Fig. 3.1
An 84-year-old man with herpes simplex virus encephalitis. The EEG demonstrates lateralized periodic discharges (PLEDs or LPDs) on the left
We recommend starting or maintaining a conventional, nonsedating AED in all patients with PLEDs without escalating treatment unless clear ictal electrographic or clinical semiology is observed. To distinguish electrographic characterization of PLEDs more likely associated with seizures, a distinction between PLEDs proper versus PLEDs plus has been made (Reiher et al. 1991) (Fig. 3.2). The latter are associated with brief focal rhythmic discharges and are more frequently related to seizures. Another concerning findings are PLEDs whose frequency changes periodically over time, accelerating and decelerating, creating a sort of “accordion” appearance. More aggressive treatment may be warranted in such patients.
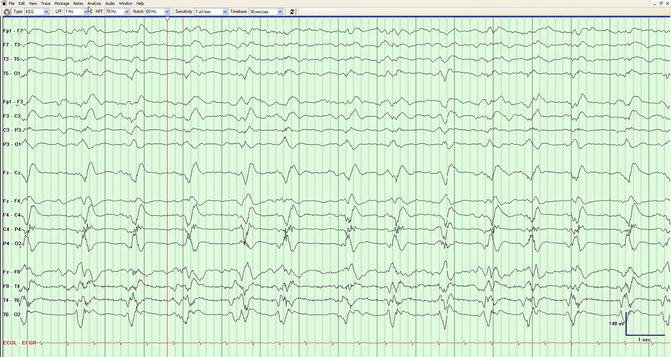
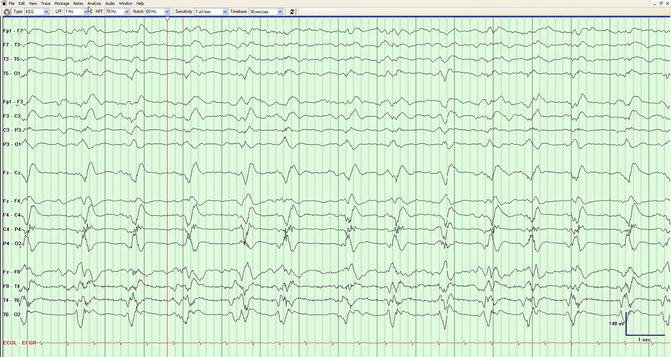
Fig. 3.2
A 78-year-old man with history of poststroke seizures. The EEG demonstrates right PLEDs and superimposed focal fast rhythmic discharges, consistent with PLEDs plus
Although subtle movements are the most common manifestation of clinical seizures in the ICU population, in rare instances seizures may result in “negative” phenomenology. One example is ictal aphasia (Herskovitz and Schiller 2012), which may present with PLEDs without definite electrographic seizures. These aphasias resolve with the administration of AEDs and, as such, are to be regarded as ictal rather than a postictal phenomenon, and the associated PLEDs should be considered ictal as well. Similar presentations of PLEDs causing ictal monoparesis have been reported as well (Murahara et al. 2013). In general, though, there is insufficient evidence to determine whether electrographic PLED suppression is of any significant value, as these generally tend to be resistant to medication; complete suppression is rarely attempted due to the risk of overmedication.
In patients with severe unilateral brain pathology and encephalopathy, pseudo-PLEDs may result because severe pathology in the injured hemisphere suppresses what would otherwise have been generalized periodic discharges, resulting in PLED-like patterns over an intact hemisphere. Aggressive treatment with AEDs therefore may generally not be warranted in such patients.
3.3.2 BiPLEDs/BiPDs
BiPLEDs/BiPDs are defined as periodic discharges which are independently and simultaneously present in both hemispheres (Brenner and Schaul 1990). They are most typically seen in strokes, anoxic brain injury, or CNS infections (de la Paz and Brenner 1981) and are far less common than PLEDs, generally portending a more severe clinical state, as they are associated with higher risk for seizures, depressed consciousness, and mortality (de la Paz and Brenner 1981). As such, greater awareness regarding epileptic activity is required than in PLEDs, though the general approach to AED management is the same.
