may acquire mutations that allow them to avoid being fully eliminated, but they remain quiescent, existing in a tenuous equilibrium state, because the immune response prevents uncontrolled proliferation and spread.
Eventually, this balance is tipped in favor of the malignant cells, because they secrete cytokines or cause proliferation of cellular subsets that result in immunosuppression and ultimately immune escape. For instance, mechanisms of escape may include secretion of soluble natural killer (NK) cell ligands, recruitment and activation of regulatory T cells, and release of immunosuppressive cytokines.
Human immunity can be broadly divided into innate and adaptive arms. Innate immunity is antigen-nonspecific, provides a crucial barrier against many foreign antigens, and stimulates the development of adaptive immunity. Adaptive immunity is specific to certain antigens and includes direct cell killing through specifically activated cytotoxic lymphocytes, and activation of lymphocytes that generate specific antibody responses. Antigen-presenting cells (APCs) link the innate and adaptive immune systems; the most efficient APC in the dendritic cell (DC). Precursors in the bone marrow give rise to immature DCs, which circulate throughout the body, or are resident in tissues, and act as immunologic sensors for foreign antigen. These immature DCs express specific Pattern-Recognition Receptors (PRRs) that are capable of recognizing pathogenic epitopes.
The most well-studied group of PRRs are the toll-like receptors (TLRs), which identify and bind to evolutionarily conserved patterns from microbes, including viruses. For example, TLR9 is expressed on the nuclear membrane of APCs and binds unmethylated CpG motifs in DNA, which are often derived from viral infection. TLRs are also found on NK cells, which are important effectors of the innate immune system that can help inhibit viral replication. Once a TLR-bearing immature DC is activated by an immune danger signal, such as mediators released after viral or bacterial infection, it migrates to lymphoid tissue as it matures and activates effectors of the adaptive immune system, including T cells and B cells.
Efficient antigen presentation requires high expression of MHC class I and II molecules. Stimulation of T lymphocytes by APCs also requires surface expression of costimulatory molecules, such as CD80 and CD86, and ligation of cognate receptors (eg, CD28) on the lymphocyte side of the immune synapse. Activated DCs up-regulate expression of MHC and costimulatory molecules and also secrete immunostimulatory cytokines, such as interleukin (IL)-12. Antigen presentation without concomitant costimulation can actually result in immune anergy.
The expression of immunostimulatory cytokines in the immune environment is critical for activating the relevant cellular entities, and many immunotherapeutic and oncolytic viral approaches drive cytokine overexpression, creating a more stimulatory milieu to shift the immune system toward an antitumor immune response. Although many cytokines and chemokines are involved, some of the most prominent with respect to the response to oncolytic viruses include granulocyte macrophage colony-stimulating factor (GM-CSF), IL-1, IL-2, IL-4, IL-12, IL-17, IL-18, interferon (IFN)-γ, and FMS-like tyrosine kinase 3 ligand (FLT3L).
IFN-γ, FLT3L, and GM-CSF are important mediators for improving tumor cell antigen presentation and DC function. IL-2 and IL-4 activate T cells, whereas IL-12 and IL-18 shift the T-cell response to a type 1 helper T cell (T H 1) rather than a type 2 helper T cell (T H 2) response. Although the array of cytokines involved in the immune response can be complex, this is one of the primary mechanisms through which oncolytic viral therapy and immunotherapy are intertwined. Genetically modifying oncolytic viruses to carry genes for immune-modulating cytokines may enhance stimulation of antitumor immunity. Viral infection itself results in the cellular elaboration of cytokines, some of which are involved in DC maturation and activation.
Although immunostimulatory activity may be productive for strengthening antitumor immunity, the normal immune response to viruses can significantly inhibit viral spread and subsequent tumor cell lysis. Wild-type HSV, for example, activates strong innate and then adaptive antiviral immune responses. Players in the innate immune system, including complement, NK cells, and type 1 IFNs, are activated early in the infection. The complement cascade activates the immune system against oncolytic viruses; complement depletion, in fact, allows better oncolytic viral replication in animal hosts.
Type 1 IFNs play an important role in activating innate immune responses. Although all cell types can express type I IFNs, plasmacytoid DCs, also activated through toll-like receptors, are the heaviest producers of type 1 IFNs. Oncolytic viruses, including HSV-1 and vesicular stomatitis virus (VSV), clearly induce strong type 1 IFN responses. NK cells, stimulated by the postinfectious inflammatory cytokine environment, bind to up-regulated receptors on infected cells and kill them.
Shortly after this innate response to oncolytic viral infection, the adaptive immune system is activated and the developing T H 1 immune response causes activation of CD4+ T lymphocytes and release of IFN-γ and IL-12. T H 1 responses are more likely effective against tumors themselves, although usually the response to viral infection is skewed toward T H 2, with expression of IL-4 and IL-10.
