and Gordon N. Dutton2
(1)
Department of Psychology, LMU Munich, München, Germany
(2)
Department of Visual Science, Glasgow Caledonian University, Glasgow, UK
The online version of the original chapter can be found under 10.1007/978-3-7091-1815-3_10
An erratum to this chapter can be found at http://dx.doi.org/10.1007/978-3-7091-1815-3_10
4.1 Preliminary Remarks
Disorders of brain function can be distinguished in relation to the time of occurrence of the pathological event in brain development, prenatal (before birth), perinatal (around birth) and postnatal (after birth). Such disorders may manifest as missing, incomplete or delayed development of the function(s) affected. Practically, all functional disorders that arise during the pre-, peri- and postnatal periods, irrespective of the underlying aetiology, can be subsumed under the term ‘early developmental disorder’. For labelling of the pathological event, the global term ‘brain injury’ is used to subsume the wide range of conditions including cerebrovascular, hypoxic and traumatic events that lead to disorder and/or dysfunction of cortical and subcortical structures of the visual system, including the white matter that contains the inter- and intracortical fibre connections, being affected.
Early developmental disorders can affect only one functional system, for example, the visual system, but can also have consequences for other functional systems. For example, in the case of hypoxic or traumatic injury, much of the brain is affected; in contrast, in cases of more ‘local’ brain injury, the function subserved by this structure or region is impaired. However, secondary effects of brain injury have always to be considered, because the functional outcome of brain injury is not just limited to the functions or functional systems that belong to the injured brain structures, but also to linked functions or functional systems. In the extreme case of multi-system disorders, overall functional development is either limited or delayed.
As the child grows and develops, impaired visual functions due to the central visual system being affected result in a range of behavioural outcomes. Affected functions may be absent or deficient, leading to failure to respond to what cannot be seen, as well as a range of typical adaptive strategies, and under certain circumstances, typical reactive behaviours. Learning through vision tends to be impaired to a degree related to the limitations in accessing information and social cues, and in mobility dictated by each child’s specific set of visual constraints, set in the context of the efficacy of the strategies put in place to avert these limitations.
Acquired disorders of brain function can occur in later childhood. The outcome of such loss of function, when it affects vision, differs from that due to early developmental disorder. Prior learning means that skills and memories acquired through the now lost functions can persist, and these may be identifiable and employed for rehabilitation.
Children with cerebral visual disorders and additional functional impairments present a special challenge for diagnostics and treatment, particularly in the various domains of cognition. Associated impairments can render objective reproducible assessment of the visual disorders and their diagnostic classification very difficult, particularly in the context of the more complex visual disorders (see Chap. 6). About 60 % of children with cerebral visual disorders also exhibit cognitive and (fine) motor impairments (Spreen et al. 1995; Dutton and Jacobson 2001). Difficulties with attention, learning, memory and motivation (curiosity) are among the most frequent associated disorders. The close interactions between vision, cognition and motivation mean that impairments in these domains can exaggerate the apparent degree of visual impairment or may secondarily affect any normally developed visual capacities. Lack of visual attention can manifest and be diagnosed as a visual disorder. For example, even detection and localisation and discrimination of simple visual stimuli require a critical minimum degree of attention (Brown 1990). A child with severely reduced attention will have great difficulties with gaining detailed visual experiences needed for detection, localisation, discrimination, identification, and storage of visual stimuli. Thus, a missing or delayed orienting response to a visual stimulus may indicate a severe degree of visual impairment, but may also result from pathologically reduced attention (Weiss et al. 2001), or both.
In this chapter, the concept of CVI is presented and developed, and the differences between cerebral visual disorders arising in (early) childhood and in adulthood are discussed. This section is followed by a more detailed presentation of the various visual disturbances manifesting in early development.
4.2 The Concept of CVI (Cerebral Visual Impairment)
Visual disorders as a sequel to injury to the central, i.e. post-chiasmatic visual system in adults are labelled cerebral visual disorders, but this is an umbrella term that does not indicate which visual functions or capacities are affected, and which are not (Zihl 2011, 2014). In most cases of cerebral visual disorders, the peripheral (pre-chiasmatic) part of the visual system is unaffected, but the optic nerves can be affected by presumed transsynaptic degeneration (Jacobson et al. 2003), or there may be associated pathology such as retinopathy of prematurity (Dutton 2013). The combination of peripheral (injury to the eye or optic nerve) and central visual disorder (posterior cerebral artery infarction) in traumatic injury is another example. For the diagnostic categorisation of visual disorders caused by post-chiasmatic injury in children the term ‘cerebral visual impairment’ (CVI) has been introduced. This term has, however, been criticised by several authors (e.g. Frebel 2006; Colenbrander 2009, 2010; Lueck 2010) for various reasons:
1.
The purely anatomical reference is biased, because the term ‘cerebral’ does not refer exclusively to the central visual system; in addition children with CVI can also suffer from peripheral visual dysfunction, which can affect visual information processing;
2.
The term CVI lacks diagnostic specificity and does not describe the visual functions/capacities that are affected and those that are spared;
3.
CVI does not specify whether the visual disorder is of primary or of secondary origin;
4.
CVI does not differentiate between ‘lower’ (for example, visual field, visual acuity, spatial contrast sensitivity) and ‘higher’ visual disorders, which also involve cognitive components (for example, visual spatial perception, visual recognition);
5.
CVI does not include information concerning the possible functional consequences in terms of individual visual disability and handicap.
Of course, these arguments warrant serious consideration when using the term CVI. On the other hand, the similar term ‘cerebral visual disorders’, which is used for adults, is only used to discriminate central from peripheral visual disorders, and the same criticisms may hold true. Given the fact that before the introduction of the term CVI no diagnostic label existed to denote the full range of cerebral/cortical visual disorders in children, the term CVI may be useful to mark visual dysfunction due to brain injury of whatever aetiology. Of course, a better diagnostic definition of the critical issues mentioned above is not only useful but also crucial for a valid and reliable diagnostic classification, and the selection and application of habilitation/rehabilitation and treatment measures. The main difficulty may rest with the term ‘impairment’. According to the WHO International Classification of Impairment, Disabilities and Handicaps (ICF), impairment is defined as ‘any loss or abnormality in an anatomical structure or a physiological or psychological function’. Thus, visual impairment is defined as a limitation of functions and capacities of the peripheral and central components of the visual system, which subserve visually guided actions. According to the WHO, visual impairment considers visual acuity and visual field only, which is definitely too restrictive in the context of CVI. However, if experts in this field use the term CVI as a diagnostic category that denotes dysfunction of the post-chiasmatic visual system, then the term CVI can be used as in adults with cerebral visual disorders, because the same diagnostic criteria apply. Therefore, we would like to propose the use of the term CVI by specifying its significance in the following ways:
1.
When referring to cerebral visual impairment, it should be accepted that the term ‘cerebral’ refers to the post-chiasmatic structures of the visual system. In this sense, the term ‘cerebral’ is more valid than the term ‘cortical’, because all aetiologies of posterior brain injury affect both the cortex and the underlying white matter. Thus the term ‘cortical’ has been considered too restrictive (Frebel 2006; Colenbrander 2010; Lueck 2010). Furthermore, if a child also suffers from peripheral visual dysfunction, the appropriate diagnosis (e.g. cataract or optic nerve hypoplasia) and the potentially resulting visual impairment (e.g. reduced visual acuity or contrast sensitivity; visual field disorders; colour vision deficiencies) should be reported in addition (Lerner et al. 2006). The same diagnostic procedure is used in adults with combined pre- and post-chiasmatic visual dysfunction.
2.
There should be agreement that a complete report on CVI includes both affected and spared visual functions as a standard. However, this supposes that all visual functions and capacities are assessed. Assessment of visual acuity and visual field is definitely insufficient, because visual acuity measures do not always reflect the degree of visual disability (Colenbrander 2009; Lim et al. 2005). Meanwhile, recommendations exist concerning standardised visual assessment in children with CVI (see Tables 4.1 and 6.4).
