Figure 14.1 Diagram of screenshots depicting the order and timing of events in a computerized Corsi blocks procedure. The example shown depicts a two-location trial. Events run in the direction of the arrow and represent (1) initial presentation of array of blocks, (2) presentation of first location, (3) presentation of second location, (4) five-second retention interval with presentation of uniform field and fixation point (screenshots from conditions with continuous pursuit eye movements and visual noise also illustrated), (5) block array reappears and participants indicate position and order of presented locations using the PC mouse. Reproduced from Pearson and Sahraie (2003).
a significantly greater reduction in span than comparable conditions in which covert attention shifts or limb movements occurred while the eyes remained fixated (Figure 14.2). The type of eye movement performed during the retention interval was also found to be important, with saccadic eye movements producing significantly greater interference than continuous smooth pursuit of a moving target. Finally, analysis of the type of recall errors committed by participants showed that both saccadic and smooth pursuit eye movements produced a significantly greater proportion of spatial location errors (i.e., selecting a location that was not part of the presented sequence) than temporal order errors (i.e., selecting a presented location in the incorrect serial order) in comparison with non-eye movement conditions.
An earlier study conducted by Lawrence, Myerson, Oonk et al. (2001) had proposed that all spatially directed movement produces similar effects within visuospatial working memory. In contrast, Pearson and Sahraie (2003) found that concurrent eye movement produces significantly greater levels of interference than other spatially directed disruptors, and that the strength of interference can be modulated by the type of eye movement being executed. On the basis of these findings it was argued that eye movements can act as a unique form of disrupter for the temporary memory of sequentially presented spatial locations. However, no evidence was found to suggest that participants were using overt eye movements as a rehearsal strategy to retain a sequence of serially presented locations. Participants made no consistent eye movements during (p.234)

Figure 14.2 Illustration of the percentage change in baseline for: (a) Free eye movement; (b) Eyes shut with no movement; (c) Visual noise; (d) Articulatory suppression; (e) Continuous covert attention shifts; (f) Continuous covert attention shift plus color change monitoring and report; (g) Discrete covert attention shifts; (h) Spatial tapping; (i) Smooth pursuit eye movement; (j) Smooth pursuit eye movement plus color change monitoring and report; (k) Saccadic eye movement with eyes shut; (l) Saccadic eye movement with eyes open. Reproduced from Pearson and Sahraie (2003).
In the theory of spatial rehearsal proposed by Awh and colleagues (Awh et al. 1998, 2000, 2005, Awh and Jonides 2001) spatial representations are actively maintained by focal shifts of attention to memorized locations held in working memory. It has been well-established that, while in the majority of cases attention shifts are accompanied by corresponding movement of the eyes or limbs, focal attention can also be dissociated from the direction of gaze (James 1890/1905; see also Posner 1980; Umiltà 1988). In addition, the preparation of an eye movement towards a location appears to automatically induce a concurrent shift of attention towards the same location (p.235) (Chelazzi, Biscaldi, Corbetta et al. 1995; Shepherd, Findlay and Hockey 1986). Therefore, while voluntarily produced eye movements are always accompanied by a corresponding overt shift in attention (Hoffman and Subramaniam 1995; Rizzolatti, Riggio, Dascola et al. 1987), covert attention shifts can also be carried out independently of eye movements.
While previous studies have shown that covert attention shifts alone can disrupt temporary memory for spatial location (Smyth and Scholey 1994; Smyth 1996; Awh et al. 1998), the results of Pearson and Sahraie (2003) show that this disruption is significantly less than that produced by equivalent eye movements. These findings could be accounted for by an attention-based theory of spatial rehearsal if it is assumed that the attention shift associated with a voluntary eye movement is substantially larger than a covert attention shift carried out while the gaze remains fixated. Alternatively, separate processes could underlie both effects. Considering the close integration of attentional and oculomotor processes at the neural level, it may be misleading to consider the temporary maintenance of spatial information in terms of a supramodel model of attention, in which the control of focal attention is viewed as being independent of the processes involved during oculomotor control (Posner 1980; Klein 1980; Klein, Kingstone and Pontefract 1992). As an alternative, Rizzolatti and colleagues have put forward a premotor account of attention which proposes that spatial attention is derived from the operation of maps used to code space for programming eye movements, rather than as a consequence of specific attentional circuits (Rizzolatti 1983; Rizzolatti, Riggio, Dascola et al. 1987, 1994; Sheliga, Riggio and Rizzolatti 1995; Umiltà, Mucignat, Riggio et al. 1994). Support for this view is provided by an fMRI study conducted by Beauchamp et al. (Beauchamp, Petit, Ellmore et al. 2001). They found that covert attention shifts to a target location were produced by moderate degrees of activation in oculomotor control areas, which were sufficient to orient the focus of spatial attention to a target without generating a corresponding eye movement. This suggests that covert attention shifts are mediated by the same processes that mediate oculomotor programming.
