14
What Are the Best Ventilator Settings for Head-Injured Patients? What Is the Role of Hyperventilation
BRIEF ANSWER
- There is no support for the use of hyperventilation to treat elevated intracranial pressure (ICP) except as a short-term temporizing measure while other diagnostic and therapeutic interventions are initiated (class II and III evidence).
- There is no role for prophylactic hyperventilation to prevent intracranial hypertension (class II and III evidence).
- Chronic hyperventilation may worsen cerebral ischemia (class II and III evidence).
- There is inadequate evidence to either support or refute the use of positive end-expiratory pressure (PEEP) at the end of each breath for brain-injured patients (class III evidence).
- There may be a role for the use of normobaric supranormal concentrations of inspired oxygen (class III evidence).
- Optimal ventilator settings and the use of hyperventilation for brain-injured patients should be individually assessed at the bedside by a practitioner experienced in both ventilatory and neurological issues.
Background
The three major components of the intracranial cavity are brain parenchyma, cerebral blood volume (CBV), and cerebrospinal fluid (CSF). A reduced volume of any one of these components, such as redistribution of CSF to external drainage, may decrease elevated ICP and prevent herniation of an edematous brain. Since the middle of the last century, we have known that hyperventilation dramatically decreases ICP due to cerebral arterial constriction secondary to reduced arterial carbon dioxide tension (PaCO2). However, the desired vasoconstriction may also decrease cerebral blood flow (CBF), resulting in hypoperfusion and subsequent global or focal ischemic injury. Because of these concerns, the dogma of ” therapeutic hyperventilation“ for the treatment of prolonged elevations in ICP has been challenged in recent years. Class II and class III data currently support a management strategy of hyperventilation for acutely elevated ICP to reverse signs and symptoms of brain herniation. Conversely, analyses of patient outcomes, both long- and short-term, do not support the use of prophylactic or continuous hyperventilation in patients at risk of developing elevated ICP or in those who already have chronic elevations of ICP.
Pearl
By decreasing CBV, hyperventilation-induced vasoconstriction may be helpful to lower acutely elevated ICP while diagnostic and other therapeutic maneuvers are initiated.
Pearl
Hyperventilation-induced vasoconstriction may also decrease CBF, resulting in hypoperfusion and subsequent ischemic injury.
Ventilator Basics
Adjustment of ventilator settings allows a caregiver to control a patient’s ventilation and oxygenation. Tidal volume (VT), or the volume of air delivered in each breath, and respiratory rate (RR), or number of breaths per minute, affect the PaCO2; that is, ventilation. Inspired oxygen concentration (FiO2) and positive pressure at the end of each breath (PEEP) affect the PaO2 or oxygenation. The recent literature supports the use of a smaller VT, ~5 to 7 mL/kg of body mass, to reduce barotrauma to the pulmonary parenchyma, especially when lung compliance is decreased (class I data).1 Minute ventilation (VE), which is the RR multiplied by the VT, need not exceed 10 to 12 L per minute to achieve adequate ventilation in most patients. FiO2 has the most influence over PaO2, and PEEP has a supporting role through alveolar recruitment, which allows a greater surface area for oxygen exchange. A balance between FiO2 and PEEP must be established to provide an optimal PaO2 with minimal risks of O2 toxicity and barotrauma.
Review of Cerebral Hemodynamics and Oxidative Metabolism after Brain Injury
Cerebral physiology and metabolism differ greatly between injured and healthy brain. Glucose and oxygen, delivered by the cerebral circulation, are the primary fuels on which the brain functions. Under normal conditions, oxidative metabolism and CBF are coupled, a concept known as ” metabolic autoregulation.“2 Cerebral metabolism is quantified primarily by the difference between oxygen delivery and oxygen extraction by the brain parenchyma, a concept referred to as the cerebral metabolic rate of oxygen consumption (CMRO2). In the normal brain, CBF is maintained at a relatively constant rate, proportional to the cerebral perfusion pressure (CPP) and inversely proportional to cerebral vascular resistance (CVR). This ” pressure autoregulation“ allows for relatively unaltered levels of CBF despite a wide range of perfusion pressures, from as low as 50 to as high as 150 mmHg.2 In the healthy state, ~30 to 40% of oxygen in arterial blood is extracted and utilized, as demonstrated by the cerebral oxygen extraction fraction (CEO2) or the difference between the partial pressures of oxygen in arterial and venous blood (AVDO2). Mathematically, the Fick principle relates O2 metabolism, CBF, O2 extraction (arteriovenous difference of oxygen, or AVDO2), and O2 uptake, such that CBF is proportional to O2 metabolism divided by AVDO2 (O2 metabolism = CBF × AVDO2).