3.3.3 GPEDs/GPDs
GPEDs/GPDs are repetitive synchronous bilateral discharges occurring at regular or near-regular intervals. Among the periodic discharges, these remain the most difficult patterns to analyze, as they commonly span the gamut from the nonepileptic to status epilepticus, at times within a short period of time. GPEDs are nonspecific and may be seen in nearly any cause of depressed mental status. Although anoxic/metabolic or infectious etiologies were formerly believed to be the most common underlying cause (Husain et al. 1999; Yemisci et al. 2003), a recent large survey found that acute brain injury (44 %), acute systemic illness (38 %), and cardiac arrest (15 %) are often associated with these features (Foreman et al. 2012). The same group reported that GPEDs are more frequently associated with nonconvulsive seizures or nonconvulsive status epilepticus as compared to a control group undergoing EEG monitoring.
There is however a lower risk for seizures in patients whose GPEDs exhibit a triphasic wave morphology (Markand 2003) (Fig. 3.3), defined as complexes with a prominent surface negative deflection discharging every 1–2 Hz, often with an anteroposterior or posteroanterior phase lag (Brenner and Schaul 1990). The American Clinical Neurophysiology Society has defined “triphasic morphology” more formally as complexes with either two (positive–negative) or three (negative–positive–negative) phases, each longer than the preceding one (Hirsch et al. 2013). Generally, it is difficult or impossible to determine solely on waveform morphology whether GPEDs are seizure related or result from metabolic dysfunction (Husain et al. 1999). GPEDs discharging at rates higher than 3 or 4 Hz are generally considered to be of ictal nature (Young et al. 1996; Kaplan 2007) (Fig. 3.4). It has been suggested that a challenge dose of intravenous benzodiazepine might be given in order to sort out the nature of the periodic pattern: a clinical or incontrovertible electrographic improvement (Jirsch and Hirsch 2007) would suggest an ictal phenomenon. In reality, this procedure is difficult to perform, as benzodiazepines will electrographically resolve both ictal and nonictal GPEDs. Furthermore, observing clinical improvement is extremely challenging as benzodiazepines will depress mental status in both scenarios.
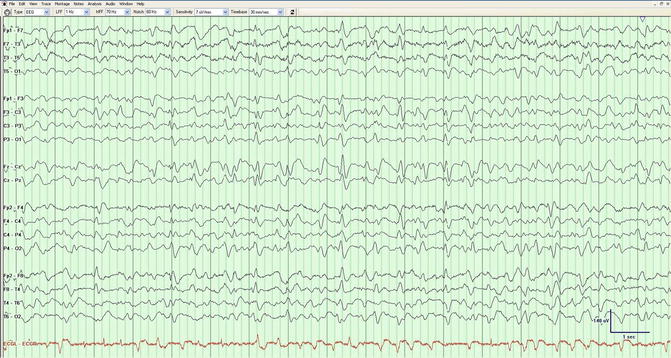
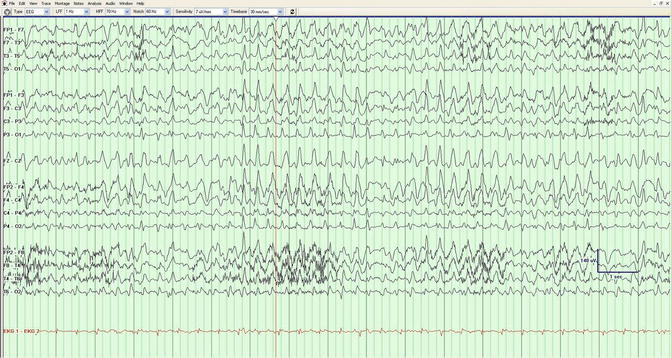
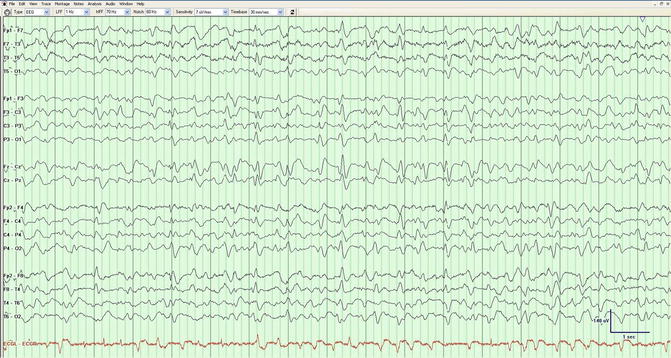
Fig. 3.3
A 61-year-old man with hepatic encephalopathy. The generalized periodic discharges have a triphasic morphology; this pattern should not be considered ictal
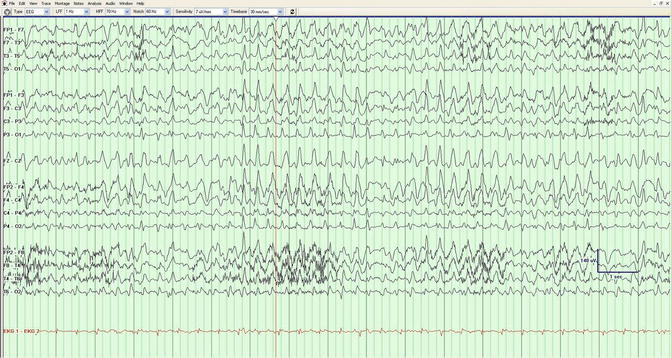
Fig. 3.4
A 64-year-old female after cardiac arrest. Generalized periodic discharges are synchronous with subtle concomitant eye movements, consistent with an ictal phenomenon
In summary, AED management of these patterns must be done in conjunction with careful clinical and pathophysiological assessments. For example, GPEDs with triphasic morphology due to purely metabolic etiologies, without any clinical correlation, should not be treated with AEDs, whereas other GPEDs with clear manifestations of status epilepticus (Husain et al. 1999) deserve an aggressive management.
3.4 Rhythmic Activity (See Also Chap. 5)
