OKR, optokinetic reflex; VOR, vestibuloocular reflex; NA, not applicable.
Visual motor systems also mediate a set of reflex actions. Compensatory reflexes keep the eyes on target despite body movements. Sensory inputs from the vestibular and visual systems tell the brain that the body is in motion. During movement, the vestibuloocular reflex compensates for acceleration, which is sensed by the vestibular labyrinth, whereas the optokinetic reflex compensates for velocity, which is indicated by movement of the whole visual field (Table 28-1). Visual motor systems also compensate for the amount of light falling on the retina. The pupillary light reflex maintains the level of retinal illumination within the working range of the photopigments in the photoreceptor cells (rods or cones). Finally, rhythmic and reflex blinks of the eyelids protect the eye.
Disturbances of the visual motor systems are common and often produce the first symptoms recognized by a patient. Understanding of these ocular signs provides for timely and effective diagnosis. For instance, strabismus is a defect in which the eyes are misaligned. If this defect is left untreated, the brain reacts to the constant diplopia (double vision) by ignoring the input from one eye and failing to focus it (amblyopia) and, eventually, failing even to orient it. However, amblyopia can be avoided through early treatment of strabismus.
PERIPHERAL STRUCTURES
Extraocular Muscles
The eye is moved in the orbit by six extraocular muscles (Figs. 28-1 and 28-2). These muscles produce movements in the horizontal plane (left and right) around a vertical axis, movements in the vertical plane (up and down) around a horizontal axis, and torsional movements (clockwise and counterclockwise) around an axis running through the center of the pupil to the fovea. There are two pair of rectus muscles, with the members of each antagonistic pair arranged opposite one another on the globe, and a single pair of oblique muscles, which also act as antagonists (Figs. 28-1 and 28-2). For horizontal eye movements, the medial rectus muscle rotates the eye toward the nose (adduction) and the lateral rectus muscle rotates the eye toward the temple (abduction). For vertical eye movements, the primary action of the superior rectus is to rotate the eye upward (elevation), and the primary action of the inferior rectus is to rotate the eye downward (depression). The direction of pull of the superior oblique muscle (Figs. 28-1 and 28-2A, B) is modified because its tendon passes through a loop of connective tissue, the trochlea, on the medial wall of the bony orbit. Its insertion on the globe is caudal to that of the superior rectus. As a consequence, the actions of the superior oblique are intorsion, depression, and abduction. Conversely, the actions of the inferior oblique muscle (Figs. 28-1 and 28-2A, C) are extorsion and elevation as well as abduction.
Figure 28-1. The six extraocular muscles of the left eye in frontal (left) and dorsal (right) views. The primary (long arrow) and secondary (short arrow) actions of each muscle and the position of the pupil after the movement (red oval) are indicated on the frontal view. These muscles originate at the common tendon (annulus of Zinn), with the exception of the inferior rectus, which originates from the orbit’s nasal wall. Rectus muscles insert in front of the equator of the globe, and the oblique muscles insert behind it. Secondary actions are important for clinical evaluation of muscle function. In these cases, the eye is first rotated to align its axis with that of the muscle; for example, for the inferior oblique, the eye is first adducted and then elevated.
Figure 28-2. Coronal (A, B) and sagittal (C) T2-weighted magnetic resonance images showing the relationships of the extraocular muscles within the orbit. The coronal planes are through (A) and caudal to (B), the bulb of the eye. Compare these images with the drawing in Figure 28-1.
The extraocular muscles are supplied by three cranial nerves. The superior oblique muscle is innervated by the trochlear nerve; the lateral rectus muscle, by the abducens nerve; and the medial, superior, and inferior recti and inferior oblique muscles, by the oculomotor nerve. The formula (SO4 LR6) 3 (superior oblique 4, lateral rectus 6, all others 3) is a convenient memory aid for this pattern.
Extraocular muscles are striated and contain fibers adapted to produce extremely high contraction velocities and nearly constant tension. These muscles contain some of the smallest motor units in the body, giving them great precision of movement. The muscles run through connective tissue sheaths that act as pulleys controlling their pulling directions (Fig. 28-3). Each muscle has an inner global layer and an outer orbital layer. The thick global layer inserts onto the sclera of the eye, whereas the thin orbital layer inserts into the connective tissue sheath to regulate the muscle’s pulling direction (Fig. 28-3). Some forms of strabismus may be due to misaligned pulleys. The trigeminal nerve carries sensory information from the extraocular muscles. This proprioceptive signal appears to be critical for normal development of stereoscopic vision (perception of three-dimensional space) and may have other roles. Visual feedback is the primary source of information revealing eye movement accuracy.
