Abbreviations
25(OH)D
25-hydroxyvitamin D, also known as calcidiol
1,25(OH) 2 D
1,25-dihydroxyvitamin D, also known as calcitriol
IOM
Institute of Medicine
UVB
ultraviolet B
SCI
spinal cord injury
VDR
vitamin D receptor
Introduction
Based on data collected from the National Health and Nutrition Examination Survey (NHANES), the prevalence of vitamin D deficiency (< 50 nmol/L) in the United States is estimated to be 39.9% ( ). Historically, vitamin D is known for its role in supporting bone health and preventing osteoporosis ( ; ). However, vitamin D is also important for various functions in the body including immunity, cell growth, and neuromuscular function ( ). The discovery of vitamin D receptors in skeletal muscle tissue has led to an increase in research examining vitamin D and its potential effects on exercise performance. Active individuals with spinal cord injury (SCI) have been suggested to be at an increased risk for vitamin D insufficiency and deficiency due to low dietary intake of foods high in vitamin D ( ), anticonvulsant medications which interfere with vitamin D metabolism ( ), and lifestyle factors such as reduced exposure to sunlight ( ; ). Thus, a sufficient vitamin D (> 75 nmol/L) status is dependent on diet, location of residence, skin pigmentation, time spent outdoors and whether an individual wears sunscreen or sun protective clothing ( ; ). Research has suggested that dietary intake for both micronutrients and macronutrients are insufficient in the para-athlete population ( ).
Vitamin D recommendations: Intake, sources, and status
Vitamin D, also referred to as calciferol, is a fat-soluble vitamin that acts like a prohormone. Vitamin D can be obtained from sun exposure, supplements, and foods like fatty fish, beef liver, eggs, mushrooms, and fortified foods (see Table 1 ), which are the main source of dietary vitamin D in American diets ( ).
Food | Standard Portion | Approximate Vitamin D Content (IU) |
---|---|---|
Fortified foods | ||
Milk, low fat | 1 cup | 117 |
Soy beverage (soy milk) | 1 cup | 119 |
Yogurt, low fat | 8 oz | 116 |
Cheese, American | 1.5 oz | 85 |
Orange juice | 1 cup | 100 |
Nonfortified foods | ||
Salmon (various) | 3 oz | 383–570 |
Rainbow trout, freshwater | 3 oz | 645 |
Light tuna, canned | 3 oz | 231 |
Mushrooms (various | 1 cup | 114–110 |
Eggs | 1 egg | 37 |
Beef liver | 3 oz | 42 |
While there are several forms (vitamers) of vitamin D, two major forms include cholecalciferol (D 3 ) and ergosterol (D 2 ). Ergosterol is added to foods to fortification purposes (i.e., cereal), and it naturally present in fungi like mushrooms. Vitamin D 3 is synthesized in the skin as well as consumed in the diet through animal sources ( ). When exposed to ultraviolet B (UVB) radiation, 7-dehydrocholesterol (7-DHC) is converted into previtamin D 3 , and later converted vitamin D ( ) ( Fig. 1 ).

This type of synthesis is influenced by variables like skin exposure, season, and location of residence. There are seasonal variations in vitamin D synthesis for individuals greater than 35 to 37 degrees N latitude during the winter due to the unavailability of UVB radiation during winter months in these locations. Excessive pollution and cloud cover are also variables that can impact the ability to synthesize vitamin D through UVB radiation ( ). Other variables that affect the ability to synthesize vitamin D via sunlight are sunscreen and clothing choice. Recent research suggests that sunscreen use may not play a large role in low 25(OH)D status when using moderate sun protection factor (SPF). Currently, research has not examined the use of high SPF sunscreens and the effect they may have on 25(OH)D concentrations ( ). Protective clothing as well as increased indoor activity does decrease skin exposure to the sun which may negatively impact 25(OH)D status. Individuals that have dark pigmented skin are at higher risk for vitamin D deficiency as well since darker skin pigmentation requires more sun exposure to absorb the same amount of UVB radiation as someone with lighter skin pigmentation ( ). Fig. 2 displays risk factors for low 25(OH)D status.