Pearson and Sahraie (2003) concluded that the disruption of spatial working memory caused by concurrent eye movement and covert attention shifts were produced by interference in an oculomotor control network rather than a more general attention-based rehearsal mechanism. This can be related to the work of Brockmole and colleagues, who have proposed that visual processing involves an integration between visual percepts and representations stored in visual short-term memory (Brockmole, Irwin and Wang 2003; Brockmole and Wang 2003; Brockmole, Wang and Irwin 2002). Recently Brockmole and Irwin (2005) have examined the role of eye movements during the consolidation and maintenance of information in visual short-term memory. Their participants were required to integrate together two sequentially presented visual arrays that appeared either in the same or different spatial locations. When the spatial location of both arrays remained constant, there was little evidence that overt eye movements were necessary for integration to occur. However, when the position of the second array was different to that of the first, participants made systematic eye movements to the spatial position of the original array during integration. This finding is consistent with previous research which suggests that eye movements may play a functional role in linking together mental and retinal images (Hebb 1968; Brandt and Stark 1997; Spivey and Geng 2001).
Such temporal integration is a key feature of procedures such as the Corsi blocks paradigm (Corsi 1972; Berch, Krikorian, and Huha 1998), in which a series of sequentially presented locations must be integrated together into a single continuous path or movement sequence. A number of previous studies have demonstrated that serial memory for a sequence of locations is affected not just by the absolute number of locations presented, but also by the characteristics of the order in which the locations are presented (Smirni, Villardita and Zappala 1983; Helstrup 1999; Kemps 2001). Parmentier, Elford and Maybery (2005) have recently examined in detail (p.236) how serial memory for a sequence of locations can be affected by different characteristics of a presented sequence. The number of path crossings, path length and mean angular variation of a sequence were all found to significantly affect participants’ serial recall accuracy. Parmentier et al. characterize these factors as representing transitional information, and argue that their influence on serial recall of locations bears similarity to the effect of transitional information on order memory for verbal material (Murray and Jones 2002; Stuart and Hulme 2000).
Whilst visuospatial working memory lacks the type of formal models for serial memory which have been proposed for verbal material (e.g., Henson 1998; Burgess and Hitch 1992), empirical data such as that collected by Paramentier et al. can be seen to represent an essential step in establishing such models. However, while such models may prove valuable in reaching a greater understanding of serial memory than that known for verbal stimuli, their usefulness in terms of a general understanding of visuospatial working memory may be more limited. The development of visuospatial memory procedures such as the Corsi blocks task were strongly influenced by serial recall techniques developed to study verbal short-term memory (e.g., Ebbinghaus 1885/1913; Wickelgren 1965). However, while serial recall of verbal stimuli arguably captures important functional characteristics of verbal short-term memory, the same need not be true of the serial recall of visuospatial material. Auditory input is inherently serial in nature, and accurate representation of serial order forms an essential aspect of the comprehension and recall of linguistic information. In contrast there is much greater variety in terms of visuospatial input, where the same series of locations can be presented either sequentially or simultaneously (Logie and Pearson 1997; Pickering, Gathercole, Hall et al. 2001; Rudkin, Pearson and Logie, 2007). The functional value of preserving serial order outside the artificial constraints of experimental learning paradigms is considerably weaker for visuospatial working memory than that established for verbal working memory. Parmentier et al. (2005) have argued that participants’ performance on serial visuospatial tasks should be considered as a problem-solving exercise. They note that, in contrast to verbal tasks, visuospatial serial recall does not seem to benefit from a well-practiced rehearsal strategy such as subvocal rehearsal, nor does it involve well-established preexisting representations in long-term memory. This view is consistent with studies which have shown a heavy executive involvement in visuospatial short-term memory (Baddeley, Cocchini, Della Sala et al. 2007), as well as recent data which shows greater executive involvement in serial sequential visuospatial tasks than simultaneous tasks that do not require serial recall (Rudkin, Pearson and Logie, 2007). If this is accepted, however, it raises the issue of whether there may be alternative paradigms that better capture how visuospatial working memory might operate under more naturalistic settings. The second half of this chapter will concentrate on one such alternative: multiple-target tracking.
Working memory involvement during multiple-target tracking
In addition to the limitations of focusing on serial recall, paradigms such as Corsi blocks or similar variants only present participants with static target locations that, although they may become occluded during a retention period, do not change spatial position relative to the viewer. Such procedures seem poorly equipped to capture the dynamic nature of the environment we live in. Navigation through a complex three-dimensional environment requires a coordination of movements with a number of objects that may themselves also be in motion. This will require some form of real-time cognitive ability that can simultaneously keep track of a number of moving targets (Allen, McGeorge, Pearson et al. 2004, 2006).
