Time consuming
Modest effect (e.g., initially not appreciated by stroke survivors)
Resource and therapy intensive
Limited compliance/adherence
Transportation to a rehabilitation facility
Availability
Coverage vs. out-of-pocket costs
What Is Virtual Reality?
Virtual reality (VR) is a computer-based technology that allows users to interact with a multisensory simulated environment and receive “real-time” feedback on performance.
Most current VR systems are primarily visual experiences, using either a computer screen or special stereoscopic 3D screens (e.g., virtual reality room). Additional sound information is provided through speakers or headphones. Some advanced VR systems include haptic feedback technology that takes the advantage of the sense of touch by applying forces, vibrations, or motions to the user providing tactile information [9–12].
VR has been used in a wide variety of applications, including aeronautic and military training, architecture, sports, entertainment, art, and medicine. In the medical field, VR is being applied in rehabilitation, laser eye surgery, training for performing medical procedures (e.g., endoscopic or endovascular), and behavioral education (cognitive behavioral therapy) [9, 10, 13, 14].
VR applies relevant concepts in stroke rehabilitation, such as high repetition, high intensity, and task-oriented training of the paretic extremity [7, 15]. VR applications range from non-immersive to fully immersive depending on the degree to which the user is isolated from the physical surroundings when interacting with the virtual environment [15]. Also classified as VR are a variety of non-immersive video-game systems developed by the entertainment industry for home use, making this technology less costly and more accessible to clinicians and individuals. Several of these games have been adopted by clinicians as rehabilitation interventions even though they have not been especially designed to meet rehabilitation goals, nor validated in large randomized clinical trials.
Neurobiological Principles in Virtual Reality Applied to Stroke Rehabilitation
Recovery depends on learning new strategies and motor patterns. Three important principles may be involved in motor recovery using virtual reality technologies: (1) brain plasticity, (2) “mirror-neuron system,” and (3) the “brain reward system.”
The presence of a hemiparetic limb may result in suppression of the cortical representation of the affected limb (i.e., hand) and further inhibit its spontaneous use [16]. Motor improvement can be achieved by the brain’s own ability to relearn and readjust. Repetitive, intensive, and task-specific functional training are the current paradigms applied in neurorehabilitation after stroke to facilitate motor relearning and consequently improvement of function [17, 18]. This is possible due to cortical reorganization and rewiring in the injured brain (brain plasticity) [16, 18]. Intense training and the observation, practice, and representation on the screen of task-specific activities can facilitate cortical reorganization. This is possible by engaging the “mirror neuron system” and/or long-term potentiation effects. The use of virtual reality showed practice-dependent enhancement of the affected arm through the facilitation of cortical reorganization using functional MRI [19]. These observations were also consistent with previous studies using transcranial magnetic stimulation and functional MRI that have demonstrated decreased ipsilateral cortical activation and increased contralateral activation as functions of intensive practice of the affected limb [20, 21]. A learning by imitation has been suggested to induce an imitation-dependent organization around the motor cortex through “mirror” neural networks [22]. Similarly, participants who received sensory feedback during the VR training learned the motor pattern by imitation. This might have facilitated use-dependent cortical plasticity, which was primarily reorganized at the supplementary motor cortex [19].
For example, a study including 154 healthy teenagers revealed morphological changes (e.g., thickening of grey matter) and activation of the ventral striatum using functional MRI among frequent (defined as more than 9 h per week) video-game players. The association of video-game playing with higher left ventral striatum volume could reflect altered reward processing and represent adaptive neural plasticity [23].
VR gaming technology has all the advantages of virtual reality systems including the provision of multisensorial (visual, auditory, and tactile) feedback, affordability, and easy implementation. Moreover, VR may engage the “brain reward system,” thus improving performance by optimizing or increasing patients’ motivation.
The brain reward system is a group of dopamine-mediated mesolimbic structures (nucleus accumbens and orbitofrontal circuits) that may be activated when participants play a video game. Some studies suggest an improvement of patients’ attitudes toward chemotherapy in healthy individuals immediately after the interactive gameplay. Using functional MRI, researchers found increased activation in several brain regions including a subregion of the left parahippocampal cortex. Another study including 18 health males found two different stimuli for the reward circuits: (1) reward-specific and (2) reward nonspecific networks. The anterolateral orbitofrontal cortex processes monetary gains, whereas the posterior lateral processes more basic erotic stimuli. Further studies may be needed to validate these findings [24].