Intermittent rhythmic activity, frequently in the delta range, was originally described by Cobb (1945). The most common pattern seen in acutely encephalopathic adults is frontal intermittent rhythmic delta activity (FIRDA) (Zurek et al. 1985). Although initially believed to be due to deep midline lesions causing increased intracranial pressure, it is a nonspecific feature that can be seen in a variety of structural and metabolic conditions, not independently associated with a greater risk of seizures (Accolla et al. 2011; Brigo 2011). As such, specific treatment for FIRDA with antiepileptic drugs is discouraged. Occipital intermittent rhythmic delta activity (OIRDA) is associated with genetic (idiopathic generalized) epilepsies in children (Gullapalli and Fountain 2003). Temporal intermittent rhythmic delta activity (TIRDA) is conversely related to a specific condition – mesial temporal lobe epilepsy (Reiher et al. 1989) – and correlated to seizures (Reiher et al. 1989; Normand et al. 1995). Neither OIRDA nor TIRDA is typically seen in patients in the ICU.
A recent study has explored the significance of lateralized rhythmic delta activity (LRDA), which is defined as unilateral, or bilateral asymmetric, repetitive delta waves having a uniform morphology (Hirsch et al. 2013). This pattern is encountered in approximately 5 % of patients who undergo continuous EEG monitoring (Gaspard et al. 2013), often appearing in short bursts, usually of 1 min or less, and most commonly in the frontotemporal regions. These features were shown to be independently predictive of nonconvulsive seizures during acute illness. Patients with LRDA had seizures at a rate of 63 %, which is similar to lateralized periodic discharges. Nonrhythmic polymorphic delta waves, on the other hand, were not predictive of seizures. There is insufficient evidence at this time to determine whether LRDA should be routinely treated with an AED. Unlike PLEDs, there is no suggestion that LRDA is ictal in nature; it may merely be a marker of severe focal brain injury.
3.5 SIRPIDs (See Also Chap. 5)
Stimulus-induced rhythmic, periodic, or ictal discharges (SIRPIDs) are found in approximately 20 % of ICU patients undergoing continuous EEG monitoring and are considered to fall somewhere along the ictal–interictal continuum. Clinical or subclinical/electrographic seizures are found in about half of these patients; status epilepticus is more frequent in focal or ictal-appearing SIRPIDs (Hirsch et al. 2004). Other studies have shown no increase in regional cerebral blood flow and as a result have advocated against aggressive treatment (Zeiler et al. 2011). Experience in our own center indicates that SIRPIDs are a transitional, unstable pattern that either will evolve into more definitively ictal pattern or more commonly dissipate in time, in either case losing the stimulus-induced character. Our recommendation is to start a conventional nonsedating AED; if this is already prescribed, further escalation of treatment is not recommended. Although a solid evidence for this approach is lacking, we then minimize stimulating the patient any more than medically necessary. After cardiac arrest, SIRPIDs are associated with poor outcome (Alvarez et al. 2013), but in other instances, outcome is yet to be defined.