A highly influential account of multiple-target tracking is the spatial index theory proposed by Pylyshyn and colleagues (McKeever and Pylyshyn 1993; Pylyshyn 1994, 2001; Pylyshyn et al. 1994; Pylyshyn and Storm 1988; Sears and Pylyshyn 2000; Trick and Pylyshyn 1989, 1993).
(p.237) The theory proposes that multiple-target tracking is dependent on a limited number of preattentive indexes that can be attracted to targets as they appear within the visual field. Once an index has been assigned it will continue to point to the target even if it moves, thereby drawing attentional processes to its location. This spatial index model was first proposed by Pylyshyn and Storm (1988) who studied participants’ ability to track a number of visual targets amongst a set of distractors. They were required to respond accurately to the onset of a probe which could appear over a target or over a distractor. Their results showed that participants could successfully keep track of up to five targets from a set of ten. However, participants’ accuracy and reaction times declined gradually as the number of targets increased rather than failing catastrophically, which was more consistent with a resource-limited rather than preattentive process. Pylyshyn and Storm argued that this could be due to the operation of a resource-limited serial process which cycled through indexed targets in order to determine which one had been probed.
However, Yantis (1992) provides an alternative explanation. Yantis argues that, prior to the commencement of tracking, targets are grouped together into a single, higher-order virtual object to which attention is then directed – a “virtual polygon” (Yantis 1992, p. 301). Once established this virtual object is then monitored and updated by comparing it with the stimulus input throughout the objects’ motion. Unlike the initial preattentive assignment of indexes to targets, this updating process is dependent upon attentional resources. The points at which the sides of the virtual polygon meet define the moment-by-moment locations of the targets being tracked. Therefore the size, shape, orientation and position of the virtual polygon will change continuously as the individual targets move.
A common feature of the explanations for multiple-target tracking offered by Pylyshyn and Yantis is that both divide the tracking task into two distinct phases: a target acquisition phase, followed by a tracking phase concerned with the maintenance and/or identification of the indexed targets. Where the explanations diverge is in terms of the stage of tracking at which attentional resources become involved. According to Yantis, attentional involvement is vital for the initial formation of the virtual object prior to the commencement of tracking. In contrast, Pylyshyn argues that attention is engaged during the tracking phase, but is not involved during initial target acquisition.
Within the multicomponent working memory framework established by Baddeley, Hitch and colleagues, it would seem reasonable to expect that the visual, spatial and executive components could all make some form of contribution during performance of multiple-target tracking. However, although both Pylyshyn and Yantis propose a tracking phase that requires the temporary maintenance and updating of targets after initial acquisition, the cognitive processes within short-term memory that are responsible have not been clearly specified. Yantis’ description of the formation of a virtual polygon in particular could be viewed as an example of ‘chunking’ phenomena, in which separate items in short-term memory are organized into combined units (Miller 1956; Cowan 2001). Yantis (1992) found that participants who were presented targets in a canonical configuration (thereby providing visual clues about virtual polygon formation) performed better than participants presented with a random display of targets. However, this advantage disappeared after the initial stages of the experiment. Yantis suggested that this was due to participants in the random condition discovering a grouping strategy for themselves. This implies that parts of the target acquisition process could be subject to conscious strategic influences that might engage the resources of the central executive component.
A series of studies conducted by Allen and colleagues have examined the potential role played by working memory during multiple target tracking (Allen, McGeorge, Pearson et al. 2004, 2006). Allen et al. (2004) contrasted multiple target tracking ability in groups of professional radar operators and undergraduate students. The radar operators where classified as experts in (p.238) tracking because of their presumed skill at dynamic spatial tasks, while the undergraduates were classified as novices. All participants completed the tracking task (Figure 14.3) together with a concurrent digit categorization task that took place either before or after the target acquisition stage. Results showed that executive resources appeared to make a contribution to target tracking for both novice and expert groups, but only the novice group showed greater disruption from the categorization task if it occurred during target acquisition. The expert group also outperformed the novice group on the tracking task in all conditions, suggesting that performance may improve given sufficient practice in related spatial tasks. Recently Trick, Jaspers-Fayer and Sethi (2005) have examined tracking performance in children aged from six to nineteen years of age using a modified

Figure 14.3 (a) Target acquisition phase (objects in brackets are flashing on and off). (b) Target tracking phase (objects no longer flashing and all are moving at random). (c) Probe (the probed object is briefly transformed into a square). Reproduced from Allen, McGeorge, Pearson, and Milne (2006).