The Current Evidence
Two large meta-analyses evaluating the use of virtual reality revealed the potential benefits of VR [25, 26]. Saposnik et al. evaluated the benefits of VR in the upper extremity after stroke. They initially found 35 studies, but only 12 studies (five RCTs and seven observational) met the inclusion/exclusion criteria comprising 195 participants (on average, less than 20 patients per study). Age ranged from 26 to 88 years old. Two-thirds (n = 8) of the interventions used non-immersive VR systems (Virtual teacher, Cyberglobe, VR Motion, Pneumoglobe, Wii). Eleven of 12 studies showed a significant benefit toward VR for the selected outcomes (Table 22.2). Among the RCTs, there were three studies using immersive VR (e.g., Glasstrom, IREX, Playstation EyeMotion) and two applying non-immersive systems (e.g., VR Motion, Wii). In the pooled analysis of all five RCTs, patients randomized to VR were five times more likely to improve motor function (OR 4.89; 95 % CI 1.31–18.3) (Fig. 22.1). Interventions were delivered within 4–6 weeks with an average of 1-h duration for each session (range 30 min–2.5 h/session) in most of the studies [25].

Table 22.2
Systematic review on virtual reality in neurorehabilitation of the upper extremity after stroke
Author | Design | N | Time since stroke onset | Type VR | Intervention | Outcome | Conclusion |
---|---|---|---|---|---|---|---|
Holden et al. (2002) | Pre-Post | 9 | >6 months | Non-immersive (virtual teacher) | 1 h/day, 3 days a week × 20–30 sessions | FM, WMFT | Significant improvement in FM and WMFT |
Boian et al. (2002) | Pre-Post | 4 | 1–4 years | Non-immersive (CyberGlove and a Rutgers Master) | 2 h/day, 5 days a week, 3 weeks | JTHF | Significant improvement in computerized measure of thumb range, finger speed, fractionation, and JTHF |