Figure 28-3. Frontal section through the orbit showing the extraocular muscles and the connective sheaths (pulleys) through which these muscles pass. These pulleys help define the pulling directions of the respective muscle; see also Figure 28-1. (Photo courtesy of Dr. Joseph Demer.)
Intraocular Muscles
The eyes contain three intrinsic smooth muscles (Fig. 28-4). The ciliary muscle changes the curvature of the lens to bring visual targets into focus on the retina. The sphincter (or constrictor) pupillae muscle and dilator pupillae muscle control the size of the pupils in an antagonistic fashion to regulate the amount of light entering the eyes and the depth of field.
Figure 28-4. The anterior segment of the eye showing the intrinsic eye muscles and optical components (A). The zonule of Zinn (suspensory ligament) extends from the lens capsule and inserts into the epithelium covering the ciliary muscle (B). The pupillary sphincter and dilator muscles are separate muscles in the iris (C). The dilator muscle is actually made up of the myoid processes of the anterior iridial epithelium, as shown in D. The mechanism (left) and effects (right) of accommodation (E, F) are shown. On looking at a nearby target (E), the ciliary muscle contracts (arrows), releasing tension in the zonule and allowing the anterior surface of the lens to round up (arrow) owing to its own elasticity. On looking at a distant target (F), the ciliary muscle relaxes, and the unopposed tension in the zonule (arrows) flattens the lens (arrow).
The ciliary muscle, found in the ciliary body, is connected to the lens by the suspensory ligaments (zonule of Zinn). These fine connective tissue threads resemble the spokes of a bicycle wheel. The action of the ciliary muscle changes the shape of the lens (via the zonule) to adjust its refractive state and focus the image on the retina (Fig. 28-4E, F). These changes are termed lens accommodation. Constriction of the ciliary muscle is produced by activation of cholinergic postganglionic parasympathetic fibers from the ciliary ganglion. With age, the lens grows less elastic, so that the actions of the ciliary muscle have less effect on refraction. This loss of accommodation produces a blurring of near vision termed presbyopia. Myopia, a loss of distant acuity, generally appears at an early age and may be due to genetic factors or overwork at close focal distances (e.g., reading).
The sphincter pupillae is a ring-shaped muscle that lies along the pupillary margin (Fig. 28-4A, C). It contracts in response to activation of cholinergic parasympathetic fibers from the ciliary ganglion to constrict the pupil (miosis). The dilator muscle is radially arranged, so that its action folds the iris and draws open the pupil (mydriasis). The dilator is activated by adrenergic postganglionic sympathetic fibers from the superior cervical ganglion.
Eyelid
The eyelid is controlled by the levator palpebrae superioris, the orbicularis oculi, and the tarsal or Müller muscles (Fig. 28-5; see p. 395). The levator palpebrae is supplied by cranial nerve III. This muscle originates with and travels parallel to the superior rectus but continues forward to insert into the upper lid (Fig. 28-2A, C). The levator holds the eyelid up when the eyes are open, and it functions in concert with the superior rectus, increasing the elevation of the lids, when the eyes look up. The orbicularis oculi, supplied by cranial nerve VII, closes the eyes by depressing the upper lid and elevating the lower lid. At the same time, co-contraction of the rectus muscles retracts the eye. The tarsal muscles are small smooth muscles at the edge of the bony orbit. They are supplied by postganglionic sympathetic fibers and help keep the lids open.
Figure 28-5. The structure of the upper eyelid.
CENTRAL STRUCTURES
Oculomotor Nucleus
The oculomotor nucleus (Fig. 28-6A, B; see p. 395) lies near the midline in the inferior part of the periaqueductal gray of the rostral midbrain. Beneath it lie the fibers of the medial longitudinal fasciculus, many of which synapse within the oculomotor nucleus. Axons from oculomotor motor neurons generally pass medial to the red nucleus and exit the midbrain just medial to the crus cerebri. These structures are supplied by paramedian branches of the basilar artery and the proximal part of the posterior cerebral artery (P1 segment). Vascular lesions in this region produce oculomotor deficits in combination with other symptoms (Table 28-2).
Figure 28-6. Frontal sections through the human oculomotor complex at rostral (A) and caudal (B) levels. The tissue in A has been stained immunohistochemically to reveal the presence of choline acetyltransferase, which is found in cholinergic motor neurons. Thus the motor neurons within the oculomotor nucleus as well as the motor neurons found in the preganglionic subdivision of the Edinger-Westphal complex are stained. The peptidergic cells of the centrally projecting subdivision of the Edinger-Westphal complex are not stained. B is a Nissl-stained section showing the oculomotor nucleus and select adjacent structures. (Photo in A courtesy of Dr. Anja Horn.)