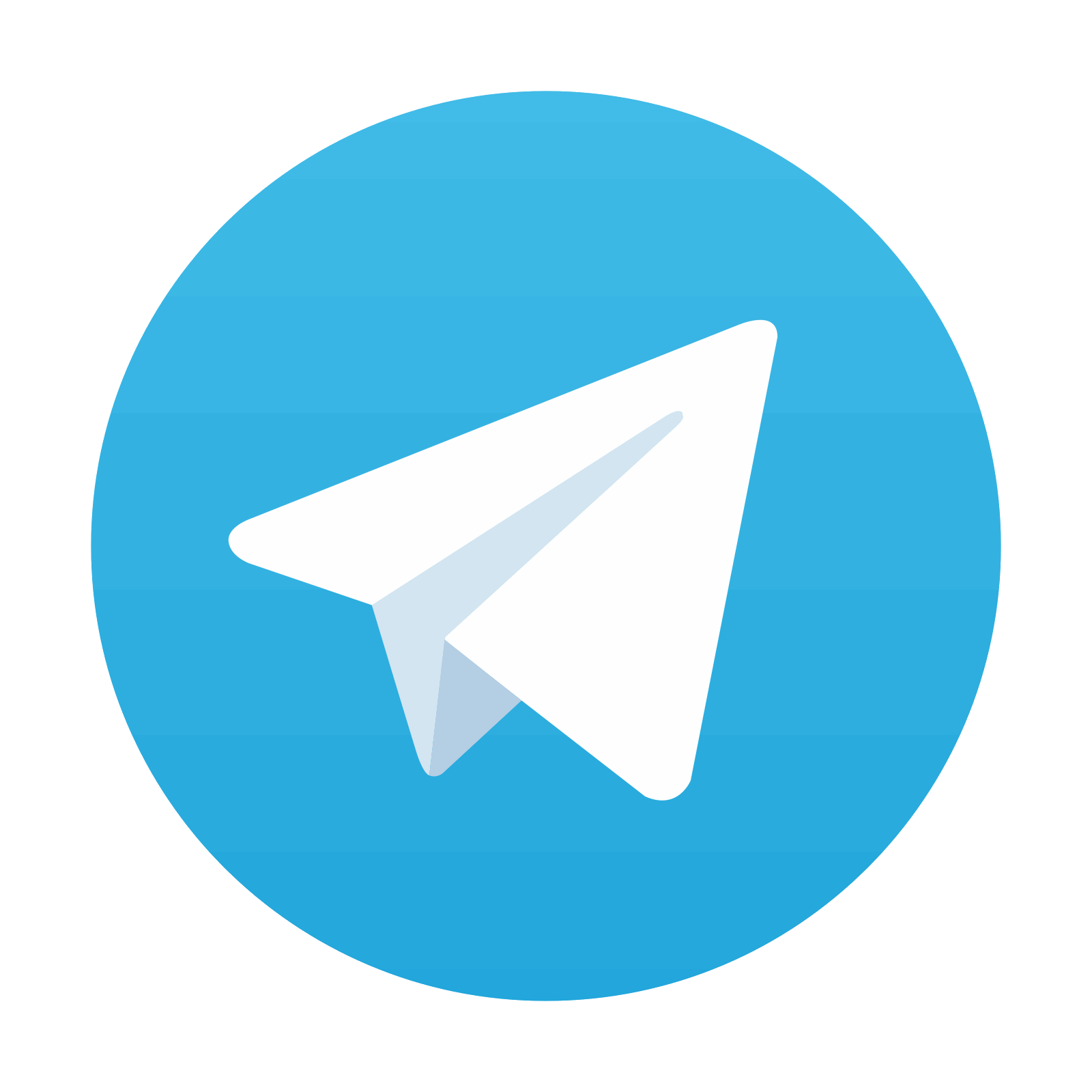
Stay updated, free articles. Join our Telegram channel

Full access? Get Clinical Tree
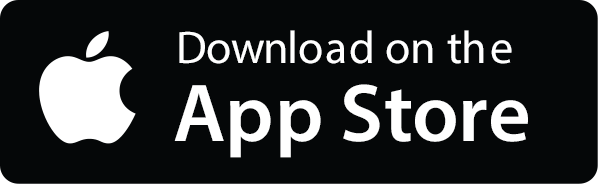
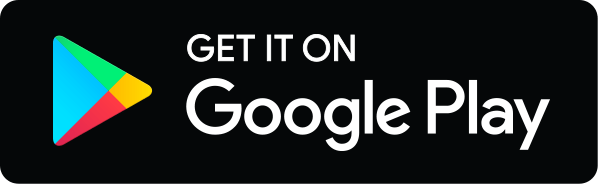