Pearl
When the relationship between CMRO2 and CBF becomes uncoupled, either a hyperemic state (blood flow exceeding metabolic need) or an oligemic state (inadequate blood flow in relation to metabolic demand) may develop.
Under normal circumstances, O2 delivery exceeds the brain’s demand for oxygen. In settings characterized by decreased cerebral oxygen delivery, an increase in oxygen extraction by the brain parenchyma leads to a widened AVDO2 and a constant CMRO2.3 Failure of autoregulatory mechanisms to meet increased oxygen demand leads to insufficient oxygen delivery, metabolic failure, neuronal dysfunction, and cell death.4,5 When the relationship between CMRO2 and CBF becomes uncoupled, either a hyperemic state (blood flow exceeding metabolic need) or an oligemic state (inadequate blood flow in relation to metabolic demand) may develop.6 As predicted by the Fick principle, extreme oligemia (<18 mLO2/100 g brain tissue/minute) results in ischemic injury, with the threshold largely dependent on the rate of oxygen consumption. A decreased CMRO2 lowers the ischemic injury threshold.5–7 For the clinician, this means cerebral ischemia can be identified only after measurements of CMRO2 and AVDO2 in relation to CBF are obtained. There exists class II and III evidence that CMRO2 is markedly decreased after brain injury and that uncoupling exists between CBF and CMRO2.8–10 As many as 30% of patients with severe traumatic brain injury (TBI) demonstrate reduced CBF; in contrast, others have reported that 20% of patients may have elevated CBF when compared with healthy subjects (class II and III data).9–11 This uncoupling makes it very difficult for clinicians to predict from CBF analysis alone which patients are suffering from secondary ischemia because reduced CBF with an even greater reduction in CMRO2 could mean a relatively hyperemic state, with adequate O2 delivery. Some investigators have reported that elevated ICP measurements correlate well with the hyperemic state (class III data)10 and predict a worse outcome when not managed appropriately. What remains unclear is whether further reductions of an already reduced CBF, despite the existence of a relatively hyperemic state, can lead to worsening cerebral ischemia.
Pearl
It is very difficult to predict from CBF studies alone which patients are suffering from secondary ischemia because reduced CBF with an even greater reduction in CMRO2 could produce a relatively hyperemic state, with adequate O2 delivery.
Monitoring Techniques
Several reports have documented early cerebral ischemia in TBI patients, with severity of ischemia predictive of worsened patient outcomes (class II data).12 Within the medical community there is tremendous debate regarding optimal management of patients with TBI, much of which stems from modern medicine’s inability to assess cerebral ischemia with technical precision. Most measurements of cerebral AVDO2 represent average determinations of global cerebral oxidative metabolism and do not assess focal ischemic areas that may exist within the brain parenchyma.13 Conversely, more modern techniques that measure intracranial PO2, pH, lactate, and PCO2 within small anatomic areas may not adequately measure or reflect overall cerebral perfusion.14 There is class II evidence pointing to regional differences in parenchymal oxygen tension (PbtO2) between normal and damaged tissue without histologic evidence of ischemia due to hypocapneic vasoconstriction, possibly because of locally disrupted autoregulation after brain injury.15 Whether the differences in PbtO2 are due to altered vasoreactivity or altered metabolism is not clear, but more work in monitoring cerebral tissue metabolism needs to occur before a consensus is reached.
The role of monitoring CPP to determine cerebral oxygenation status is even less clear. Earlier studies linked elevated CPP with increased cerebral oxygenation and assumed a direct relationship between CPP and CBF.16,17 As discussed above, the mechanism of ” pressure autoregulation“ in healthy brain maintains constant CBF and oxygen delivery over a wide range of perfusion pressures. Investigators examined the link between CPP and oxygen metabolism in brain-injured patients and could not document a relationship between CMRO2 and CPP or between AVDO2 and CPP over a wide range of CPP values (60 mmHg to 130 mmHg) (class II data).18 However, positive correlations were seen between CMRO2 versus CBF, AVDO2 versus CVR, and CMRO2 versus AVDO2, confirming earlier data on the relationship among O2 metabolism, CBF, and O2 extraction. Interestingly, negative correlations were noted between AVDO2 and CVR with CBF. The authors concluded that, in the presence of normal or high CPP, cerebral hemodynamics and metabolic patterns are more closely associated with CVR than with CPP.18 It is important to note that the study did not examine the relationships of CMRO2, CBF, and AVDO2 versus CPP in association with CPP <60 mmHg. These data suggest that CPP may or may not be the optimal parameter for drawing conclusions at the bedside about the status of CBF in brain-injured patients.