Whether synthesized in the skin or ingested through foods and supplements, vitamin D is hydroxylated by the liver into the major circulating form of vitamin D, 25(OH)D, which is the form of vitamin D measured to assess vitamin D status ( ). 25(OH)D is further hydroxylated in the kidneys to form 1,25(OH) 2 D Vitamin D, which is the biologically active form of vitamin D, performing many functions in the body related to its interactions with vitamin D receptors (VDR) ( ). Vitamin D that has been synthesized by the sun stays in circulation two to three times longer than vitamin D that is taken in supplement form. This is because vitamin D taken in supplement form is partially bound to lipoproteins which is cleared from the system before being absorbed ( ). Since vitamin D is a fat-soluble vitamin, there is research showing an inverse relationship between adiposity and low vitamin D status, which puts those who are obese at greater risk for vitamin D deficiency ( ; ).
The recommended intakes of vitamin D from the Institutes of Medicine (IOM) and Endocrine Society are noted in Table 2 . The IOM guidelines are created from a population model aiming to prevent vitamin D deficiency in most of the population ( ). IOM notes that most of the population intakes enough vitamin D to reach “sufficient” serum 25(OH)D levels (20 ng/mL) ( ). Guidelines from the Endocrine Society recommend higher intakes of vitamin D, as it is based from a medical model that incorporates the wide array of vitamin D functions, and suggests 30 ng/mL 25(OH)D levels are beneficial, and that there is a higher upper limit than IOM suggests ( ).
Age | Recommended Intake (IU/day) | Upper Limit (IU/day) |
---|---|---|
National Institute of Medicine | ||
Children (0–18 years) | 400–600 | 2500 (1–3 years) 3000 (4–8 years) 4000 (13–18 years) |
Adults (19–70 years) | 600 | 4000 |
Older Adults (> 70 years) | 800 | 4000 |
Pregnancy/Lactation | 600 | 4000 |
25(OH)D status can be measured in either nanograms (ng/mL) or in nanomoles (nmol/L). The World Health Organization and Endocrine Society considers a vitamin D deficiency as < 50 nmol/L (< 20 ng/ml), insufficiency as 50 to 75 nmol/L (25–30 ng/mL) and a sufficient status would be > 75 nmol/L (> 30 ng/mL) ( ). The best indicator of vitamin D status is a serum concentration of 25(OH)D according to the National Institute of Health due to its 15-day half-life ( ). 1,25(OH) 2 D concentration, on the other hand, is not a good indicator of vitamin D status since concentrations are constantly changing related to metabolic processes ( ).
Function and physiology
Vitamin D plays an important role in many different functions of the body, including immunity, bone health, muscle and neuromuscular function, hormone synthesis, cell development, and gene expression. Vitamin D has also been linked to a reduction in cancer risk, autoimmune disorders, and chronic disease ( ).
Bone health
Vitamin D is known for its role in bone health and prevention of osteoporosis. Vitamin D is vital to the transcription of calbindin, a carrier protein present in enterocytes which aids in the absorption of calcium, the most abundant mineral in bones. Vitamin D is also involved in the regulation of serum calcium and phosphorus, which is important to the processes of bone mineralization and contraction of muscles ( ). In athletes, vitamin D status is important to consider for bone health and preventing fractures ( ). provided 2000 mg of calcium and 800 IU of vitamin D to a group of female Navy recruits while the other group received a placebo for 8 weeks. The supplement group had a 20% lower prevalence of stress fractures than the placebo group ( ).
Muscle function
Vitamin D receptors (VDR) have been identified in nearly every type of cell in the body, including muscle cells ( ). The VDR has either a positive or negative response after being bound with vitamin D which targets gene expression, and therefore may play a large role in regulating skeletal muscle tissue ( ; ). Vitamin D may also affect muscle contractions by influencing calcium levels in the sarcoplasmic reticulum of muscles through alteration in the quantity and/or efficiency of calcium binding sites ( ). In addition to associations found between vitamin D status and muscle weakness, pain, and balance, research has also suggested that vitamin D plays a role in fast twitch muscle fiber morphology. It has been suggested that with an increase in vitamin D status from deficient to sufficient, fast twitch muscle fiber size and number will increase ( ; ). However, further research is needed to determine the role of vitamin D in muscle fiber morphology.