Table 4.1
Visual disorders in children with CVI
Function/capacity | Disorders |
---|---|
Visual functions/capacities | |
Visual field | Homonymous field disorders (hemianopia); bilateral lower quadrantanopia; unilateral upper or lower quadrantanopia |
Visual acuity | Often reduced; may also be normal |
Spatial contrast sensitivity | Often reduced; may also be normal |
Colour vision | May be impaired, but often normal |
Space perception | Position and distance perception may be impaired |
Depth perception | May be impaired; visual navigation may be impaired |
Movement vision | Detection of moving stimuli may be impaired |
Visual identification | Objects, faces and facial expressions, places and routes may only partly or not at all, be correctly discriminated and/or identified (e.g. misidentification due to common features) |
Overview | May be restricted (only local processing; see also Attention) |
Figure-Ground-Discrimination | Often impaired |
Visual search | Often impaired |
Visual guidance of movement | Can affect upper limbs and/or lower limbs |
Oculomotor functions | |
Accommodation | Often impaired |
Vergence | Strabismus |
Nystagmus/tonic deviation | May be present |
Fixation | Unstable; spontaneous nystagmus |
Saccadic eye movements | Inaccurate (dysmetria: hypo-/hypermetria) |
Pursuit eye movements | Inaccurate (jerky) |
Visual search/exploration | Reduced (constricted) and unsystematic, exacerbated by fatigue |
Other functions | |
Attention | Field of attention is often restricted → reduced overview in one or both hemifields; global reduction of attention focused and divided attention are impaired (neglecting of stimuli) |
Visual memory | Stimulus positions, places and paths are inaccurately stored in working and long-term memory |
Objects and faces (and proper features) are inaccurately stored in working and long-term memory | |
Visual curiosity | Reduced motivation for visual stimuli and visual cognition |
Grasping, pointing | Often inaccurate when visually guided (see space perception) |
3.
The specification as to whether a particular visual dysfunction is of primary or secondary origin is an important diagnostic demand. Hence it follows that for a valid and complete assessment of CVI, cognitive functioning has also to be assessed in regard to visual perception, and one should also consider interactions between vision and cognition (as well as motivation and emotion) (Dutton 2002, 2009).
4.
Detailed diagnoses include refractive disorders and ‘lower’ as well as ‘higher’ visual disorders; however, because there is no accepted definition of ‘lower’ and ‘higher’ functions, it may be difficult to assign visual functions/capacities exclusively to one or other category. The best compromise seems therefore to screen then assess as appropriate, salient visual functions and capacities. In particular in the case of ‘higher’ visual disorders, it is important to differentiate between primary and secondary causation, i.e. visual recognition difficulties also arise from impairment in ‘lower’ visual functions.
5.
Consideration of the possible functional consequences of individual visual disability and handicap is an important diagnostic issue, and it is essential to determine which visual capacity management measures are required. However, in the absence of research reports on functional consequences and their amelioration, this can sometimes be a difficult task, and only intuitive or ‘common sense’ provisions may be possible.
In conclusion, for a valid and reliable use of the term CVI as a diagnostic category a standard is needed, which not only defines which assessment tools should be used and how, but also takes into consideration (oculo-) motor and cognitive functions (see Table 4.1). CVI is still not defined and used consistently (Boot et al. 2010). It would, however, represent an important step forward to use the term CVI in a standardised form in all disciplines involved in the diagnostics, treatment and advice concerning CVI. We therefore adopt the recommendation raised by Boot et al. (2010) for a definition of CVI that ‘is based on functional visual processing rather than anatomical landmarks’ (p. 1149). In this book, we use the term CVI in this sense to denote cerebral visual disorders, which are then further specified.
Visual disturbances in CVI can vary markedly in degree. (1) The most profoundly affected children may show little evidence of useful functional vision apart from reflex ‘blindsight’ in some cases, which may only be intermittent in nature (Boyle et al. 2005). (2) In other cases there may be associated cerebral palsy and intellectual and attentional disorders related to extensive brain involvement. Indeed new classifications of cerebral palsy include CVI as a potentially integral element (Bax et al. 2007; Rosenbaum et al. 2007). (3) At the other end of the spectrum, perceptual visual dysfunction with normal or near normal visual acuities and abilities commensurate with mainstream education has also been described in relation to premature birth (Macintyre-Béon et al. 2013). Such ‘minor’ degrees of perceptual visual dysfunction may not be uncommon but may be going unrecognised (Williams et al. 2011).
While the manifestations of CVI can be seen as a spectrum, ranging from profound to mild, the pragmatic differentiation of affected children into the above three groups is arguably warranted because they tend to be managed and taught by different professionals in different environments, who may thus be unaware of the features and needs of children in the other two categories. Yet the lessons learned from children in one category can be conceptually transferred, to benefit children in the other two groups.
CVI in children can differ from patterns seen in adult patients with cerebral visual disorders. Knowledge of how the human higher visual system functions when damaged has been informed by the extensive literature describing the specific visual dysfunctions that occur following focal disorder of the adult visual brain, supported by experimental work studying the visual outcome in affected subjects. In children however, pathology affecting the developing visual brain leads to similar but significantly different features because:
Early onset damage to the brain is frequently diffuse and affects a range of interacting and developing brain functions.
Brain growth, neuroplasticity and development, following the injury, lead to variable degrees of recovery.
Compensatory behavioural adaptations made by the developing child may mask the underlying deficit, unless that specific function is stressed, either by tiredness or by a scenario that isolates the disordered function.
Reactive behaviours, for example, distress in crowded environments, are common.
Habilitational strategies may facilitate and thus enhance both functional and behavioural adaptations.
Young children have not knowingly experienced any other type of vision and so ‘know’ their vision to be ‘normal’. They are therefore ‘asymptomatic’ and cannot describe their visual difficulties, as they are not initially aware of them, until they can make comparisons with their peer group. Their visual disorders need therefore to be inferred from their observed behaviours, and information needs to be sought from parents and carers by structured history taking.
Performance in tasks requiring visual search, visual attention and visual guidance of movement can diminish in the context of tiredness, distraction and stress, perhaps due to diminished functional reserve. The resulting day-to-day variation in performance may be incorrectly attributed to lack of concentration, if an adult construct is inappropriately applied.
Parents and professionals, such as teachers and psychologists may misinterpret a child’s visual performance as a ‘behavioural disorder’. This can lead to criticism, which can lower self-esteem and affect the behaviour of a child who cannot understand what is wrong, and cannot therefore ‘improve’.
Adult models of interpretation of the features of perceptual and cognitive visual dysfunction in children therefore have significant limitations, and for children, a model of interpretation founded upon observation and interpretation of visual behaviour is needed, to provide the foundation for both diagnosis and management. This subject is in its infancy. Many psychological visual testing strategies are founded upon a presumption of normal visual acuities and visual fields (which is commonly not the case in children with damage to the visual brain). They are also designed to detect aberrance from the normal range, rather than applying a diagnostic model to identify and typify specific perceptual dysfunctions that result from brain injury. Thus there is a paucity of investigations designed to characterise the wide range of perceptual and cognitive visual problems that parents commonly describe. Detailed history taking is therefore essential to reveal the nature and degree of the visual dysfunctions, which can then be corroborated, by observation of the child’s behaviour in salient contexts.
4.3 Visual Disturbances
In the first years, children are unable to report on visual difficulties or answer questions about them. Therefore, no visual ‘anamnesis’ can be recorded. However, expert/skilled history taking that characterises the visual difficulties provides typical patterns of visual behaviour, identifies disorders and skills, and determines the parents goals and ambitions for their child combined with systematic and detailed observation and analysis of visually guided behaviours, particularly at this age, allows important conclusions to be drawn concerning affected and spared visual functions and capacities (see Chap. 6). The analysis of these observations provides valuable information, which can be enhanced by systematic manipulation of stimulus and/or task conditions and determination of whether the features of a suspected visual disorder increase or decrease, respectively. This approach also provides useful insights to guide intervention, because any decrease in the impact of the visual disorder in question indicates that improvement may be possible (see Chap. 7).
In the following sections, the range of visual disorders in infancy is described. Where only few reports are available in the literature, descriptions of visual disorders are used from adults, because corresponding disorders in adulthood are of a similar nature. A significant difference exists, of course, between adults and children, in that children have not gained sufficient visual experience before the age of 2 years, and thus their mental representation of the visual world is still limited, and any injury to the central visual system during this period of life affects an incompletely developed visual system. Visual disorders depend on the site and extent of injury to the visual system. Persisting vision after brain injury is evaluated on the basis of its usefulness in everyday life activities; not all persisting capacities necessarily accord an advantage despite being demonstrated under examination conditions. Numbers and percentages, respectively, of children reported with CVI differ between studies because of different selection criteria, i.e. in groups with suspected visual dysfunction numbers are usually higher than in unselected groups.
Table 4.2 summarises typical visual disorders after unilateral or bilateral post-chiasmatic injury depending on the locus of injury. Most commonly, cortical and subcortical visual structures are affected; in this case, associations of visual dysfunction (e.g. absence of visual field, reduced visual acuity, oculomotor dysfunction) occur. For the classification and understanding of the various visual disorders it has to be recognised, that as a rule, a range of visual disorders tend to occur in association, and that they are only seldom isolated. Table 4.3 gives an overview of the frequency of visual disorders in 401 children. These data show that about 50 % of children exhibit a combination of reduced acuity, strabismus and/or nystagmus. In the individual case, this combination may make it very difficult if not impossible to disentangle estimated visual acuity values from fixation issues, especially in younger children.