Literature Review
Hyperventilation
In the 1970s, investigators discovered that CBF and CBV could be manipulated using hyperventilation, allowing indirect adjustment of ICP.19 These and other data were used to justify the use of hyperventilation in brain-injured patients to manage acute elevations of ICP. It is now recognized, however, that such a practice may be quite deleterious.
The mechanism behind the manipulation of ICP and CBF is adjustment of CSF pH via arteriolar vasoconstriction. This pH-driven vasoconstriction is transitory to the extent that carbonic anhydrase metabolism in the choroid plexus is able to correct CSF pH. Normally, within 4 to 6 hours, there is either normalization of cerebral arteriolar caliber, ICP, and CBF or a rebound effect of hyperemia with increased ICP. Carmona-Suazo et al20 showed that the cerebral arteriolar response to CSF pH was dampened in patients with decreased CBF (class II data).
Pearl
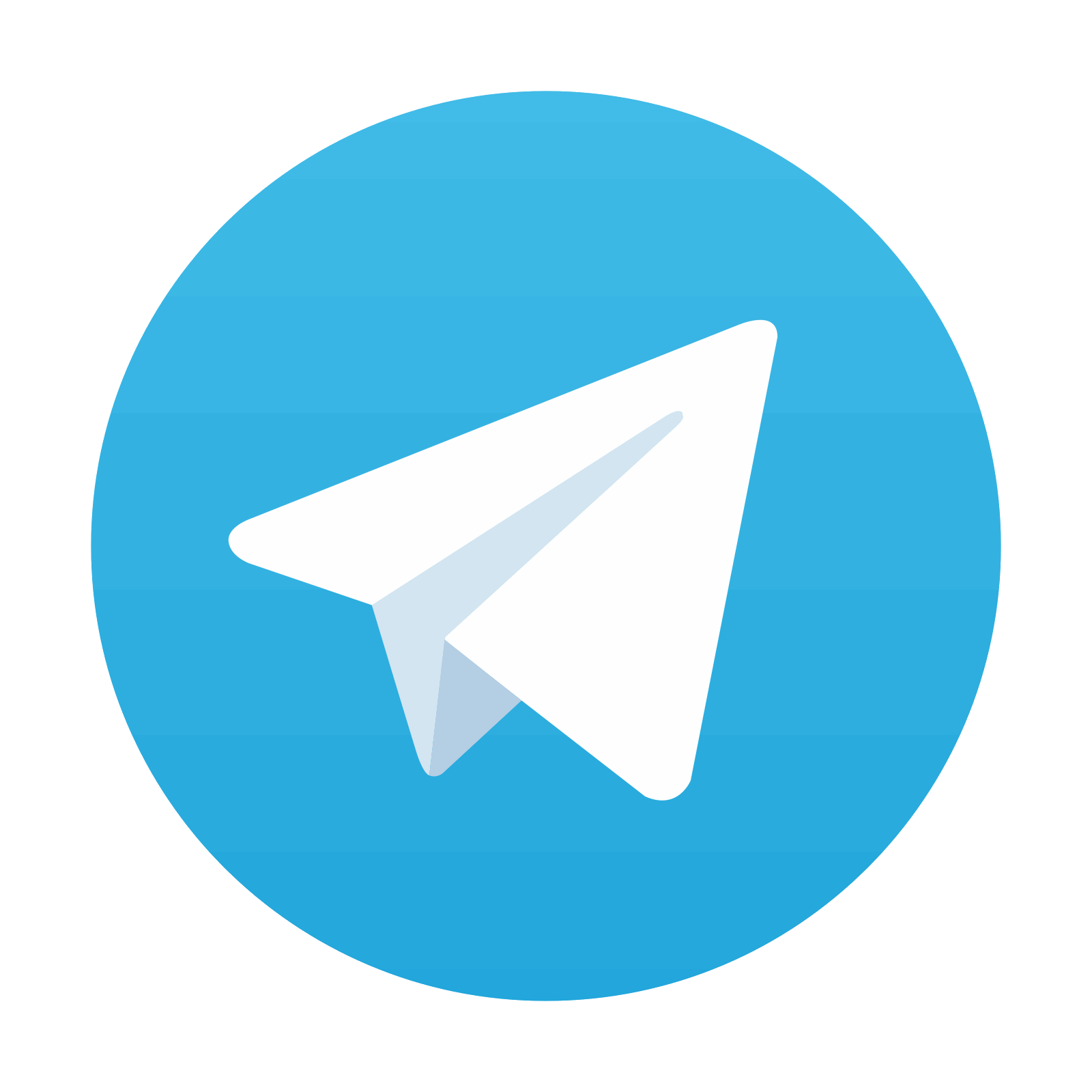