Piron et al. (2003) | RCT | VR = 12 | <3 months | Non-immersive (VR Motion) | 1 h/day, 5 days a week, 5–7 weeks | FM, FIM | Small difference between VR and conventional therapy groups in FM and FIMTM |
CR = 12 | |||||||
Piron et al. (2005) | Pre-Post | 50 | >6 months | Non-immersive (VR Motion) | 1 h/day, 5 days a week, 4 weeks | FM, FIM | Significant improvement in FM and FIMTM |
Jang et al. (2005) | RCT | VR = 5 | >6 months | Immersive (IREX) | 1 h/day, 5 days a week, 4 weeks | FM, BBT, MFT | Significant difference between VR and no therapy groups in FM, BBT, and MFT |
Control = 5 | |||||||
Merians et al. (2006) | Pre-Post | 8 | 1–4 years | Non-immersive (CyberGlove and a Rutgers Master) | 2–2.5 h/day, 13 days, 3 weeks | JTHF | Significant improvement in computerized measure of thumb range, finger speed, fractionation, and JTHF |
Broeren et al. (2007) | Pre-Post | 5 | >6 months | Immersive (Reaching APE and Crystal Eyes) | 45 min/day, 3 days a week, 5 weeks | Kinematics, BBT, AMPS | Significant improvement in motor performance. No difference in BBT and AMPS |
Fischer et al. (2007) | RCT | CO = 5 | 1–38 years | Immersive (Glastrom/CAVE) | 60 min, 18 sessions, 6 weeks | WMFT, FM, BBT | Significant improvement in WMFT. Trend favoring PO in FM and BBT |
PO = 5 | |||||||
Control = 5 | |||||||
Yavuzer et al. (2008) | RCT | VR = 10 | <12 months | Immersive (Playstation EyeToy) | 30 min/day, 5 days a week, 4 weeks | Brunnstrom, FIM | Significant difference in motor performance and DIM |
Sham VR = 10 | |||||||
Kamper et al. (2010) | Pre-Post | VR + device = 7 | >6 months | Non-immersive (PneuGlove) | 60 min × 3 days a week, 6 weeks | FM, BBT | Significant improvement in FM and BBT |