3.6 Nonconvulsive Seizures
Nonconvulsive seizures (NCS) are commonly found in the ICU setting, in between 18 and 35 % of patients (Privitera et al. 1994; Claassen et al. 2004). Of these, up to 75 % of patients are in nonconvulsive status epilepticus (NCSE) (Jordan 1992) (see Chap. 4). The determination of NCS can be challenging due to the fact that many of the observed waveforms lie in the ictal–interictal continuum. The Young criteria (Young et al. 1996) (Table 3.1) provide a reasonable guideline in determining whether a pattern is consistent with NCS. This has been modified by other groups to emphasize the importance of frequency/locational evolution and de-emphasize amplitude changes (Chong and Hirsch 2005) (Fig. 3.5).
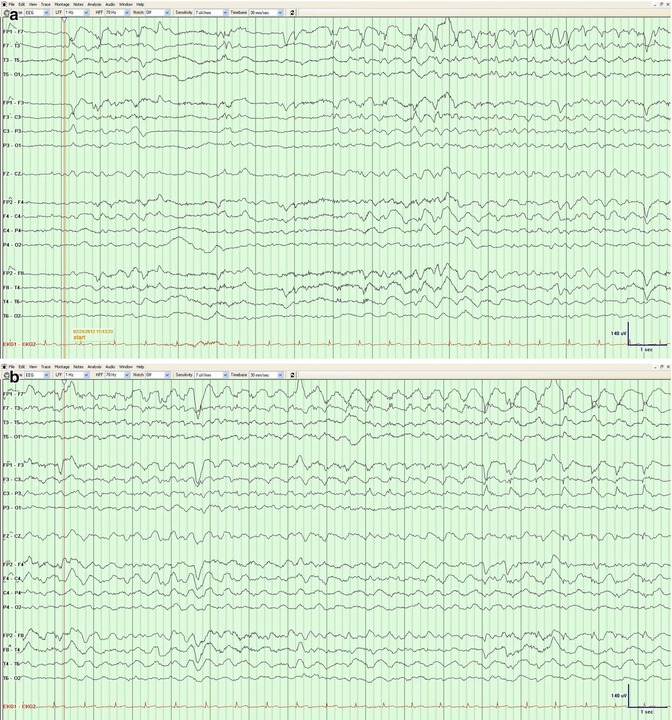
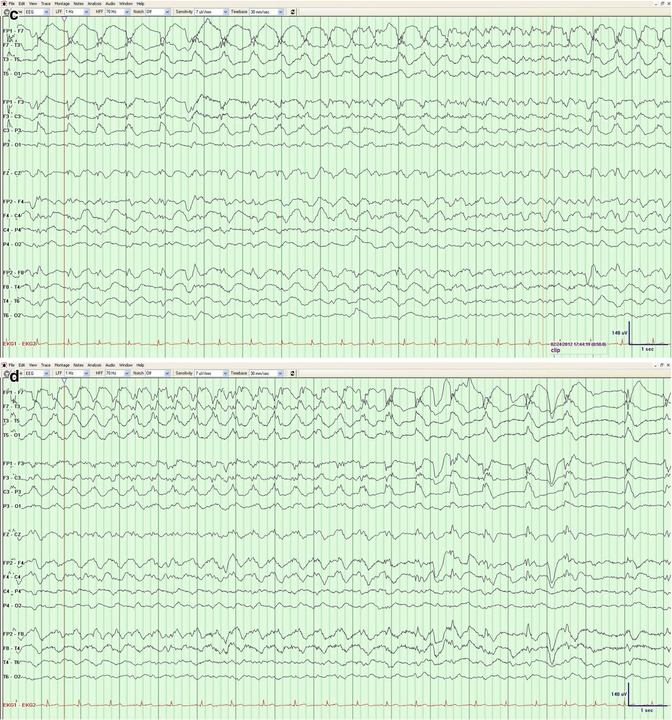
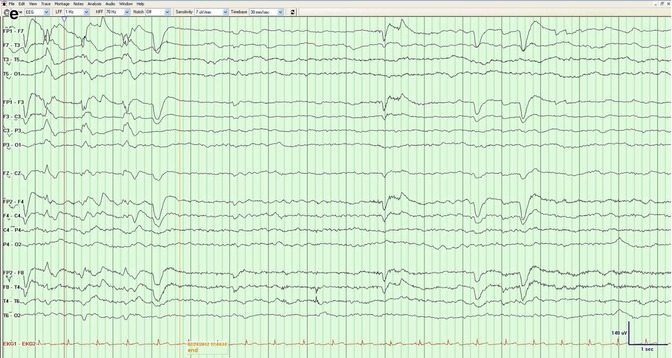
Table 3.1
Young criteria for an electrographic seizure or a nonconvulsive seizure; in order to qualify, at least 1 of the primary criteria and ≥1 of the secondary criteria are needed, with discharges lasting >10 s
Primary criteria | |
A. | Repetitive generalized or focal spikes, sharp waves, spike-and-wave, or sharp-and-slow wave complexes at >3/s |
B. | Repetitive generalized or focal spikes, sharp waves, spike-and-wave, or sharp-and-slow wave complexes at <3/s and secondary criterion d |
C. | Sequential rhythmic waves and secondary criteria a–c, with or without d |
Secondary criteria | |
(a) | Incrementing onset: increase in voltage and/or increase or slowing of frequency |
(b) | Decrementing offset: decrease in voltage or frequency |
(c) | Post-discharge slowing or voltage attenuation |
(d) | Significant improvement in clinical state or baseline EEG after antiepileptic drug |
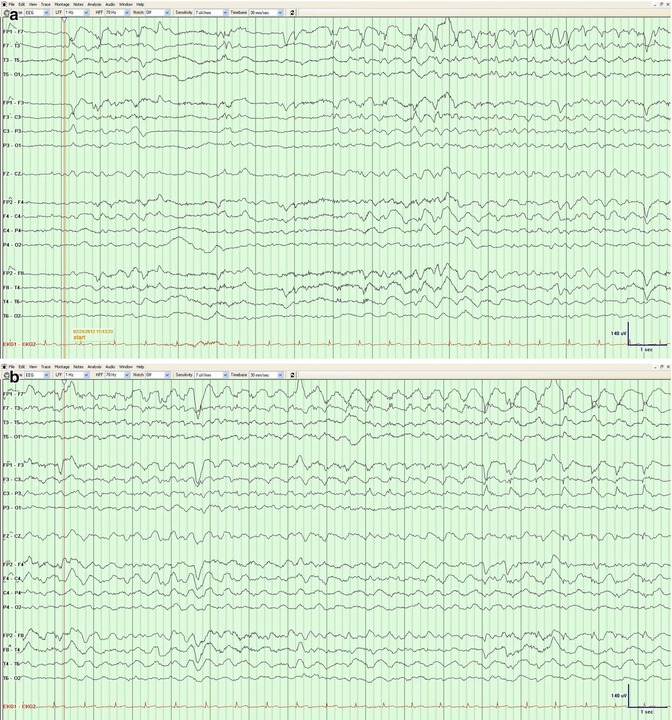