Optic chiasm |
Monocular or binocular visual field defects |
Visual acuity and contrast vision may be reduced in one or both eyes |
Optic tract |
Homonymous visual field defects that tend to differ in extent and are therefore incongruous |
Visual acuity and contrast vision may be reduced in both eyes when both sides are affected |
Optic radiation, striate cortex (area 17, V1) |
Homonymous visual field defects that tend to be similar in extent and are congruous |
Visual acuity and contrast vision may be reduced and visual fields constricted when both sides affected |
Visual association cortex (prestriate cortex) |
Occipito-parietal visual areas (dorsal route; WHERE- pathway) |
Impairments in spatial perception and perception of movement |
Impairments in spatial orientation, and navigation |
Impaired spatial guidance of motor activities (saccadic and pursuit eye movements; grasping and pointing, walking over steps, and through crowds or past obstacles) |
Occipito-temporal visual areas (ventral route; WHAT- pathway) |
Impairments in contrast sensitivity, form and colour vision, and stereopsis |
Impairments in object and face perception, and route finding (identification and recognition) |
Table 4.3
Summary of visual and oculomotor disorders in 401 children with mainly pre- and perinatal brain injury (age at assessment: 1–16 years)
Homonymous visual field defects | 32.4 % |
Reduced visual acuities | 48.6 % |
Strabismus | 8.6 % |
Nystagmus | 3.9 % |
Combinations | 50.1 % |
The high variability in the frequencies of childhood cerebral visual disorders reported in the following sections may be explained by differences in inclusion/referral criteria, assessment methods, heterogeneity of aetiology, associated disorders, age at time of brain injury, age at time of testing, and group size. In Table 4.4, results of visual assessment from 82 children with CVI and complete data sets are summarised. Data were collected between 1994 and 2002 in a study carried out in cooperation with Siegfried Priglinger, former head of the Unit for developmental visual disorders and early intervention, Hospital of the Hospitaller in Linz (Austria; in the following referred to as ‘Linz study’). For comparison, data from 121 children (age: 3–180 months) with CVI from the study published by Fazzi et al. (2007) are shown. Among 186 children with visual dysfunction and complete assessment, the group with CVI represents 44 %. Nielsen et al. (2007a) found CVI in 48 out of 97 children; this corresponds to 49.5 %. The most frequent aetiologies for CVI in the Linz study was perinatal hypoxia or hypoxia in preterm children (56.3 %), followed by morphological disorders of brain development (28 %). In ~30 % the aetiology of abnormal brain development was of prenatal origin, in ~60 % of perinatal, and in ~10 % of postnatal origin. At the time of assessment, about 15 % of children were younger than 2 years; about 70 % of children were between 3 and 5 years old and about 15 % were older than 5 years. More than half of the children (55 %) showed optic nerve atrophy; about two-thirds suffered from epilepsy. The majority of children (~98 %) also suffered from global developmental disorder, mainly in the domains of cognition. In two children without associated cognitive developmental disorders, CVI was caused by bilateral occipital cysts and by an occipital tumour, respectively, the latter being removed at the age of 2 years. Visual curiosity (spontaneous exploration of the surrounding by eye movements) was reliably observed in only about 25 % of children; however, all children responded promptly and reproducibly to a peripheral light stimulus. About 90 % of children suffered from additional motor disorders, mostly cerebral paresis or delayed motor development. The combination of CVI, developmental cognitive and motor disorders is the typical constellation of features after chronic cerebral hypoxia (Dutton and Jacobson 2001). Our observations in the Linz study are in good agreement with the reported disorders in the study of Fazzi et al. (2007). Cerebral hypoxia and epilepsy were reported as being more frequent causes by Fazzi et al., while global developmental disorder, motor disorders, and atrophy of the optic nerves were less frequently reported. Table 4.5 shows the most frequent visual and oculomotor disorders in the Linz study. The proportion of children with homonymous visual field defects is in the region of 60 %. A high percentage of children (~75 %) had difficulties with discrimination of colours and with visual orientation; and the majority of children (~90 %) found it difficult to discriminate patterns, figures, and objects accurately and reliably. Strabismus (convergent and divergent) was found in about 60 % of children; a minority (<5 %) showed congenital nystagmus. However, about 60 % of children had difficulties with accurate fixation. In about half of the group (52 %) oculomotor abnormalities were associated with low visual acuity; this number is similar to other studies (Groenendaal et al. 1989; Dutton et al. 1996; Jacobson et al. 1996; Mercuri et al. 1997; Stiers et al. 1998; see also Table 4.3). Fazzi et al. (2007) found that in their group of 121 children with CVI of mostly perinatal origin only ~6 % manifested a homonymous visual field defect, but in ~87 % there was low visual acuity, and in 48 % inaccurate fixation. The relatively severe nature of the visual features described indicates that children with ostensibly perceptual visual dysfunction alone, that for example, may well be common but often undiagnosed sequel to premature birth (MacIntyre-Béon et al. 2013), and may be not infrequent in the general population (Williams et al. 2011), were probably not included in these studies.
Table 4.4
A (N = 82) | B (n = 121) | |
---|---|---|
Aetiology | ||
Hypoxia | 9 (35.4 %) | 81 (66.9 %) |
Morphological brain disorders | 23 (28.0 %) | 15 (12.3 %) |
Preterm | 17 (20.9 %) | 63 (52.1 %) |
CV | 08 (9.7 %) | |
Encephalitis | 06 (7.3 %) | |
Traumatic brain injury | 02 (2.4 %) | |
Occipital tumour (operated) | 01 (1.2 %) | 02 (1.7 %) |
Others | 23 (19.1 %) | |
Time of brain developmental disorder or brain injury | ||
Prenatal | 23 (28.0 %) | 28 (23.1 %) |
Perinatal | 50 (61.0 %) | 81 (66.9 %) |
Postnatal | 09 (11.0 %) | 12 (10.0 %) |
Additional disorders | ||
Global developmental disorder | 80 (97.6 %) | 90 (74.4 %) |
Reduced visual curiosity | 61 (74.4 %) | |
Motor disorders | 74 (90.2 %) | 88 (72.7 %) |
Tetraparesis/-plegia | 19 (25.6 %) | 42 (34.7 %) |
Hemiparesis/-plegia | 06 (08.2 %) | 46 (52.3 %) |
Developmental delay | 49 (66.2 %) | |
Optic nerve atrophy (bilateral) | 45 (54.9 %) | 17/53 (32.1 %) |
Epilepsy | 26 (31.7 %) | 55 (45.5 %) |
Time of visual assessment | ||
≤3 years | 22 (26.8 %) | |
4 years | 23 (28.0 %) | 4.5 years (SD: 3.3 years) |
5 years | 19 (23.2 %) | |
6–7 years | 18 (22.0 %) |
Table 4.5
Visual and oculomotor disorders in the Linz study (82 children with CVI; see also Table 4.4)
Homonymous visual field defects | 48 (58.5 %) |
Unilateral | 09 (18.7 %) |
Bilateral | 39 (81.3 %) |
Reduced visual acuity | 82 (100 %) |
Impaired colour vision | 63 (76.8 %) |
Impaired light adaptation (photophobia) | 18 (2.0 %) |
Impaired spatial orientation | 61 (74.4 %) |
Impaired form/object perception | 76 (92.7 %) |
Inaccurate fixation | 48 (58.5 %) |
Strabismus | 50 (61.0 %) |
Congenital nystagmus | 03 (03.6 %) |
Combination | 43 (52.4 %) |
4.3.1 Visual Field
Visual field defects are the most frequently identified visual disorders in adults with cerebral visual disorders (Zihl 2011). Vision is affected in homonymous regions of the left (after right-sided) and right hemifield (after left-sided brain injury), because of crossing of about half of the fibres in each optic nerve (arising from the nasal retina of each eye) at the optic chiasm. Typical unilateral visual field defects include loss of vision in one hemifield (hemianopia), upper or lower quadrant (quadranopia), and islands of blindness in the inner visual field (paracentral scotomata) (see Fig. 4.1, 1-4), which are identical or very similar in both eyes. Bilateral injury to the post-chiasmatic visual system can cause complete blindness in both visual fields (‘cerebral blindness’). Less dramatic consequences are bilateral homonymous hemianopia but with sparing of central visual function or ‘tunnel vision’, bilateral upper or lower quadranopia leading to bilateral upper or lower visual field impairment, sometimes also referred to as upper or lower homonymous hemianopia, and central scotoma, i.e. loss of vision in the central visual field (Fig. 4.1, 5-8). While cerebral anopia and scotoma refer to total lack of vision, cerebral homonymous amblyopia refers to the homonymous depression of light vision, with lack of colour and form vision in the affected hemifield or in both hemifields (Fig. 4.1, 9-10). Figure 4.2 shows the effect of homonymous visual field loss on global perception of a scene.