In summary, viral infection causes a strong innate immune response, largely characterized by a type I IFN response, followed by adaptive (T H 1 and T H 2) antiviral responses, which are associated with cell-mediated humoral immunity, and then the virus may be eradicated or driven into latency ( Fig. 1 ).
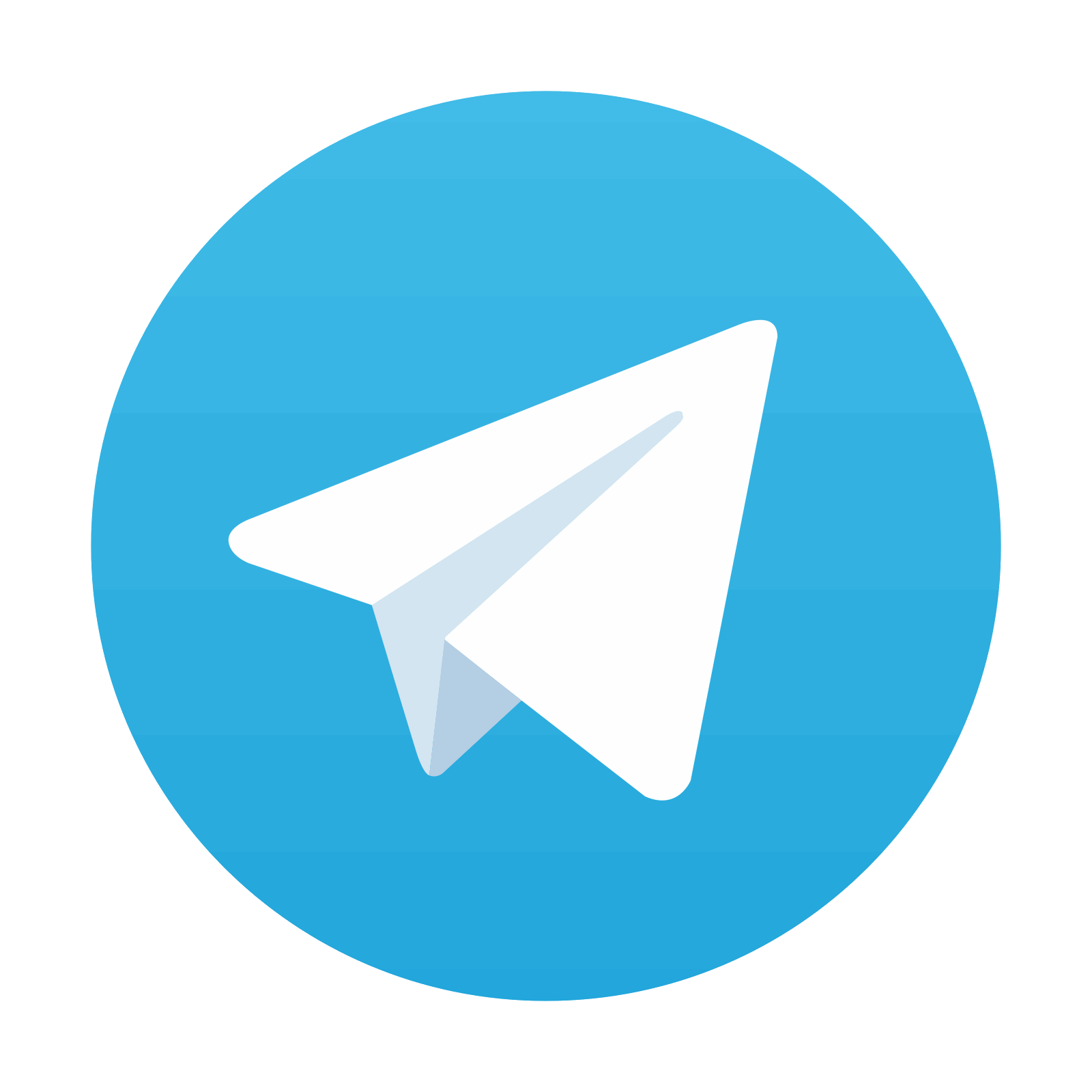
Stay updated, free articles. Join our Telegram channel

Full access? Get Clinical Tree
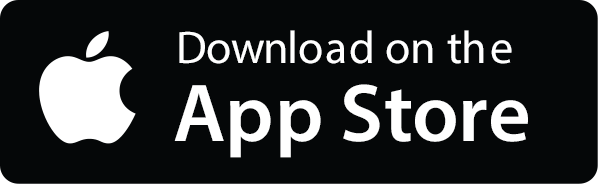
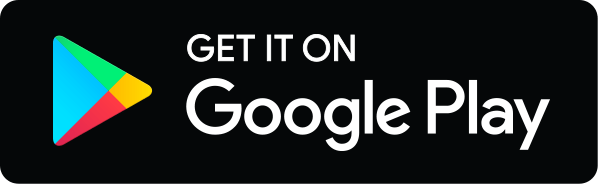