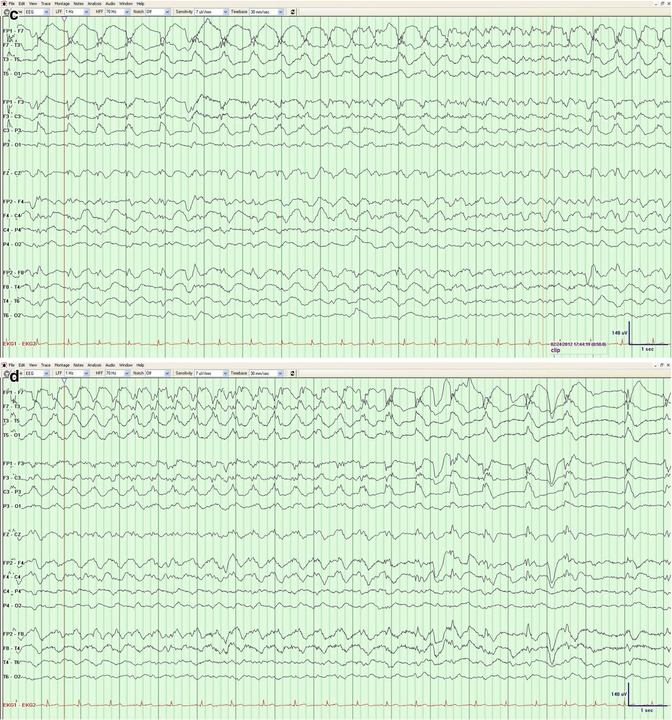
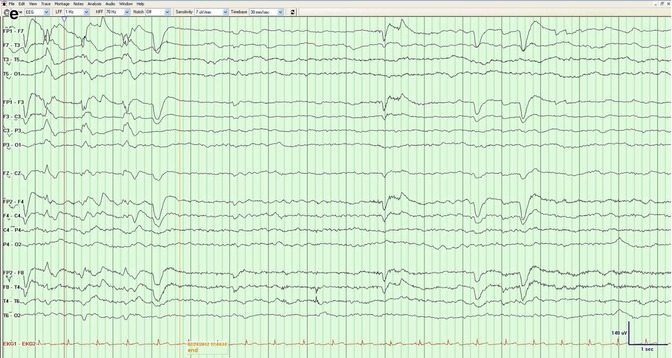
Fig. 3.5
(a–e) A 63-year-old man after meningioma resection. The evolution of morphology and frequency over the left anterior region is consistent with a nonconvulsive seizure
It has yet to be definitely determined whether NCS and NCSE independently cause neuronal injury or are mere epiphenomena of the underlying insult. NCSE occurring in the ICU is linked to a high mortality, and most of the morbidity from NCS is likely due to the underlying conditions, rather than the seizures themselves (Drislane 2006; Rossetti et al. 2006). In fact, there is no evidence of a clear neurocognitive deterioration after status epilepticus after eliminating progressive illness (Adachi et al. 2005), and aggressive treatment is likely to incur iatrogenic morbidities. However, other studies have shown deleterious effects associated with NCS. For instance, in patients with intracerebral hemorrhage, lesion expansion has been demonstrated in patients with NCS (Claassen et al. 2007). In subjects with traumatic brain injury, an increase in intracranial pressure and lactate–pyruvate ratio (Vespa et al. 2007) as well as hippocampal atrophy (Vespa et al. 2010) has been observed in those patients with NCS. However, the nature of the causality still remains unclear.
It is reasonable to treat all patients with NCS with at least a conventional AED. Escalation of treatment must be decided on a case-by-case basis. In general, there are few, if any, scenarios where de novo intubation and administration of an intravenous anesthetic is indicated solely for the purpose of the treatment of an EEG pattern.
3.7 Anoxic Brain Injury