Table 28-2 Summary of Lesions of the Exiting Oculomotor Nerve
The third cranial nerve passes along the wall of the cavernous sinus and enters the ipsilateral orbit by way of the superior orbital fissure. Its three branches supply the superior rectus and levator palpebrae muscles, the inferior rectus and inferior oblique muscles, and the medial rectus muscle. The motor neurons supplying each of these individual muscles form rostrocaudally oriented columns within the nucleus (Fig. 28-7; see p. 396). The motor neurons supplying the levator palpebrae superioris muscle form a separate dorsal midline subnucleus called the caudal central subdivision.
Figure 28-7. Lateral view of the oculomotor and related nuclei (A). The motor neuron pools in the nucleus are shown in frontal sections through anterior (B) and posterior (C) parts of the nucleus. The names of the major nuclei are indicated on the left in B and C, and the targets of each subdivision are indicated on the right in B and C. As indicated by the stippled and clear areas, these motor neurons project ipsilaterally, with the exception of the contralaterally projecting superior rectus motor neurons and the bilaterally distributed levator motor neurons. The axons of contralateral motor neurons cross immediately to join the ipsilateral oculomotor nerve. Thus the oculomotor nerve projects entirely to ipsilateral muscles.
Edinger-Westphal Complex
The Edinger-Westphal complexconsists of the Edinger-Westphal centrally projecting nucleus and the Edinger-Westphal preganglionic nucleus. In humans, the centrally projecting cell group wraps around the posterior medial corner of the oculomotor nucleus but is not related to oculomotor function. These neurons project diffusely in the brainstem and spinal cord and are peptidergic. The preganglionic nucleus lies on either side of the midline, immediately posterior to the centrally projecting group. It contains cholinergic, preganglionic motor neurons whose axons project to the ciliary ganglion to control the lens and pupil (Figs. 28-6 and 28-7). The preganglionic fibers travel with the ipsilateral oculomotor nerve and synapse in the ciliary ganglion. Cholinergic, postganglionic motor neurons send their axons to the globe via the short ciliary nerves. These motor neurons supply the ciliary muscle and pupillary constrictor, with the great majority supplying the ciliary muscle. Thus, in Weber syndrome (Table 28-2) or other lesions of the oculomotor nerve, loss of the preganglionic fibers may result in ipsilateral mydriasis (dilation of the pupil) and paralysis of accommodation.
Trochlear Nucleus
The trochlear nucleus is a small, ovoid group of neurons nestled in the medial longitudinal fasciculus of the caudal midbrain (Fig. 28-8; see p. 396). These motor neurons supply the contralateral superior oblique muscle. The axons forming the trochlear nerve arch dorsally and caudally around the periaqueductal gray, cross the midline in the anterior medullary velum, and exit the dorsal surface of the brainstem at the base of the inferior colliculus. The nerve courses laterally and then anteriorly, hugging the surface of the midbrain. It passes rostrally along the wall of the cavernous sinus before entering the orbit via the superior orbital fissure to innervate the superior oblique muscle.
Figure 28-8. The trochlear nucleus and nerve shown in a dorsal view of the midbrain (A) and in a coronal section through the nucleus (B).
Abducens Nucleus
The abducens nucleus is a spherical cell group located in the facial colliculus, adjacent to the internal genu of the facial nerve in the caudal pons (Fig. 28-9 [see p. 396]; also see Figs. 12-10 and 12-12). Abducens fibers pass slightly caudally and then anteriorly (ventrally) to exit near the midline at the pontomedullary junction. En route, they pass adjacent to the medial lemniscus and corticospinal tract. Owing to this relationship, loss of paramedian branches of the basilar artery compromises both the corticospinal tract and the exiting abducens fibers, resulting in a contralateral hemiplegia and paralysis of abduction in the ipsilateral eye (Foville syndrome). This pairing of motor symptoms is called an alternating hemiplegia or crossed deficit. Crossed deficits can also occur in association with cranial nerves III and XII. After exiting the brainstem, the abducens nerve (cranial nerve VI) enters the dura and ascends the clivus. It then passes beneath the petroclinoid ligament (the Dorello canal) to enter and pass through the cavernous sinus. Cranial nerves III, IV, and VI and branches of cranial nerve V may be damaged in this sinus, singly or in combination, by pituitary tumors or carotid aneurysms. Cranial nerve VI supplies the lateral rectus muscle, so damage to this nerve in isolation will paralyze abduction ipsilaterally (Fig. 28-10; see p. 397).
Figure 28-9. Human abducens nucleus and adjacent structures in Nissl-stained cross section showing neuron cell bodies.
Figure 28-10. Paralysis of abduction in the patient’s left eye on looking to the left. The computed tomography scan shows demyelination of the pons at the level of the exiting left abducens nerve (arrow).