VR control = 7 | |||||||
Yong et al. (2010) | Pre-Post | 16 | <3 months | Non-immersive (Nintendo Wii) | 30 min × 6 sessions within 4 weeks | FM, MFT, MAS | Feasible. Significant difference in FM and MFT |
Saposnik et al. (2010) | RCT | VR + CR = 10 | <3 months | Non-immersive (Nintendo Wii) | 60 min × 8 sessions within 2 weeks | WMFT, BBT, SIS | Feasible, safe, and significant improvement in WMFT, BBT |
RA + CR = 10 | |||||||
Kwon et al. (2012) | RCT | VR + CR = 13 | <3 months | Non-immersive (IREX) | 30 min × 5 days per week within 4 weeks | FM, MFT | Feasible, safe, and significant improvement in FM in VR and CR |
CR + CR = 13 | |||||||
Subramanian et al. (2013) | RCT | VE = 16 | 6–60 months | Immersive (CAREN) | 12 sessions over 4 weeks | FM, RPS, WMFT | VE training led to motor improvement compared to PE |
PE = 16 |

Fig. 22.1
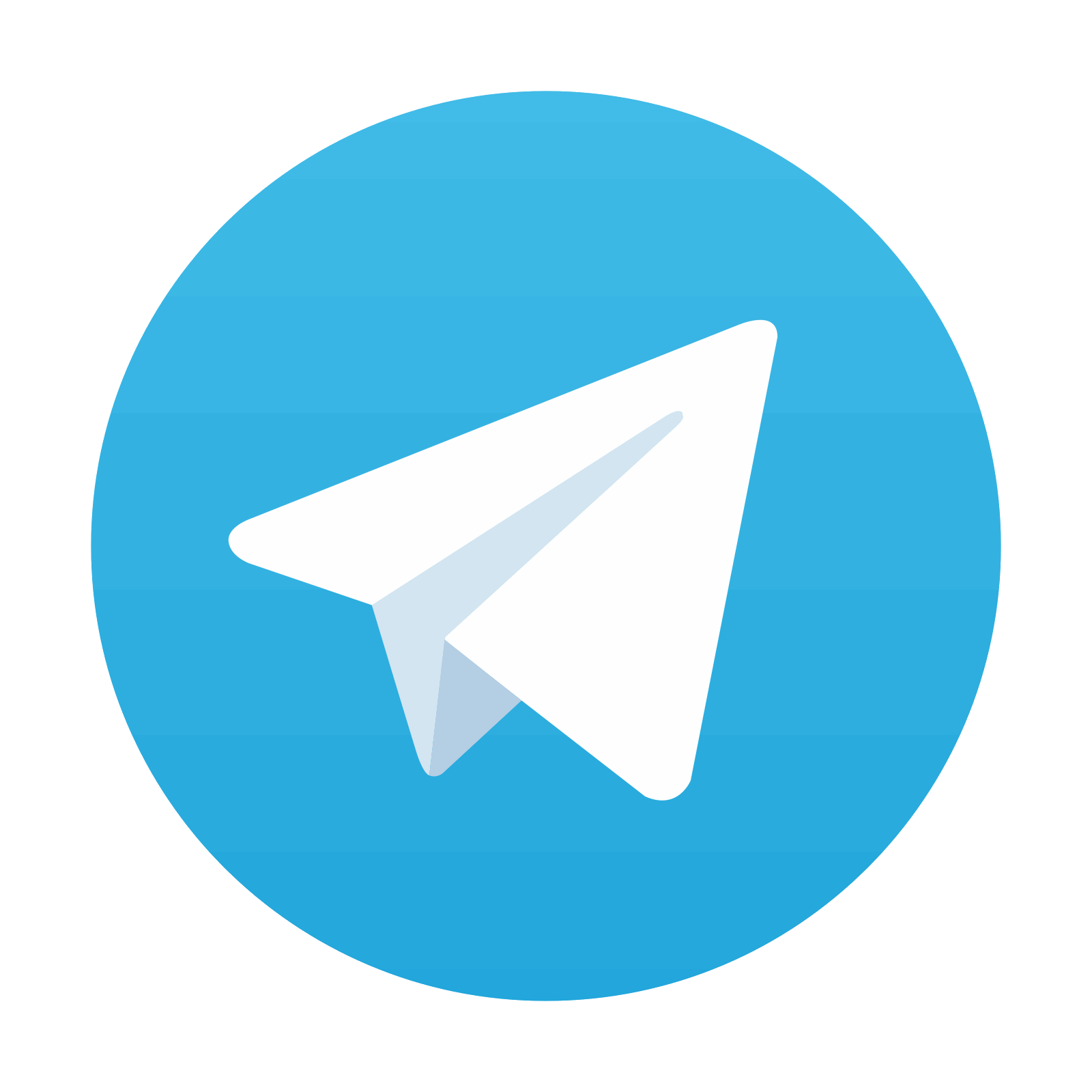
(a) Benefits of VR in RCTs (with permission from Saposnik et al.). Stroke patients randomized to VR were nearly five times more likely to achieve a motor improvement compared to controls (a). (b) Benefits of VR in observational studies (with permission from Saposnik et al.). In observational studies (b), patients receiving VR had a mean 20 % improvement compared to their baseline performance [25]. Wolters Kluwer Health, Stroke, Virtual Reality in Stroke Rehabilitation: A Meta–Analysis and Implications for Clinicians, Gustavo Saposnik, Mindy Levin, for the Stroke Outcome Research Canada (SORCan) Working Group, May 1, 2011, Volume 42, Issue 5
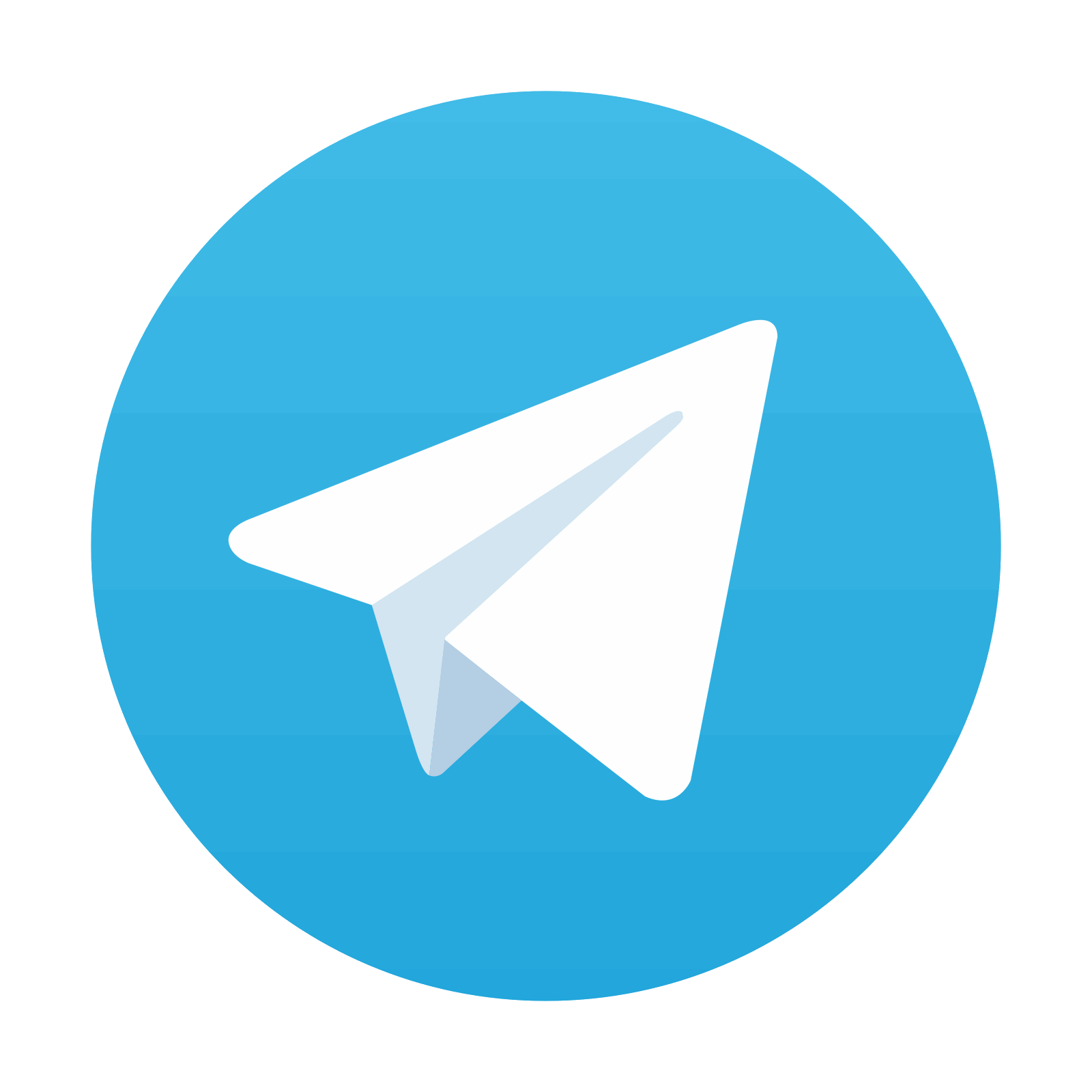
Stay updated, free articles. Join our Telegram channel

Full access? Get Clinical Tree
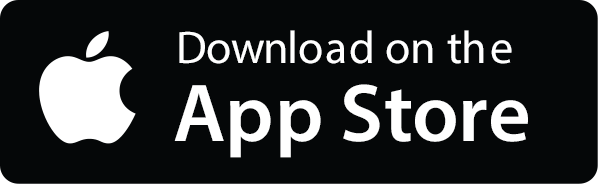
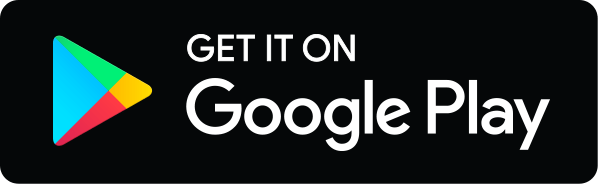