Prior to the introduction of therapeutic hypothermia, status epilepticus was a predictor of poor outcome in anoxic brain injury (Rossetti et al. 2007). In particular, myoclonic status epilepticus was considered an agonal phenomenon (Wijdicks et al. 1994). In these patients, treatment of the epileptiform patterns on EEG was considered futile. The introduction of therapeutic hypothermia has resulted in an increased attention to the related EEG patterns. Seizures are common, occurring in 9–33 % of patients (Rundgren et al. 2010; Mani et al. 2012; Rittenberger et al. 2012; Crepeau et al. 2013); these frequently occur early on, often during the cooling or rewarming (Rittenberger et al. 2012; Crepeau et al. 2013). Postanoxic status epilepticus is still a strong independent predictor of poor outcome (Rittenberger et al. 2012; Crepeau et al. 2013; Legriel et al. 2013), despite treatment with AEDs. Nonetheless, a small number of patients with postanoxic status epilepticus, including myoclonic status, have been reported to survive beyond the vegetative state (Rossetti et al. 2009; Lucas et al. 2012).
The optimal AED management of patients with electrographic seizures undergoing therapeutic hypothermia remains unclear. On the one hand, the presence of seizures, particularly myoclonic status epilepticus, still portends extremely poor prognosis. On the other hand, it is unknown whether the presence of seizures may potentially cause additional morbidity in an already severely compromised brain. Particularly in a patient who presents seizure only upon rewarming and has other good prognostic signs (brainstem reflexes, reactivity on EEG, intact SSEP), suppression of seizures with an increase of an intravenous agent that may already be in place (e.g., midazolam) plus a moderate dose of a classical AED may be most reasonable, at least for some limited time (see also Chap. 5).