Abducens Internuclear Neurons
Conjugate eye movement is achieved in the horizontal plane by a pathway from the abducens nucleus to the contralateral medial rectus subdivision of the oculomotor nucleus (Fig. 28-13, green
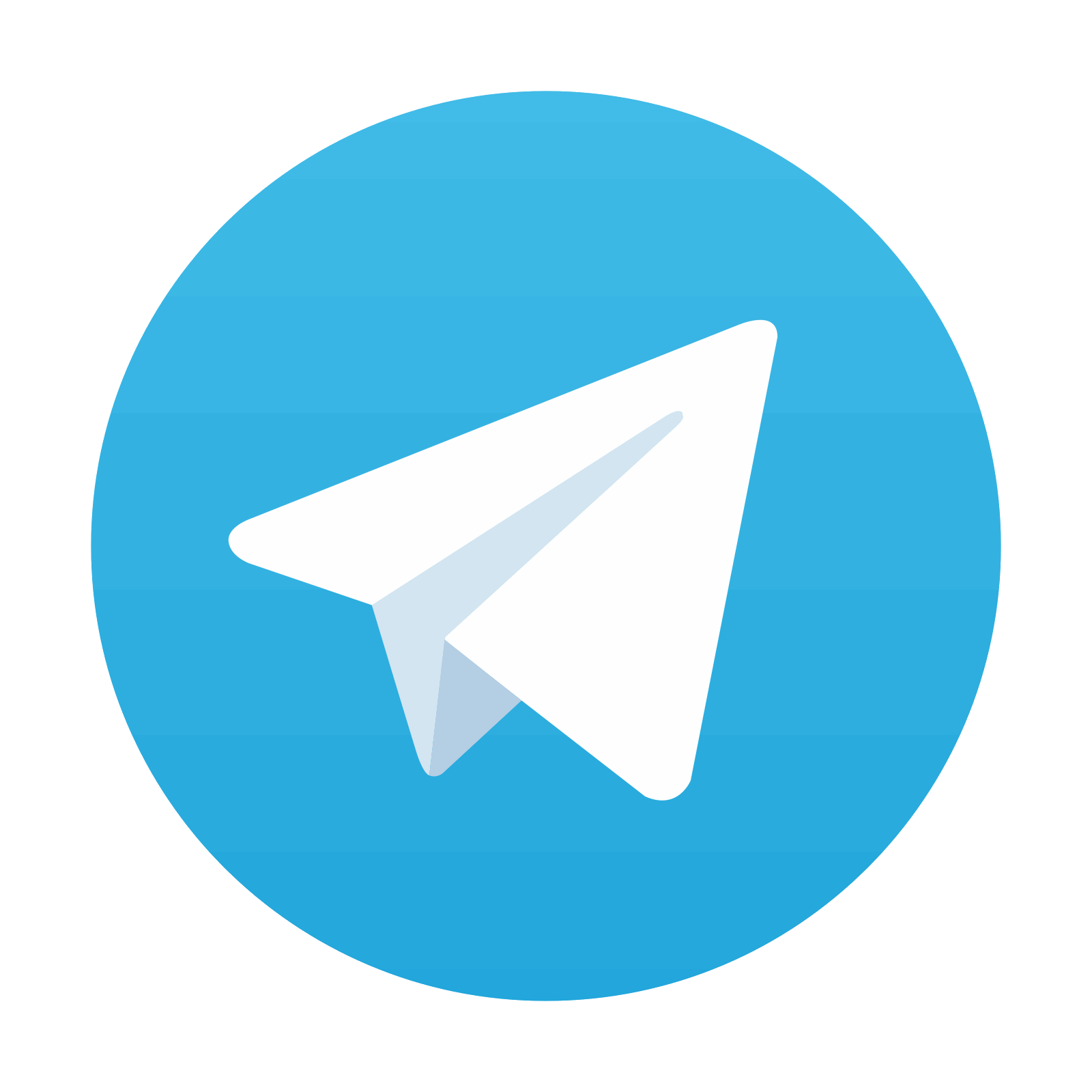
Stay updated, free articles. Join our Telegram channel

Full access? Get Clinical Tree
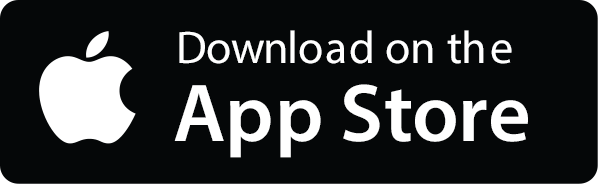
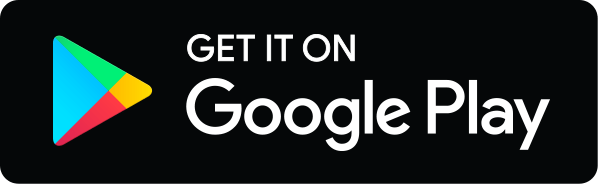