Extraskeletal functions
Adequate vitamin D status has been associated with decreased risk of certain cancers, autoimmune disorders, infectious disease, mental disorders, cardiovascular disease, and Type 2 diabetes ( ). Vitamin D can work as an immune modulator, mainly through interactions with receptors and affecting T-cell differentiation ( ). Vitamin D may decrease risk of cardiovascular disease through action in the regulation of the renin-angiotensin-aldosterone system and inflammatory pathways, although clinical trials in this area have not been conclusive ( ). Vitamin D is involved in neurological function, as VDRs are present on neurons and glia ( ). VDRs are also present on pancreatic beta cells, and may be involved in insulin signaling, glucose control, and reducing inflammation, thereby possibly decreasing risk of Type 2 diabetes, although more research is needed in this area ( ).
Vitamin D status of active individuals with SCI
The vitamin D status of active individuals has become a popular area of study over the past decade. Athletes are potentially at an increased risk for vitamin D deficiency due to the increased enzymatic activity that occurs during exercise ( ). Many studies have found that athletes are at an increased risk for 25(OH)D deficiency during the winter months and that athletes participating in an indoor sport have a higher rate of than those that play an outdoor sport ( ; ; ; ; ; ; ; ; ).
Recently, research has examined the vitamin D status of athletes with a physical impairment. This includes athletes with SCI, amputations, cerebral palsy, and other impairments as well. Athletes with SCI may be at higher risk for vitamin D deficiency due to a lack of mobility and thermoregulatory dysfunction. Athletes with SCI may also have additional malabsorption concerns due to taking anticonvulsant medications ( ; ; ; ). These aspects decrease the amount of time that individuals with SCI spend outside, thus decreasing the ability to synthesize vitamin D by sunlight ( ). It is important for active individuals with SCI to get adequate vitamin D as it is important for bone health.
examined the nutrient intake of elite Canadian athletes with SCI ( ). This study used self-reported food diaries to assess diet and found energy and micronutrient intake, including vitamin D, to be lower than recommended. Scaramella and colleagues further highlighted that para-athletes are at an increased for carbohydrate, iron, protein, and vitamin D deficiencies ( ).
Studies have also focused on 25(OH)D status related to lifestyle factors in athletes with SCI. These lifestyle factors include diet, place of residence, and skin color. Other factors that are important for determining 25(OH)D status in athletes with SCI are whether they play an indoor or outdoor sport, medication use that may inhibit vitamin D absorption and limited time outdoors due to impaired thermoregulation ( ).
Multiple studies have found that athletes with SCI are at greater risk for vitamin D status. found that 96.4% of participants with chronic SCI had an insufficient 25(OH)D status during the winter months, and 81% were insufficient during the summer months ( ). This was further supported by another study in Swiss wheelchair athletes that compared 25(OH)D status between winter and summer months, and as indoor versus outdoor athletes. Flueck et al. found that vitamin D insufficiency was more prevalent in athletes during winter months and in athletes that played indoor sports ( ; ).
An observational study in athletes from the Canadian Wheelchair Sports Association and the US Olympic Committee Paralympic program was conducted to examine the effects of lifestyle factors on 25(OH)D status during the autumn and winter months ( ). There was no significant difference found in vitamin D status between autumn and winter, with 41% to 51% of athletes having an insufficient and 15.4% having a deficient vitamin D status. Time spent outside was significantly correlated with vitamin D status in the autumn, but not during the winter. Furthermore, a seasonal decline in vitamin D status was not observed regardless that athlete sun exposure decreased from autumn to winter. In this study, a greater percentage of athletes that competed in indoor sports were deficient compared to those that played outdoor sports ( ). Thus, research is needed to identify the optimal 25(OH)D levels for active individuals with SCI.
Vitamin D and athletic performance in athletes with SCI
There have been conflicting results in the literature as to whether vitamin D provides an ergogenic benefit for exercise performance and recovery in active individuals. Flueck et al. examined the effects of vitamin D supplementation in athletes with a chronic SCI on 25(OH)D status and whether it would subsequently impact performance ( ). Twenty indoor elite wheelchair athletes received 6000 IU of vitamin D 3 over a 12-week period. After 6 weeks of supplementation, vitamin D status increased significantly and reached an optimal level in all athletes. However, there was no significant difference found for any performance measurements except for isokinetic strength in the nondominant hand, although this result may have been influenced by training. In order to truly test whether the neuromuscular changes were due to vitamin D supplementation or a change in 25(OH)D status, a placebo group is needed ( ).