Fig. 4.1
Schematic illustration of homonymous visual field loss (binocular visual fields; spared parts are shown in white). 1–4 unilateral visual field loss, 5–8 bilateral visual field losses, 9–10 amblyopia. 1 left-sided hemianopia, 2 left upper quadranopia, 3 left lower quadranopia, 4 left-sided paracentral scotoma, 5 bilateral hemianopia (‘tunnel vision’), 6 bilateral upper quadranopia (upper hemianopia), 7 bilateral lower quadranopia (lower hemianopia), 8 central scotoma, 9 left-sided hemiamblyopia, 10 bilateral cerebral hemiamblyopia

Fig. 4.2
Effects of homonymous visual field loss on global perception of a scene. Unseen portions of the scene are indicated in white. (a, b) left- and right-sided hemianopia; (c, d) left upper and left lower quadrantanopia; (e) bilateral hemianopia with central sparing, (f) central scotoma
Homonymous visual field defects after post-chiasmatic brain injury of different aetiologies have also been reported in children (van Hof-van Duin and Mohn 1984; Flodmark et al. 1990; Ragge et al. 1991; Dutton et al. 1996; Jongmans et al. 1996; Mercuri et al. 1997; Jacobson et al. 2006; Fazzi et al. 2007). Bilateral homonymous visual field defects, in particular, bilateral hemianopia, are the most frequent type of homonymous visual field disorders in infancy, probably due to the high percentage of cases of the central visual system being affected, and being manifest at this stage of development (Table 4.6; see also Sect. 4.5). Bilateral lower visual field impairment is particularly common in children with periventricular white matter pathology (Jacobson and Dutton 2000).
Unilateral post-chiasmatic brain injury occurs less frequently in younger children (van Nieuwenhuizen and Willemse 1984), and, therefore, unilateral homonymous visual field defects are infrequent. In older children, unilateral homonymous visual field defects predominate and are as frequent as in adults (Kedar et al. 2006). Interestingly, children do not seem aware of their lack of visual field, when compensation tends to be efficient. Bajandas et al. (1975) investigated three children at the age of 10–14 years, who exhibited homonymous hemianopia because of congenital brain injury, but were never aware of their hemianopia. Possibly these children had developed compensatory strategies quite early in life and had successfully adapted to their lack of visual field. Consequently, they had no (or only mild) difficulties in everyday life activities, at least no more ‘difficulties’ than children of the same age may also have. This report is supported by the observation of one author (JZ). In the context of neurological and neuroradiological examinations of an 11-year-old girl with suspected epilepsy, a small cystic lesion was detected in the left occipital lobe. She did not report any difficulties in school, in everyday life and in sports (for example, table tennis, cycling). Quantitative perimetry revealed right-sided homonymous hemianopia, with central sparing of 4°. Recording of oculomotor scanning during the inspection of scenes showed nearly normal scanning patterns (see Fig. 4.3). It may be assumed that this efficient spontaneous oculomotor compensation was possible, because visual curiosity, spatial guidance of attention and eye movements, and executive function, were not affected by the occipital cyst. About 80 % of adults with homonymous visual field defects do not usually show spontaneous compensation, probably because additional injury to brain structures involved in the visual-spatial guidance of attention and eye movements (thalamus, dorsal information processing route) and/or the underlying white matter, leading to disconnection from one another and from the prefrontal cortex. Typical consequences include time-consuming scanning patterns of the visual environment, or even smaller scenes, and – to a lesser extent – omission of stimuli on the affected side, in the absence of visual neglect. Scanning patterns are characterised by a considerably higher number of small saccades and fixations; in cases with bilateral visual field defects the range of visual search may in addition be restricted (Zihl 2011; see also Fig. 4.3).


Fig. 4.3
Oculomotor scanning patterns during inspection of a scene in a normal 20-year-old subject (a), a 24-year-old patient with a right-sided hemianopia (sparing: 6°) after surgical removal of an occipital tumour (b 13 weeks ‘post-op’), and an 11-year-old girl with a right-sided congenital hemianopia (c sparing: 4°). Black dots: fixation positions, lines: saccadic movements. Scanning times were 17.7 s in a, 43.3 s in b, and 19.3 s in c. All patients reported all relevant items of the scene. Note the efficient oculomotor compensation in the case of congenital hemianopia in c
Acquired homonymous hemianopia in later childhood is rare, but in one author’s (GD) experience, is most commonly related to a vascular event (such as spontaneous rupture of an arterio-venous malformation). As in adults, this type of visual field loss tends to be problematic. One affected child (seen by GD), whose right-sided intra-occipital haemorrhage caused left hemianopia, discovered herself that reading was enabled by reading vertically upwards, so that the material that had been read moved into the hemianopic visual field. She disguised this behaviour by tilting her head to the right and the text to the left.
It is possible that in some cases, the development of divergent strabismus develops and becomes compensatory for the hemianopia, and care has to be taken when considering cosmetic strabismus surgery (Jacobson et al. 2012).
Dutton et al. (2004) found in children with occipito-parietal brain injury and bilateral lower quadranopia a limitation of downward gaze, indicating that children with injury to the superior radiation can exhibit a combination of homonymous lower visual field impairment and reduced spontaneous oculomotor compensation in the same way as adults.
Homonymous quadrantic and hemianopic visual field deficits potentially draw attention to associated perceptual disorders. A unilateral or bilateral lower quadrantic visual field deficit points to possible contralateral unilateral or bilateral posterior parietal dysfunction respectively, while a unilateral or bilateral upper quadrantic deficit points to possible contralateral unilateral or bilateral temporal lobe pathology (if the visual field deficit relates to damage to the optic radiations as they pass through these structures).
4.3.2 Visual Acuity, Contrast Sensitivity, Optics and Accommodation
4.3.2.1 Low Acuity and Contrast Sensitivity
After unilateral post-chiasmatic injury visual acuity in adults is not at all or only moderately impaired. After bilateral post-chiasmatic injury, visual acuity can be completely preserved, or impaired to varying degree; in the extreme case only finger counting may be possible (Zihl 2011).
Bilateral reduced visual acuity is the most frequently reported visual dysfunction in children with CVI (see Table 4.7); reported frequencies vary between 30 and 100 %. Acuity values range between normal acuity and 2.0 logMAR, i.e. visual form discrimination impairment varies between moderate and very severe, with only coarse discrimination between black and white, or light and dark, respectively (Jan et al. 1986; Dutton et al. 1996). Many children with CVI and reduced visual acuity in addition exhibit myopia, hyperopia, or astigmatism, which may also contribute to low acuity and should, of course, be corrected by optical means. Refraction anomalies have been reported by Khetpal and Donahue (2007) in 20 % of 98 children with CVI; Fazzi et al. (2007) reported such anomalies in 80 % of 121 children with CVI. In the Linz study all 82 children with CVI had reduced visual acuities; 31 children (37.8 %) also had hyperopia, 32 children (39 %) myopia, and 54 children (65.8 %) astigmatism. Of course, visual acuity values depend on test conditions; results are therefore, not always comparable. In addition, acuity values also depend on a child’s fixation accuracy and attentional capacities and compliance; an accurate and reliable measurement outcome can only be expected, if both are sufficient (see also Sects. 4.4.2 and 6.4.2).
Table 4.7
Frequency of reduced visual acuity in children with CVI reported in different studies
Study | N | Frequency (%) |
---|---|---|
Jan et al. (1986) | 236 | 82 |
Schenk-Rootlieb et al. (1994) | 49 | 73 |
Dutton et al. (1996) | 90 | 30 |
Fazzi et al. (2007) | 121 | 87 |
Linz study | 82 | 100 |
Table 4.8
Visual performance in a case (LG) with congenital visual agnosia and prosopagnosia
Stimuli | Number | Correct responses (%) |
---|---|---|
Real objects | 45 | 77.8 (100) |
Object drawings | 30 | 90.0 (100) |
Animals made of plastic | 22 | 63.6 (100) |
Single letters | 23 | 60.9 (96.7) |
Object photographs | 30 | 56.6 (100) |
Complex scenes (photographs) | 28 | 53.6 (96.4) |
Object constancy | 23 | 30.4 (100) |
Integration of parts of object | 30 | 13.3 (73.3) |
Overlapping objects | 20 | 10.0 (100) |
Familiar faces (family) | 26 | 38.5 |
Discrimination of faces | 15 | 87.7 (66.7) |
Face constancy | 78 | 30.8 (92.0) |
Discrimination of age and gender | 25 | 28.0 (92.0) |
Identification of face parts | 10 | 00 (100) |
Identification of facial expression | 21 | 02.1 |
Adults with impaired spatial contrast sensitivity (‘contrast vision’) after brain injury report ‘unclear’ or ‘foggy’ vision, stimuli appear ‘blurred’, in particular details of patterns, figures and objects, letters and faces; it often produces considerable visual discomfort. The explanation for this visual phenomenon may be reduced acuity or difficulties with accommodation and convergence (fusion capacity is reduced), or may not be explained by these factors. Contrast sensitivity may be reduced mainly for the higher spatial frequencies (Bulens et al. 1989), but can also be depressed in the entire range of spatial frequencies (Bodis-Wollner and Diamond 1976; Hess et al. 1990; Zihl 2011).