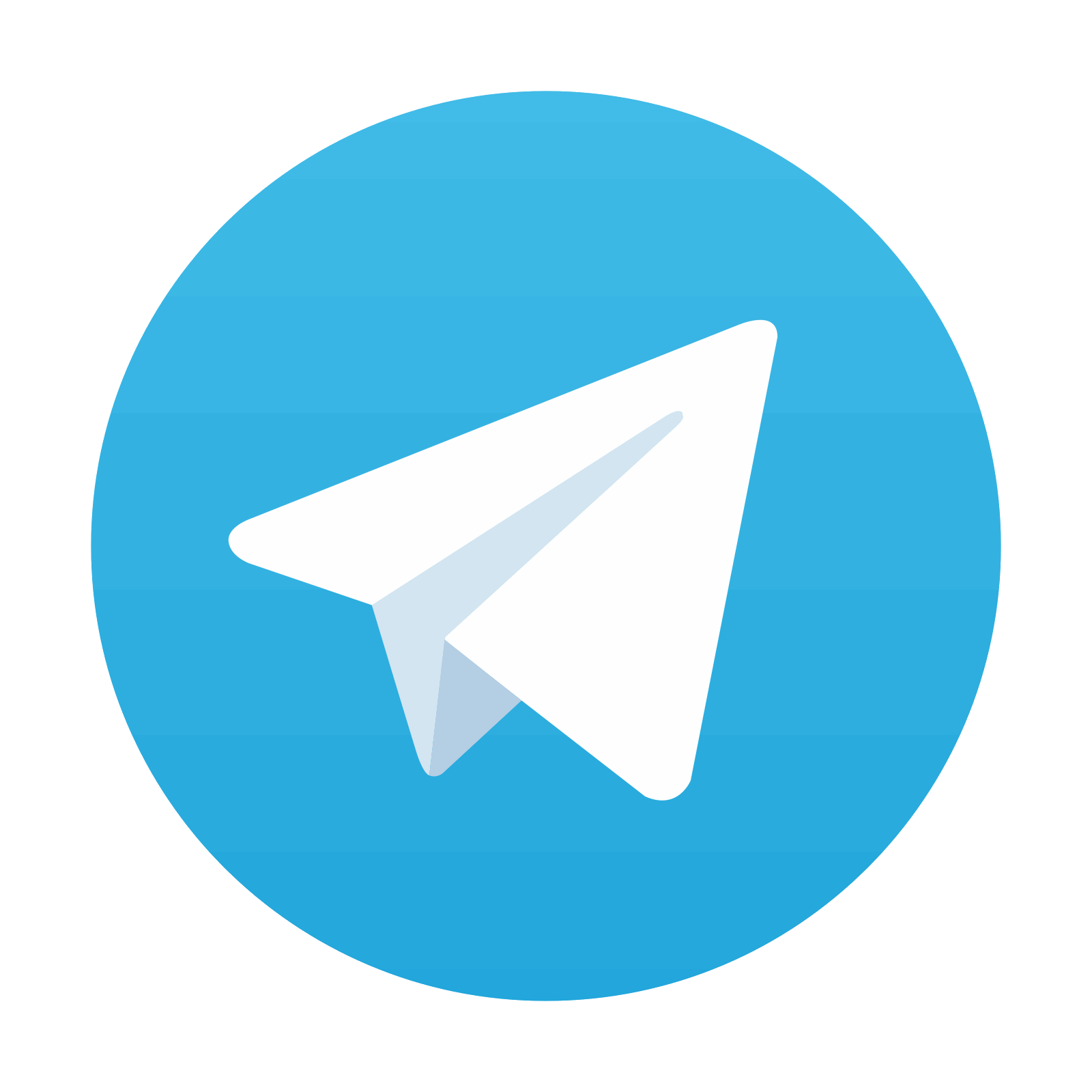
Stay updated, free articles. Join our Telegram channel

Full access? Get Clinical Tree
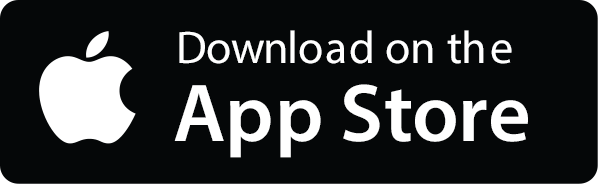
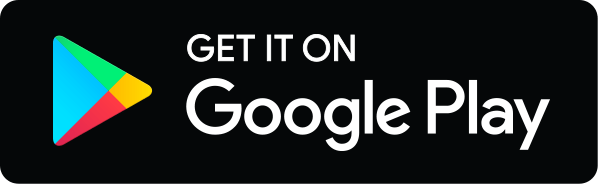