Another study examined the efficacy of a 12- to 16-week sliding scale vitamin D supplementation protocol on 25(OH)D status and performance measures (handgrip strength, and 20-m wheelchair spring) in elite Paralympic athletes (Pritchett et Al., 2019). Participants with sufficient 25(OH)D status received a maintenance dose (15,000 IU/week of vitamin D), insufficient participants received 35,000 IU/week for 4 weeks and then received the maintenance dose, and participants that were deficient received 50,000 IU/week of vitamin D for 8 weeks and then received the maintenance dose. Mean 25(OH)D status significantly increased after supplementation (66.3 ± 24.3 nmol/mL presupplementation; 111.3 ± 30.8 nmol/mL postsupplementation). There were no significant differences in 20 m wheelchair sprints or handgrip strength; however, 62% of participants did improve handgrip strength.
Conclusion
Further research is warranted in a number of areas surrounding athletic populations, especially athletes with SCI. Recommendations for optimal vitamin D status for the para-populations need to be addressed as this population can be more susceptible to deficiency. Based on previous findings, athletes with SCI should be screened biannually for 25(OH)D status to determine whether supplementation is needed. A 12- to 16-week sliding scale supplementation protocol based on the individual’s vitamin D status can be applied for individuals with an insufficient or deficient status. Furthermore, the relationship between athletic performance and vitamin D status remains unclear and there is a need for more research in this area.
Application to other areas of neuroscience (200–750 words)
This chapter reviewed vitamin D status, functions, and physiology. Vitamin D is important for various functions in the body including immunity, cell growth, and neuromuscular function. The discovery of vitamin D receptors in skeletal muscle tissue has led to an increase in research examining vitamin D and its potential effects on exercise performance. Current research suggests that active individuals with SCI are vulnerable populations with an increased risk for vitamin D deficiency. Active individuals with SCI present unique challenges in terms of nutritional needs, including vitamin D status. Thus, it is important that active individuals with SCI to obtain adequate vitamin D as it is important for bone health. It is suggested that active individuals with SCI may be a at higher risk for vitamin D deficiency due to a lack of mobility, thermoregulatory challenges, and malabsorption concerns due to taking anticonvulsant medications. These aspects decrease the amount of time that individuals with SCI spend outside, thus decreasing the ability to synthesize vitamin D by sunlight. Given that the diet does not supply enough vitamin D, active individuals with SCI may need vitamin D supplementation to obtain optimal vitamin D levels. Active individuals with SCI should receive specific and appropriate nutrition assessments and interventions, according to their needs.
Mini-dictionary of terms
Vitamin D—the fat-soluble vitamin available in food and from the sun.
Cholecalciferol—or vitamin D 3 , the form of vitamin D found in the skin when exposed to sunlight, and the preferred form found in supplements.
Ergocalciferol—or vitamin D 2 found in supplements and in mushrooms and fungi in the diet.
Vitamers—various forms of vitamins that have a similar physiological activity in the body (i.e., cholecalciferol, and ergocalciferol).
Calcitriol—(1,25 hydroxyvitamin D 3 ) the active from of vitamin by made by the kidney but used to treat low calcium in individuals with chronic kidney disease or decreased function of the parathyroid glands.
Key facts of vitamin D and spinal cord injury
- •
Vitamin D is known for its role in bone health and prevention of osteoporosis.
- •
Vitamin D deficiency (< 50 nmol/L) prevalence in the United States is estimated to be 39.9%
- •
Vitamin D status is assessed by serum 25(OH)D concentration
- •
Vitamin D can be obtained from sun exposure, foods, and supplements.
- •
Rickets was one of the first discovered conditions associated with a vitamin D deficiency.
Summary points
- •
Vitamin D is important for skeletal, muscle, immune, and neurological health.
- •
Athletes are at higher risk for vitamin D deficiency, related to increased enzymatic activity during exercise and indoor sports.
- •
Athletes with SCI may be a at higher risk for vitamin D deficiency due to a lack of mobility, thermoregulatory dysfunction, and malabsorption concerns due to taking anticonvulsant medications.
- •
Active individuals with SCI should be screened biannually for 25(OH)D status to determine whether supplementation is needed.