In children with CVI, contrast vision is probably as often impaired as visual acuity (Fig. 4.4). Jacobson et al. (1996) reported impaired contrast vision in 11 out of 13 children with CVI; Mercuri et al. (1997) found this visual dysfunction in 12 out of 31 children with CVI, while Stiers et al. (1998) found it in 21 of 22 children with CVI. Fazzi et al. (2007) reported impaired contrast vision in 58 (47.9 %) of 121 children. Good et al. (2012) reported reduced contrast sensitivity in 30 children and impaired grating acuity in 32 out of 34 children with CVI. It is important to note that contrast sensitivity is perceived more easily for single stimuli, and much more difficult to perceive for regular patterns of, for example, vertical stripes. Children with reduced single-stimulus contrast sensitivity may also show reduced visual acuities, while children with reduced pattern stimulus contrast sensitivities may show better acuity values for optotypes. Furthermore, it should be mentioned that peripheral dysfunction causing amblyopia may also be associated with reduced contrast sensitivity because of visual deprivation effects (Lerner et al. 2006). Central image crowding also disturbs visual processing of fine details and of reading (Martelli et al. 2009; Huurneman et al. 2012).


Fig. 4.4
Impaired spatial contrast sensitivity in a 5-year-old boy with central amblyopia and reduced visual acuity (0.9 near vision; circles), a 4-year-old boy with CVI and near visual acuity of 0.1 (triangles) and in a 5-year-old boy with CVI and near visual acuity of 0.1 (squares). Grey profile indicates range of contrast sensitivity in five normal children aged 5–6 years
4.3.2.2 Refractive Error
Refractive errors comprise short (or near) sightedness, long (or far) sightedness and astigmatism. The greater the refractive error, the greater is the degree of correctable visual impairment.
Newborn children have a wide range of refractive errors. These progressively diminish during the first few months of life, as growth and development of the eyes brings about emmetropisation. However, persistent refractive errors are common in children with cerebral palsy (many of whom also have CVI), probably due to impaired emmetropisation (Saunders et al. 2010). Initial refraction is carried out in at risk children (Das et al. 2010), having first paralysed accommodation using a cycloplegic agent such as cyclopentolate to ensure accurate refraction. In most cases of identified refractive error, the full spectacle correction is prescribed. For large refractive errors spectacles may not improve vision initially, because of superadded ammetropic amblyopia, for which full time spectacle wear leads to subsequent gradual improvement of vision over a period of weeks.
4.3.2.3 Lack of Accommodation
Lack of accommodation also compounds CVI in more than 50 % of those with cerebral palsy (Leat 1996; McClelland et al. 2006). Lack of the pupil constriction that normally takes place when looking at a near target gives a reliable indicator that accommodation may be lacking (Saunders et al. 2008). This leads to impairment of near acuity. In those who are hypermetropic, accommodation overcomes the refractive error, but when accommodation is poor or absent, both near and distance visual acuities are impaired. The presence and degree of accommodative function are determined clinically by a procedure known as dynamic retinoscopy, in which refraction is measured while the child looks at targets at different distances and the degree of resulting myopia is elicited. When accommodation is impaired, this myopia is less than expected or absent, and the requisite near spectacle correction is provided. Either varifocal or bifocal lenses are given for children who are able to elect to use down gaze to view near targets through the lower part of the spectacles, or as separate (well labelled) spectacle corrections for those who cannot.
Refractive error and accommodative dysfunction are common in children with CVI of early onset. Refraction is warranted for all cases, and optimal spectacle correction needs to be worn by those with refractive error, prior to assessment of functional and perceptual vision (Das et al. 2010).
4.3.2.4 Hypermetropia Causing Convergent Strabismus (Accommodative Esotropia)
Another indication for spectacle wear is convergent strabismus due to hypermetropia. The accommodative effort needed to bring the near scene into focus brings about the synkinesis of convergence, which is less likely to be inhibited in those whose damage to the brain has caused CVI, rendering accommodative esotropia more likely. Spectacle wear that corrects the hypermetropia eliminates the need for the increased accommodative effort. This in turn eliminates the stimulus for the eyes to converge, and immediate diminution in the angle of strabismus takes place. In many cases gradual, progressive further improvement of eye alignment is observed during the ensuing months. If surgical intervention is needed for this type of strabismus, it is deferred until the eye alignment has normalised as much as possible.
4.3.2.5 Spectacle Wear
Spectacle wear corrects the blurred distance vision of myopia, but a side effect of the required concave lenses is to reduce image size, especially when the myopia is marked. Children with additional low visual acuities due to CVI, or associated pathology of the eyes or optic nerves may choose to remove their spectacles when looking at books or when viewing near objects, because they can instead focus upon material held close to the eyes. This gives the magnification due to proximity, diminishes visual crowding, and helps compensate for the low vision.
Visual crowding due to CVI can impair reading. For children who are long sighted and in those who have impaired accommodation, positive lenses both magnify text, and allow it to be read closer to the eyes, bringing about additional magnification due to proximity. This can in turn reduce crowding of text on the printed page, which can enhance access to the printed word and story-book images. Correction of only one or two dioptres of hypermetropia can benefit some children with CVI.
Children who have CVI associated with visual crowding often choose to remove their spectacles when they are tired, presumably because the resulting blur serves to diminish the degree of image crowding. However, unlike in myopia, this does not lead to any improvement of vision.
4.3.3 Visual Adaptation
Visual light and dark adaptation can be impaired in (even moderate) retinopathy, as a sequel to dysfunction of retinal light receptors (Hansen and Fulton 2000), as a result from chiasmal compression (Kawasaki and Purvin 2002) and following injury to the post-chiasmatic visual system (Zihl 2011). Patients with impaired light adaptation, which can manifest with increased light sensitivity when changing from a defined scotopic level to a defined mesopic or photopic level, complain of blinding effects even under normal daylight conditions. This can particularly impair reading and discrimination of fine visual pattern. In addition, patients can report visual discomfort, with a sense of eye burning and headache. Patients with impaired dark adaptation, as demonstrated by reduced or absent enhancement of light sensitivity after a change from a defined photopic level to a defined mesopic or scotopic level, tend to report that lighting is insufficient even under normal daylight conditions; again, reading and fine visual discrimination may be secondarily affected. Particularly unpleasant is the combination of impaired light and dark adaptation, because patients need more light for clear vision, but at the same time suffer from photophobia when the lighting is enhanced. In such cases, patients have difficulties with fine visual discrimination under mesopic, photopic and scotopic light levels.
For methodological reasons, the course of light and dark adaptation cannot be measured in young children. However, impaired central light adaptation in young children is also associated with visual discomfort, and they may therefore exhibit shunning of light and avoid light sources by head shifting and eye closure (so-called central photophobia). Jan et al. (1993) found this type of avoidance behaviour in 35 (42 %) of 83 children. The majority (77 %) had shown this behaviour from birth, so that it was probably the consequence of pre- or perinatal brain injury. In the Linz study, 22 (26.8 %) out of 82 children experienced central photophobia. Some children with profound CVI show evidence of improved visually evoked potential grating acuity thresholds under low luminance conditions indicating a greater sensitivity at lower levels of ambient lighting (Good and Hou 2006), a feature consistent with the parental observation of better vision under low lighting, and one that can be sought, and integrated into habilitation plans if identified.
4.3.4 Colour Vision
Impairments in colour vision after acquired brain injury (e.g. following severe hypoxia) can either affect colour perception in the contralateral hemifield (homonymous hemiachromatopsia; see also Sect. 4.3.1), or in both hemifields, also including central vision (cerebral achromatopsia), or hue discrimination in the central field of vision can be affected (cerebral dyschromatopsia). The other visual functions, for example, light and form vision, are usually not affected (Zihl 2011) but if they are, difficulties recognising what is seen can be profound. In contrast to congenital colour vision deficiencies of peripheral origin, e.g. red-green colour blindness, cerebral colour vision difficulties are not characterised by specific patterns of deficiency. Patients with complete cerebral achromatopsia report even saturated colour stimuli as appearing pale, or sometimes as dark brownish; colour naming is incorrect or impossible. Those affected may have difficulties with visual object recognition, if colour is a characteristic property for the particular object.
Impaired colour vision in children with CVI has not often been reported. This may be explained by the difficulty of standardised assessment in young children; alternatively, one may assume that colour vision is not as often impaired after brain injury as are other visual capacities (Jacobson et al. 1996; Korkman et al. 1996). Connolly et al. (2008) have found impaired colour hue discrimination in adults who have suffered from moderate cerebral hypoxia. Because cerebral hypoxia is the most frequent aetiology for CVI in infancy, it appears likely that cerebral dyschromatopsia may be more frequent than reported (Adams et al. 2005). In the Linz study, colour discrimination was assessed using a standardised preferential looking technique (see Sect. 6.2); the systematic screening of children may explain the high rate of impaired colour vision (63/82 children, 76.8 %).
There is preliminary evidence that identification of colours may be chronically impaired despite normal colour hue discrimination; because this impairment exists from birth, it has been labelled congenital colour agnosia (Nijboer et al. 2007; Van Zaandvort et al. 2007). Adult subjects with this ‘higher order’ visual dysfunction have difficulties with the association of colours and objects or colour names.
Difficulty or inability to name colours is not uncommon in children with CVI in our experience, and is therefore a differential diagnosis that warrants consideration.
4.3.5 Stereopsis
Stereopsis is the mental facility to see in three dimensions brought about by the disparity of image data provided by the two eyes. Its development starts to become evident at the age of 4 months (see Sect. 2.3.5). The commonest cause of lack of stereopsis is absence of vision or low visual acuity and/or contrast sensitivity in one eye, often related to strabismus and amblyopia. This affects over 3 % of the general population (Ying et al. 2014).
Systematic studies on stereopsis in very young children with CVI do not exist. The additional visual and oculomotor dysfunctions mentioned may also impair development of monocular depth perception, because depth cues cannot reliably be processed and used. Fazzi et al. (2007) reported impaired stereopsis in 74 of 82 (90.2 %) children with CVI. Dutton et al. (1996) found in 4 of 90 children with congenital brain injury striking difficulties to reach correctly in depth to grasp objects, although no motor problem was present. These children systematically over- or underestimated distances and grasping movements fell short or long of target. It is possible that optic ataxia was a contributory element.
After unilateral posterior brain injury, stereopsis may be only moderately impaired; in contrast, bilateral posterior brain injury in adults may cause severe impairment (Miller et al. 1999). Patients with moderately impaired stereopsis may not report difficulties although stereoacuity is reduced when tested. However, patients with severely impaired stereopsis associated with brain injury can experience the three-dimensional world as two-dimensional (so-called astereopsis); staircases appear flat, and stairs can be difficult to go up and down; faces may look very ‘strange’, because the ‘plasticity of expression’ is less evident. In contrast to patients with moderately impaired stereopsis, patients with severe impairment typically may also lose monocular depth perception cues, and can, therefore, not compensate for their astereopsis. Distance perception may also be secondarily affected; distances are under- or overestimated. This change in distance perception may also influence object perception. In case of underestimation of distance, objects may appear larger (macropsia), in case of overestimation they may appear smaller (micropsia) (Zihl 2011).
4.3.6 Perception of Movement
The centres serving perception of movement comprise the middle temporal lobes located at the occipito-parietal temporal junction. Bilateral damage can cause impaired or absent visual perception of movement (akinetopsia) (Zihl et al. 1983). Only the static visual world can be seen and appreciated in this situation.
Children with periventricular white matter damage commonly show reduced perception of movement, which has been demonstrated with visual evoked potentials to global motion and motion onset (Weinstein et al. 2012; Kuba et al. 2008). Impaired motion perception is common in children born before 34 weeks, and is more marked in those who manifest periventricular white matter lesions (Guzzetta et al. 2009). Specific aspects of motion perception can be deficient, for example the perception of linear motion, while other aspects, such as seeing radial and circular motion may be intact in children with evidence of periventricular white matter pathology (Morrone et al. 2008). Children born extremely prematurely may also have difficulties with motion-defined form processing, but may in addition exhibit problems with visual search, stereopsis, and with visuo-spatial tasks (construction, mental rotation) (Jacobson et al. 2006). In the authors’ experience older children can volunteer that a ball that has been kicked disappears, reappearing when it slows down.
4.3.7 Visual Localisation and Visual Direction Perception
In adults, disorders of visual localisation and perception of visual direction (main spatial axes) have been reported mainly after right-sided occipito-parietal brain injury, i.e. after injury to the right dorsal route. Unilateral right-sided brain injury is associated with moderate inaccuracy in visual localisation (Fig. 4.5); horizontal and vertical spatial axes of perception may be shifted to the contralateral (i.e. left) side, while what appears as the straight ahead direction can be shifted contralaterally, i.e. to the left after right-sided injury and to the right after left-sided injury (Fig. 4.6). In addition, visual orientation may be moderately impaired; this becomes particularly manifest with scanning of a scene or with reading, because patients ‘lose’ lines and paragraphs. After bilateral superior posterior brain injury, the visual-spatial dysfunctions mentioned are more severe; in the extreme case, patients can lose the ability to localise a stimulus (so-called space blindness), cannot navigate in rooms or buildings, and are unable to read (Zihl 2011; Fig. 4.7).




Fig. 4.5
Difficulties with visual localisation in a 56-year-old patient with right-sided occipito-parietal injury (a 11 weeks post-injury), a 52-year-old patient with bilateral occipito-parietal injury (b 13 weeks post-injury), in a 9-year-old boy with CVI (c), and in a 10-year-old boy with CVI (d). Patients in a and b were asked to cancel out the dot stimuli as accurately as possible, while the two boys in c and d were asked to match positions of pairs of dot stimuli. Stars and dots indicate cancellations and matches, respectively. Note the consistent deviations of localisation responses from target positions

Fig. 4.6
Shift of vertical and horizontal spatial axes (broken lines) to the left-sided in a 58-year-old patient with right-sided occipito-parietal injury (a 14 weeks post-injury) and in a 44-year-old patient with left-sided occipito-parietal injury (b 12 weeks post-injury). Note contralateral shifts of subjective axes; shift is typically larger after right-sided brain injury. (c) Shows contralateral shifts of subjective visual midline (broken lines) in a 51-year-old patient with right-sided occipital injury (7 weeks post-injury; left figure), and in a 54-year-old patient with left-sided occipital injury (8 weeks post-injury; right figure)

Fig. 4.7
Fixation in a normal 51-year-old subject (a dot indicates fixation position) and fixation attempts in a 52-year-old patient with bilateral occipito-parietal injury (b star indicates position of fixation stimulus). (c) Pointing responses (recordings with a touch screen) of the same patients as in b. Note inaccuracy of visual localisation in both oculomotor and limb movement systems
In children with CVI, ‘pure’ disorders of visual space perception have only rarely been reported, which may be explained by the fact that visual spatial capacities can be assessed reliably only after the age of 3–4 years. Stimulus localisation can be similarly impaired as in adults (Fig. 4.5). Riva and Cazzaniga (1986) found impaired discrimination of line length and orientation of objects in 6- to 12-year-old children who had sustained brain injury in early childhood. Similar observations were reported by Meerwaldt and van Dongen (1988) in two 7-year-old children with early brain injury; in some children the degree of impairment decreased with increasing age. The outcome of both studies indicates that early brain injury can cause persistent visual-spatial deficits, but advancing visual experience may ameliorate severity. In the Linz study (see Table 4.5) we found visual-spatial impairments in about 75 % of 82 children, which became mainly manifest as inaccurate grasping and pointing and in difficulties with spatial orientation in rooms. Visual spatial difficulties may also affect copying and drawing of figures (Fig. 4.8), whereby it often remains unclear, whether visuo-constructive difficulties are primarily caused by visuo-spatial impairments, or by visuo-motor deficits (optic ataxia), or both. Dutton et al. (1996) found in 6 of 90 children with CVI significant difficulties with accurate and reliable spatial and topographical orientation. In addition, children also found it difficult to learn routes. This observation is supported by the study of Pavlova et al. (2007). These authors tested 14 young people at the age of 13–16 years with periventricular leucomalacia (PVL; see Sect. 4.5.3) using a maze and found considerable deficits in visual-spatial orientation. There is evidence that the learning of so-called landmarks, which allow accurate and reliable spatial and topographical orientation, can be specifically affected in children with CVI (Brunsdon et al. 2007). In addition, impaired ordering of landmarks properly with respect to spatial axes can also affect the acquisition of a reliable spatial reference system (McCloskey 2004).