References
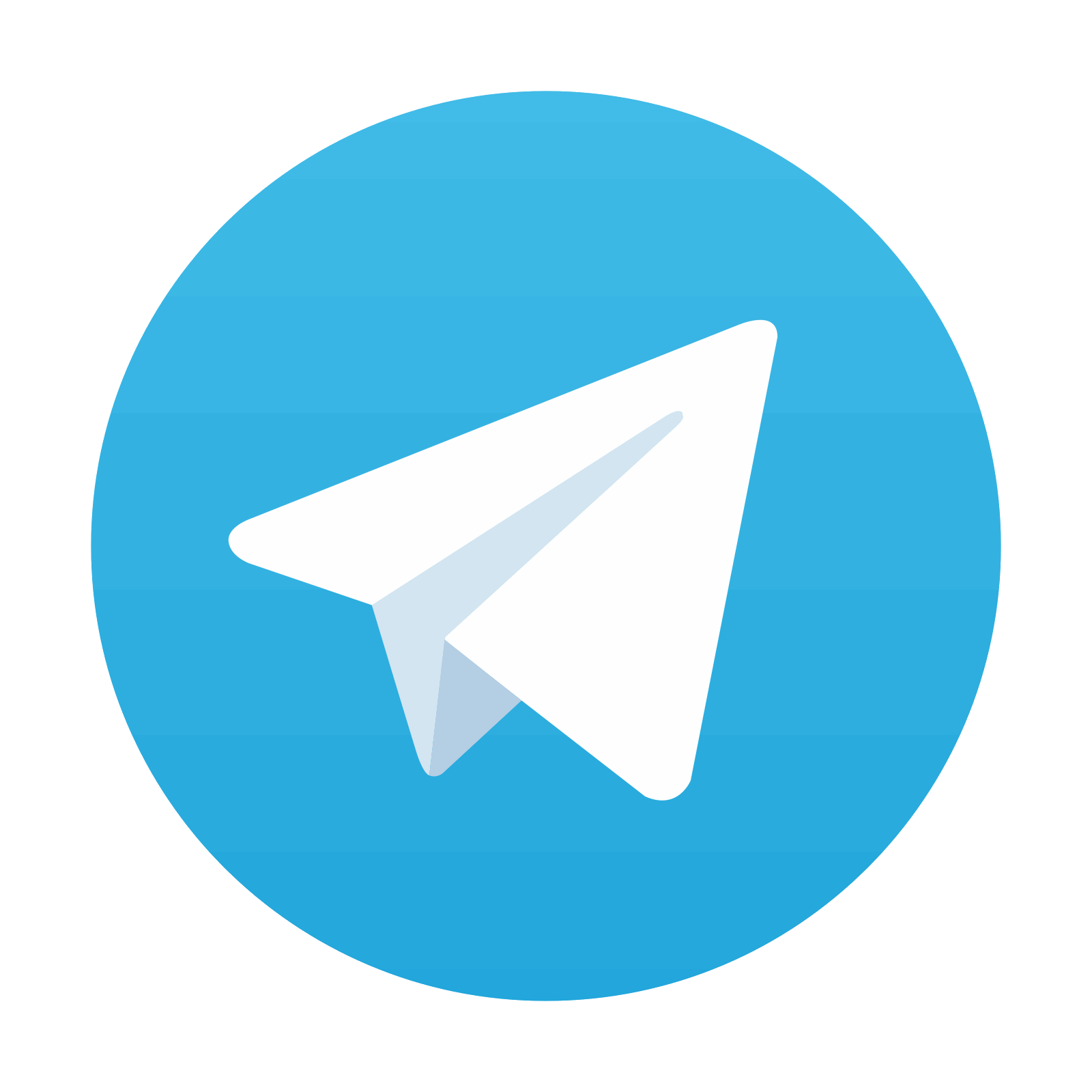
Stay updated, free articles. Join our Telegram channel

Full access? Get Clinical Tree
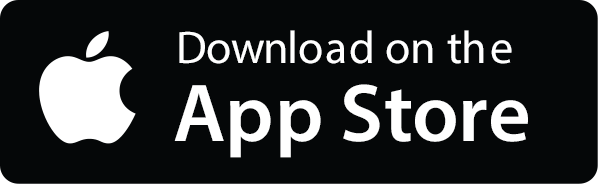
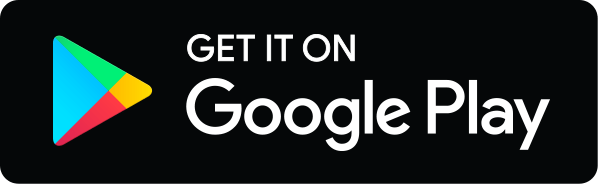