Fig. 4.8
Copying by a 9-year-old boy with Williams syndrome (a; Eriksson et al. 2003. © Elsevier reprinted with permission) and drawing from memory of an 8-year-old boy with developmental visual agnosia (b; Ariel and Sadeh 1996). © Elsevier reprinted with permission). Note difficulties with the spatial arrangement of figure elements in a, and oversimplification of figures in b
4.3.8 Form Vision, Object and Face Perception
Form vision is an important prerequisite for visual identification, because figures, objects, faces and also letters consist of forms or parts of forms. The discrimination of forms and figures is important for figure-ground separation, which supports spatial structuring of complex objects and scenes. Patients with impaired form vision and figure perception, and impaired figure-ground separation, respectively, have difficulties differentiating and separating objects from each other. Similar forms, figures and objects are confounded and overlapping parts intermingled; sometimes only parts of figures or objects are used for identification and recognition. Impaired visual identification is known as visual agnosia, which is defined as the inability to identify and visually recognise objects, faces, places and letters despite sufficient visual capacities (visual field, visual acuity, contrast sensitivity, colour and form vision), oculomotor functions (fixation, accommodation, vergence, saccadic eye movements, visual scanning) and cognitive functions (attention, executive function). Identification/recognition in another modality, i.e. by hearing sounds or voices, or by tactile exploration, is preserved; thus visual agnosia is a modality specific ‘higher-order’ visual disorder (Zihl 2011). Visual recognition concerns familiar stimuli, i.e. objects, faces, places, letters, etc. that have been known by the patient for a long time, which ensures that they are reliably and accurately represented in visual memory. A stimulus is first identified on the basis of its features; the outcome of this analysis can be used in a second step for recognition, when properties are available, which allow more or less perfect matching with its mental representation. In the context of developmental visual agnosia, it is important to consider whether the child has had sufficient visual experience, i.e. systematic and repeated visual perceptual learning with complex visual stimuli before brain injury. If this is not the case, then visual recognition cannot take place, because no match can be performed between the seen stimulus and its mental representation in visual memory.
It has been found useful to classify visual agnosia according to the affected visual category. Visual object agnosia refers to the loss of visual recognition of objects; prosopagnosia to the loss of visual recognition of faces; topographagnosia is the inability to recognise and navigate places, and paths; letter agnosia (also known as pure alexia) means the loss of recognition of letters. Each category of visual agnosia can occur in isolation, but a combination is the rule (Farah 2000). In contrast to prosopagnosia and agnosia for letters, which have been reported frequently in adults as isolated forms of visual agnosia, object agnosia has not. Lissauer, who published the first detailed single case report on visual agnosia in 1890, pointed out that the typical error is misidentification of objects, i.e. his patient used features that are also shared by other objects. It seems as if this patient still possessed the capacity to identify visual stimuli, but neither monitored the identification process (what are the significant features of the object in question?) nor controlled the outcome of his visual analysis. Thus, visual agnosia may consist of two components: a failure in identification, because specific object properties are no longer known and are therefore not discovered, and a failure in monitoring and controlling the process of recognition and its outcome; the latter capacities belong to executive functions. However, this is not a global inability to recognise, monitor and control identification and recognition of stimuli, but a modality-specific one, because these capacities are still normally operating in other modalities. Of course, for diagnosing a failure in visual recognition, it is essential to assess all prerequisites mentioned above; only if they are sufficiently available can the diagnosis of visual agnosia be made. Thus, one should carefully differentiate between visual agnosia as a primary visual disorder, and impaired visual recognition secondary to visual, oculomotor or cognitive disorders, as outlined above (see also Serino et al. 2014). Furthermore, difficulties with naming visual stimuli are not necessarily due to visual agnosia, they can also result from disconnecting visual naming from visual perception (visual anomia). In the author GD’s practice the most frequently occurring visual anomia relates to the inability to name colour in children of an age where this would be universally expected, colour anomia.
Impairments in visual form perception and in visual recognition in children with CVI may be expected in association with reduced visual acuity and inaccurate fixation, both occurring frequently in combination (see Table 4.3, p. 69). According to the definition of visual agnosia given above, these impairments are classified as secondary disorders. There exist, however, also genuine (‘primary’) disorders of visual recognition. In a study on 90 children with early brain injury, 20 % exhibited low visual acuity, and 10 % severe impairment of all visual functions/capacities (Dutton et al. 1996). Six children in this group showed evidence of a primary impairment in visual recognition. Stiers et al. (1998) assessed 22 children with early brain injury at the age of 4–14 years, and found difficulties with visual recognition in 17 children, independently of visual acuity values. The main problems were integration of features to a whole (Lissauer’s ‘synthesis’), object constancy, i.e. recognition of the same object under different conditions (perspective, context; see Sect. 2.3.7) and checking the outcome of recognition for plausibility (controlling the outcome of recognition) by referring to the context. Brain injury had typically occurred in occipito-temporal structures (ventral route) in both hemispheres, but it cannot be excluded that unilateral injury to these brain regions may also cause such deficits, as is known to occur in adults (Landis et al. 1988; Barton 2008). In the Linz study (cf. Table 4.5, p. 71) visual recognition difficulties were found in 76 out of 82 children (92.7 %). However, because all children also exhibited low visual acuity, it seems plausible to assume that in many cases visual recognition was (also) secondarily impaired. All children with impaired visual recognition, either of primary or of secondary origin, had difficulties visually identifying objects, faces and scenes. Difficulties with face recognition, including familiar faces (parents, brothers and sisters) comprised the most frequent type of recognition disorder (see also Eriksson et al. 2003). Selective developmental agnosia for objects but normal processing and recognition of faces has been reported by Germine et al. (2011) in a 19-year-old female indicating that visual recognition memory for faces is independent of object recognition and storage.
Face perception and recognition in children can be impaired in different ways. Global processing of faces may be impaired but local processing of facial features is preserved and more or less exclusively used (Avidan et al. 2011; Palermo et al. 2011). Visual imagery for faces may be difficult while visual imagery for objects may be normally developed (Tree and Wilkie 2010). An illustrative example of developmental visual agnosia has been reported by Ariel and Sadeh (1996). An 8-year-old pupil, LG, who had normal cognitive, language and motor development, was assessed. He complained of having had ‘peculiar problems’ with his eyes since his sixth year, especially in school. His mother reported that LG had had difficulties from early childhood, in recognising his grandparents’ faces and putting together even simple puzzles. Detailed ophthalmological and neuropsychological assessment did not reveal any visual, oculomotor or cognitive impairment. However, LG had difficulties in visual identification of letters and partly also of words; in contrast, writing and (mental) calculation were normal. Form vision was not impaired; discrimination of grey and colour hues was normal, but LG required more time to do this than his peers of the same age. No signs of visual spatial impairments were found, but visual object, and face identification and recognition were considerably impaired (see Table 4.8). LG found integration (synthesis) of parts of objects and recognition of real familiar objects difficult, but he could recognise them easily after tactile exploration. Particularly difficult for LG was recognition of object drawings and objects presented in different perspectives (object constancy). LG also exhibited great difficulties with discriminating and identifying unfamiliar and familiar faces in photographs, including his own face, but also identification of age, gender and expression (anger, sadness, joy) of the face holder. Interestingly, LG outperformed his peers in face discrimination, which may indicate that discrimination was better developed possibly because LG had to use fine facial differences to discriminate between faces, i.e. he had (spontaneously) gained more efficient special visual experience with faces. Asked why he had visual difficulties, LG commented that he did not exactly know what the pictures or objects signified. Thus LG appeared to have never experienced sufficiently the (particular) significance of complex visual stimuli, like objects and faces. Furthermore, similar to Lissauer’s patient, LG misidentified objects with similar features. Copying of objects and figures was characterised by a slavish mode of procedure: each line was exactly copied. Drawing of objects from memory was possible, but representations of objects (comb, spoon, fork, etc.) looked highly simplified (Fig. 4.8), resembling drawings from a much younger child, who uses basic prototypes to draw familiar objects. LG used highly efficient auditory (voice) and tactile recognition strategies for people and objects in his everyday life. His excellent adaptation to the visual demands of everyday life may be explained by the fact that LG had all the mental capacities fully available for developing and optimising efficient compensatory strategies to cope with his visual difficulties. LG provides an excellent example of the development of functional brain plasticity in terms of adaptation to functional anomalies in early visual development. Although no evidence was found in LG for morphological brain alterations, and his developmental agnosia and prosopagnosia may be attributed to genetic abnormalities (Wilson et al. 2010), one is inclined to classify his visual disorder as a form of CVI.
Developmental visual agnosia and prosopagnosia have also been reported in other single case studies (Duchaine and Nakayama 2006; Dalrymple et al. 2012; Susilo and Duchaine 2013). A common symptom of all children with this ‘higher-order’ visual disorder is difficulty integrating parts of objects or faces into a whole (Behrmann et al. 2005). When confronted with the picture of a face, it is very laborious for children to put all the pieces of the face together; this is akin to the effortful scanning of faces in adult patients with acquired prosopagnosia (Zihl 2011; Fig. 4.9). Processing of faces is assumed to be a process of integration of both global and local information (which is less important for many objects). This idea is supported by the notion that children with developmental prosopagnosia may show normal visual object recognition (Duchaine and Nakayama 2005) indicating that processing and coding of both stimulus classes are functionally segregated. But even within developmental prosopagnosia, diversity exists. Some children find it difficult to identify some but not all parts of faces on photographs (Brunsdon et al. 2006); others can correctly identify parts of faces, but cannot recognise faces they have seen very often (Joy and Brunsdon 2002). Children with prosopagnosia may also exhibit difficulties with discriminating and using abstract forms, figures and symbols; additionally, contrast vision (Barton et al. 2003; Lee et al. 2009) and processing of relative distances between facial and non-facial visual features may be reduced (Ramon and Rossion 2010). Finally, it appears that identification of facial expression is more often spared in developmental compared with acquired prosopagnosia (Humphreys et al. 2007).


Fig. 4.9
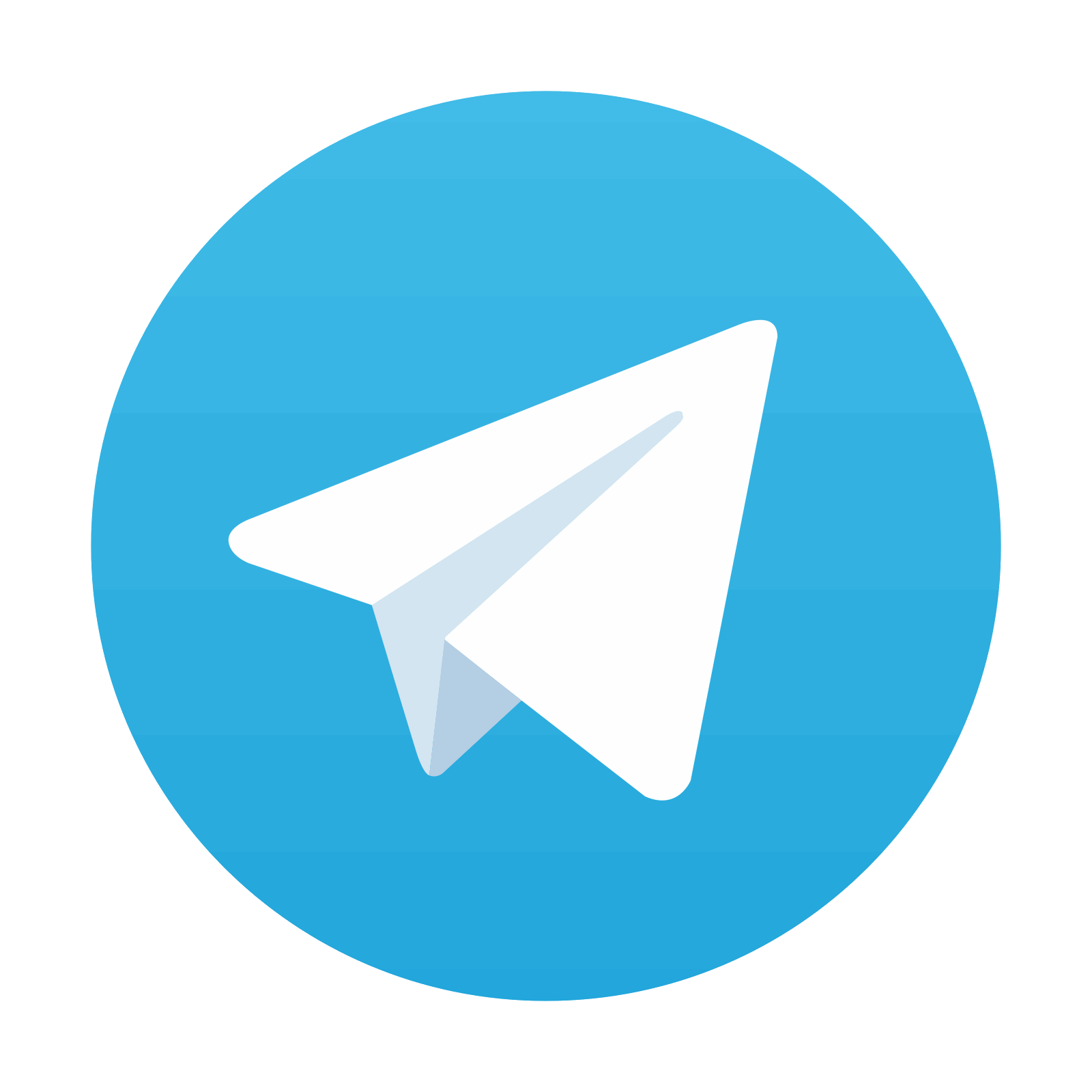
Oculomotor scanning patterns during inspection of a face (centre) in a normal subject (a) and a 62-year-old patient with acquired prosopagnosia after bilateral occipito-temporal injury (b 17 weeks post-injury). Dots: fixation positions, lines; saccadic movements. Note detailed local processing in b
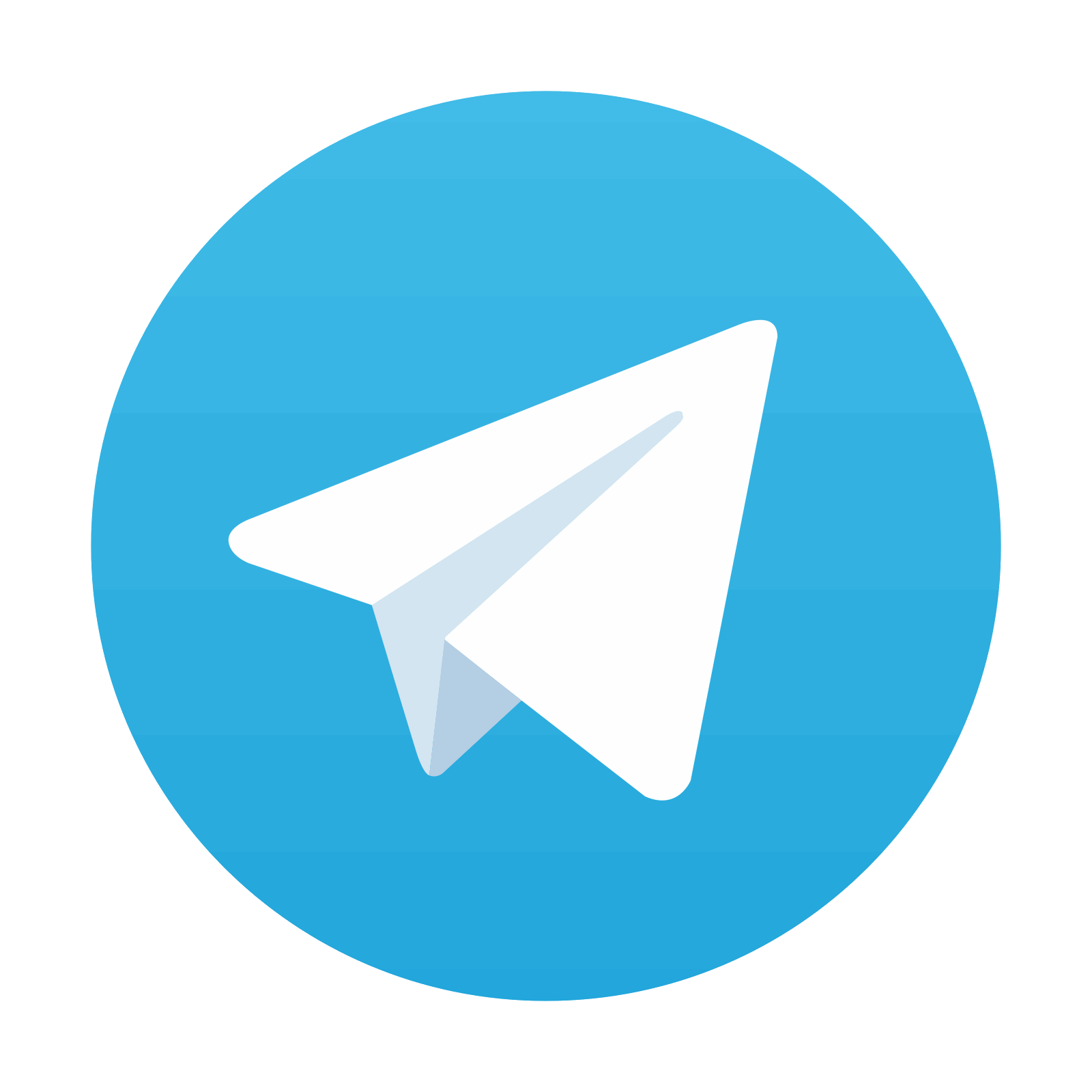
Stay updated, free articles. Join our Telegram channel

Full access? Get Clinical Tree
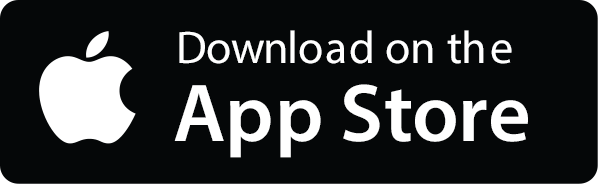
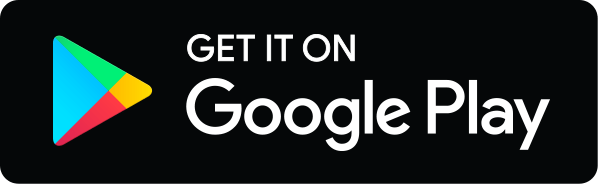
